Zhao Yong1, Ibrahim N. Muhsen2, Mohamed Tarek Abdelaty1, 3, Muhammad U. Zafar1, Arwa A. Alanqary1, Nourah A. Alrubaiq1, Samah F. Alabbasi2, Randa Alnounou4, Youssef Omaia Elakwah1
1College of Engineering, Alfaisal University, Riyadh, Kingdom of Saudi Arabia
2College of Medicine, Alfaisal University, Riyadh, Kingdom of Saudi Arabia
3Currently at Department of Industrial and Systems Engineering, Korea Advanced Institute of Science and Technology, Daeieon, Republic of Korea
4Department of Pathology, King Faisal Specialist Hospital & Research Center, Kingdom of Saudi Arabia
Correspondence to: Zhao Yong, College of Engineering, Alfaisal University, Riyadh, Kingdom of Saudi Arabia.
Email: |  |
Copyright © 2015 Scientific & Academic Publishing. All Rights Reserved.
Abstract
Blood cells are composed of 99.9% erythrocytes, red blood cells. Erythrocytes transport oxygen to all cells of the body through narrow blood capillaries. The mechanical properties and deformability of erythrocytes are therefore vital in maintaining their ability to perform their functions. Many diseases or substances are known to affect erythrocytes in both shape and mechanical properties, such as: sickle cell anemia, or carbon monoxide, respectively. In this study, an experimental approach was used to study the effect of smoking on the mechanical properties of red blood cells. Experimental measurements were obtained using a nano-indentation tester, and preliminary results are reported. The commercial FEA package ANSYS was used to simulate the experiment and gain extra insight. The results of the study showed that smoking produces no significant changes in the mechanical properties of RBCs. Research involving more subjects with larger range of ages is needed to fully understand the effect of smoking on RBCs.
Keywords:
Red blood cells, Finite element method, Nano-indentation, Smoking
Cite this paper: Zhao Yong, Ibrahim N. Muhsen, Mohamed Tarek Abdelaty, Muhammad U. Zafar, Arwa A. Alanqary, Nourah A. Alrubaiq, Samah F. Alabbasi, Randa Alnounou, Youssef Omaia Elakwah, Experimental and FEM Study of Effect of Smoking on Red Blood Cells, International Journal of Biological Engineering, Vol. 5 No. 1, 2015, pp. 16-21. doi: 10.5923/j.ijbe.20150501.03.
1. Introduction
Red blood cells (erythrocytes) account for 99.9% of blood cells. The non-nucleated erythrocyte is unique among human cells in that the plasma membrane, its only structural component, accounts for all of its diverse antigenic, transport, and mechanical characteristics. One of the unique mechanical features of the normal red cell membrane is its high elasticity, which enables the cell to rapidly respond to applied fluid stresses in the circulation. This characteristic is primarily regulated by the geometry of the cell, cytoplasmic viscosity and membrane deformability (Mohandas & Gallagher, 2008). Some alterations to the mechanical properties are physiological in nature, e.g. cell age which increases the viscosity of the cytosol (Freund, 2013). On the other hand, pathologically many inherited diseases lead to decrease in RBCs elasticity thus hemolysis (Erythrocytes breakdown) (Mohandas & Gallagher, 2008). Smoking is one of the most common preventable habits worldwide, incorporated into people’s lifestyles leading to accelerated death. Tobacco smoke contains more than 4000 components exposing smokers to extensive amounts of toxins and oxidants (Khan, 2006) increasing the prevalence of acquiring various diseases such as respiratory, cardiovascular diseases, and cancer. Among the thousands of components, some are acknowledged to have an interaction with the red blood cells (erythrocytes) giving rise to hematotoxicity. For instance, carbon monoxide (CO) binds to hemoglobin; carboxyhemoglobin (COHb) is formed, accounting for its high toxicity. Although CO can be acquired from endogenous or exogenous sources, in smokers’ blood; the main CO concentration was acquired from cigarette smoking (Aberg et al., 2009). Leifert (2008) explained that smoking would ultimately lead to hypoxemia, destructing the lungs and increasing the erythropoietin production leading to a rise in erythropoiesis. Eventually, this would induce chronic polycythemia increasing the red blood cell volume and causing hyper-viscosity of the red blood cells suppressing erythropoietin.Other researches have looked to the effect of smoking from a molecular level. Padmavathi et al., 2010, for instance, have found that smokers showed increased hemolysis and membrane lipid peroxidation. Moreover, elevated nitrite/nitrate levels in plasma and red cell lysates were observed in a sample of chronic and heavy smokers. More recently, using the scanning electron microscopy (SEM), Pretorius et al. (2013) confirmed several changes in the red blood cell membrane that were not shown on the light microscopy. Bubble-like formations were seen on the membrane’s phospholipid layer that was said to be the cause of the decreased membrane fluidity and the structural changes in the cell membrane. This paper aims to take a biomechanics experimental approach to study the effect of smoking on red blood cells, using case-control methodology. More specifically, the effect of smoking on the mechanical properties of RBCs will be studied to investigate the effects structural changes detailed above may have on the elastic properties of RBCs. Different methods are commonly used to measure the mechanical properties of human cells, such as: AFM probing (McNally & Borgens, 2004), soft substrate stretching (Brown, 2000), optical tweezers, (Dao, Lim, & Suresh, 2003), magnetic-bead attachment (Wang, Butler, & Ingber, 1993), and nano-indentation. In this study, the nano-indenter currently available at Alfaisal University will be utilized to measure the mechanical compliance of red blood cells.The advent of nano-indentation, or depth-sensing indentation, has provided a method for determining time-dependent mechanical properties on a size scale compatible with tissue dimensions in controlled, physiological environments. (Gupta, 2008) Depth-sensing indentation involves the application of a controlled load or displacement to an indenter, inducing local deformation of the surface beneath the indenter tip. Both the load and the displacement are monitored simultaneously during loading and unloading of the indenter, and the resulting load-displacement curve shall be used to characterize the mechanical compliance of the surface. (Gupta, 2008; Zhang, 2008)A finite element model of RBCs is created based on Young’s modulus and hardness data obtained from the experiment for both control and smokers samples, in order to further investigate the compliance of the cells, and visualize the cell behaviors during in stressed and unstressed conditions, and during loading and unloading. The finite element simulation of the cell indentation process was done using the commercially available finite element package, ANSYS, to obtain the force-displacement curves and compare them with experimentally obtained ones, as well as to showcase the expected stress distribution in the cell during the indentation process.
2. Methodology
The method used consists of: 1. Experimental trial: A case-control approach was used to determine the difference in the elastic properties between erythrocytes from smokers and non-smokers.2. Simulation Process: The modulus of elasticity obtained from the experiment for smokers and non-smokers was used to create a finite element model of the RBC and simulate the indentation process.
2.1. Sampling
Preliminary experimentation involved consented 14 healthy adult male subjects sampled by convenient sampling from Alfaisal University’s student, staff and faculty population. Case-control approach was used, with a case group of 7 smokers, and a control group of 7 nonsmokers. All subjects were between the age of 18 and 25. Smokers were regularly consuming a minimum of 1 pack per day for at least 5 years. The enrolled subjects all classified themselves as healthy individuals with no chronic diseases or any recent acquired infections and no history of recent drug use. This was done to make sure the effect of diseases or drugs on the mechanical properties of erythrocytes is at best avoided. The ethical clearance for this study was attained from the Institutional Review Board (IRB), Office of Research and Graduate Studies, Alfaisal University, and from the Research Advisory Committee (RAC), KFSH&RC.
2.2. Sample Preparation
Samples were prepared primarily according to (Zuk et al., 2011):1. 5 mL (1 teaspoon) of blood was drawn from volunteers.2. Sample was centrifuged and erythrocytes were extracted.3. A small sample was used to run a hemoglobin test for the erythrocytes sample. 4. Erythrocytes were then diluted using the following mixture: phosphate buffer solution, 1% bovine serum albumin, and 0.5% glucose solution in proportion of 20:4:1 respectively by volume. 5. After that, one drop of this suspension was placed on a glass substrate treated with poly-L-lysine, and incubated for an hour at 37.0 ˚C. 6. The membrane’s elasticity was then measured using a nano-indenter.
2.3. Nano-indentation
In this study, the nano=indentation system available at Alfaisal University was used to measure the mechanical compliance of red blood cells. Depth-sensing indentation was performed under controlled load and displacement conditions using a Berkovich indenter, Figure 1, to induce local deformation of the surface beneath the indenter tip. The indentation process is performed in three steps: loading, dwell time, and unloading. Displacement and force are monitored simultaneously during the three steps of the indentation, and the resulting load-displacement curve was used to characterize the mechanical compliance of the surface using the following formula (Gupta, 2008; Zhang, 2008) | (1) |
where F is the force applied to the RBC specimen, E is the Young's modulus of the specimen, S = 1/E is the mechanical compliance,
is the Poisson's ratio (often assumed to be 0.5),
is the equivalent half opening angle of the indenting tip, and
is the indentation depth. (Zhang, 2008) From the above equation,
and
are provided by the Nano indentation measurements,
is a known property of the indenter’s head used,
is assumed, and S can therefore be computed.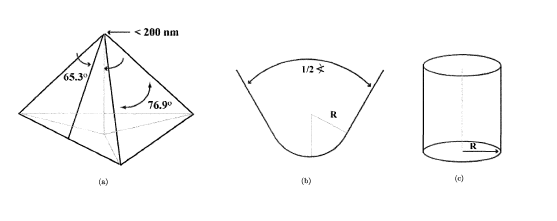 | Figure 1. Schematic Berkovich tips (Gupta, 2008) |
2.4. Finite Element Simulation
In this study, 3D models of the RBC and the Berkovich indenter were created using SolidWorks software in order to create the FE model in ANSYS. The RBCs are modeled as a linear elastic material with symmetric biconcave shape estimated by the function: (Dao et al, 2003) | (2) |
where C0 = 0.207161, C1 = 2.002558 and C2 = −1.122762. The axial cell diameter 2R0 is taken as 8.26 μm. (Yang et al, 2011). A 3D model of the RBC generated by SolidWorks is shown in Figure 2. 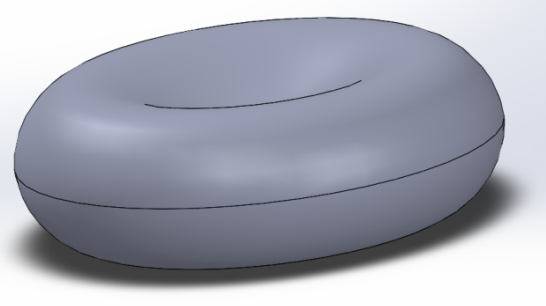 | Figure 2. 3D model of biconcave RBC |
The Berkovich tip is modeled as a rigid body with a half angle of 65.3 degree, shown in Figure 3. 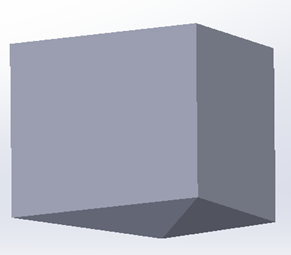 | Figure 3. 3D model of Berkovich tip |
The Ansys FEM package was employed in the numerical simulation. A fine mesh is used for the RBC model in order to obtain better result. The cell is uniformly meshed with tetrahedral elements and a 0.15µm element size. The bottom and top surfaces of the cell are attached with frictionless contact to the rigid fixed slide and the indenter tip, respectively as shown in Figure 4. The rigid Berkovich indenter is meshed with an element size of 1µm. Pressure is applied to the top surface of the indenter in the negative y-direction. 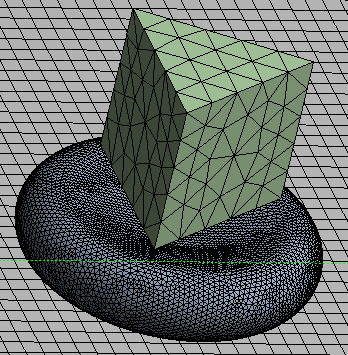 | Figure 4. RBC and indenter mesh |
As shown in Figure 5, a variable pressure is used to simulate both the loading and unloading of the indenter over a time interval of 1e-7 s. 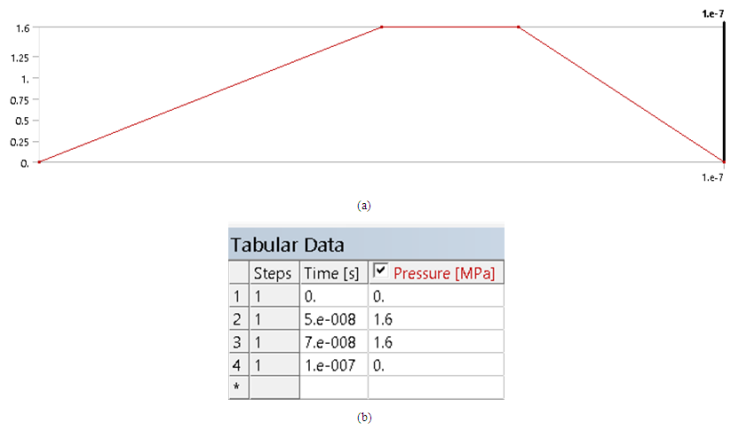 | Figure 5. Pressure vs. time |
The stress and displacement of the cell are obtained from the FE model and the force is then calculated as: | (3) |
where σ is the stress obtained from the FE model calculations at each time step, and At is the corresponding Berkovich tip area that is in contact with the cell at time step t, the area is given by the formula  | (4) |
where K = 24 is constant for the Berkovich tip used in this model, ht is the indentation depth at each time step t.
3. Results and Discussion
Microscopic view of an RBC sample is shown in Figure 6 and the nano-indentation setup in Figure 7.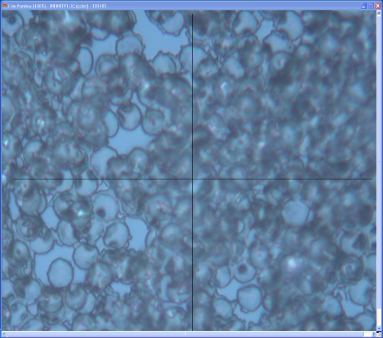 | Figure 6. RBC sample under the microscope of the nano-indenter machine |
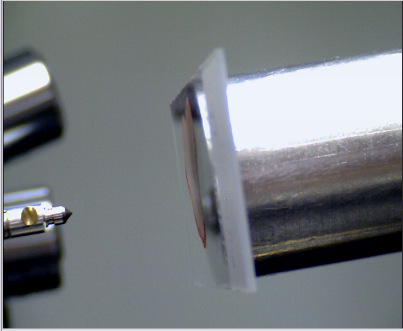 | Figure 7. A sample on a slide ready to undergo nano-indentation |
Experimental measurements of the nano-indentation are shown in the Table 1 for the hardness and reduced modulus:Table 1. Reduced moduli and hardness of the RBCs of smokers and non-smokers obtained from nan-indentation experiment 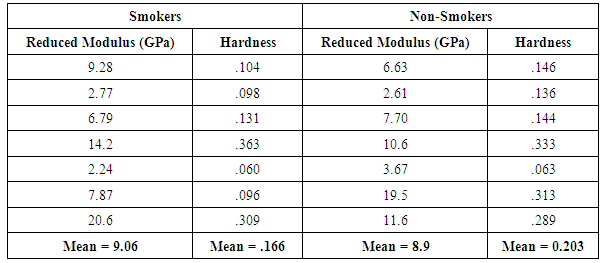 |
| |
|
A Mann-whitney U hypothesis test was undertaken to compare the means of the reduced modulus and hardness, with a null hypothesis H0:
and alternate hypothesis Ha:
. P-values obtained were 0.949 and 0.277, for the reduced modulus and hardness respectively, against rejecting the null hypothesis, showing that there is no significant difference between the two samples. FEA simulation was then performed to demonstrate a simulated nano-indentation process based on measurements from experiment, and compare it with experimental results for case and control samples as shown in Figure 9. 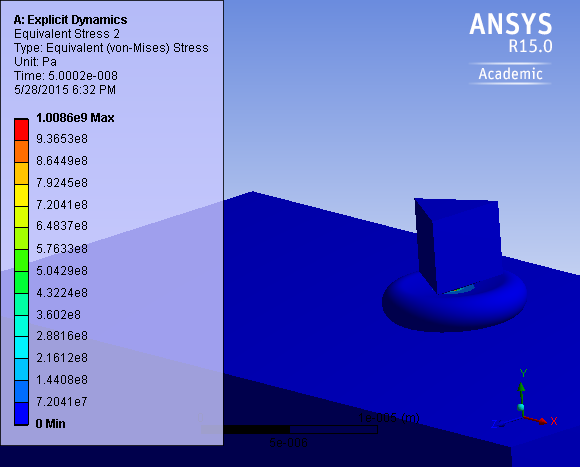 | Figure 8. Induced stress in the cell due to nano-indentation from simulation |
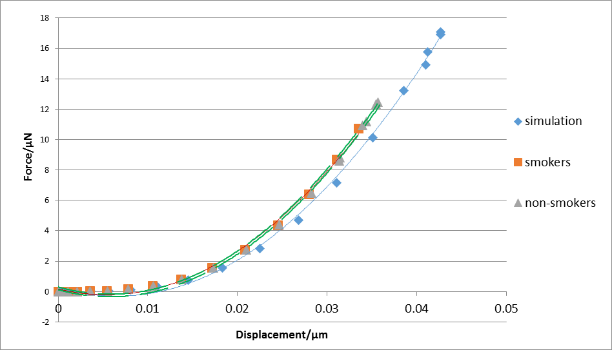 | Figure 9. The force-displacement graph for typical simulation and experimental results (smokers and non-smokers) |
Figure 9 compares the force-displacement curves obtained from experiments and FEA. It is shown that curves from experiments and the FEA generally agree with each other, but the FEA tends to underestimate the forces for the same displacements for all cases. It has been found (Chen et al., 2012) that the Hertz contact model underestimates the force for known values of elastic moduli of cells. Therefore, the Hertz model used to calculate the Young’s modulus from experimental data can generate underestimated such values for cells. Since the contact model in Eq. (1) is similar to that of the Hertz model, it is very likely that the reduced moduli for the RBCs are also being underestimated. Consequently, when these measured moduli are used in the FEM, they produce underestimated forces for the same displacements, compared with the corresponding raw experimental measurements.
4. Conclusions
In this study, preliminary experimental results are showcased to investigate the effect of smoking on the mechanical properties of RBCs. Blood samples from a case group of smokers and a control group of non-smokers were collected, pre-processed to extract RBCs, and mechanically characterized by nano-indentation. Results for Young’s Modulus and hardness were compared for both groups by hypothesis testing, and the null hypothesis of zero difference in means could not be rejected. However, the results of this research is limited by the number & age of volunteers, thus further sampling and experimentation is still thought necessary to reduce the probability of a Type II error. Finally, the elastic moduli obtained from experiments based on a contact model were used in FEM simulations. In conclusion, results thus far seem to support that smoking produces no significant changes in the mechanical properties of RBCs.
ACKNOWLEDGEMENTS
This project is supported by Alfaisal University IRG grant No. 226250101141. We would like to acknowledge also King Faisal Specialist Hospital and Research centre (KFSH & RC) for supporting the project logistically.
References
[1] | Åberg, A. M., Sojka, B. N., Winsö, O., Abrahamsson, P., Johansson, G., & Larsson, J. E. (2009). Carbon monoxide concentration in donated blood: Relation to cigarette smoking and other sources. Transfusion, 49(2), 347–353. http://doi.org/10.1111/j.1537-2995.2008.01951. |
[2] | Brown, T. D. (2000). Techniques for mechanical stimulation of cells in vitro: a review. Journal of biomechanics, 33(1), 3–14. Retrieved from http://www.ncbi.nlm.nih.gov/pubmed/10609513. |
[3] | Chen J. & Lu G.X. (2012). Finite element modelling of nanoindentation based methods for mechanical properties of cells. Journal of Biomechanics ,45, 2810-2816. |
[4] | Dao, M., Lim, C. T., & Suresh, S. (2003). Mechanics of the human red blood cell deformed by optical tweezers. Journal of the Mechanics and Physics of Solids, 51(11-12), 2259–2280. doi:10.1016/j.jmps.2003.09.019. |
[5] | Freund, J. B. (2013). The flow of red blood cells through a narrow spleen-like slit. Physics of Fluids, 25(11), 1–19. http://doi.org/10.1063/1.4819341. |
[6] | Gupta, S. (2008). Analytical and Numerical Nanoindentation Studies of Compliant Biomaterials and Soft Tissues (pp. 1–52). |
[7] | Khan, M. G. (2006). Effects of smoking and heart disease. In Encyclopedia of Heart Diseases (1st ed., p. 335–339). |
[8] | Leifert, J. a. (2008). Anaemia and cigarette smoking. International Journal of Laboratory Hematology, 30(3), 177–184. http://doi.org/10.1111/j.1751-553X.2008.01067.x. |
[9] | McNally, H. A., & Borgens, R. Ben. (2004). Three-dimensional imaging of living and dying neurons with atomic force microscopy. Journal of neurocytology, 33(2), 251–8. Retrieved from http://www.ncbi.nlm.nih.gov/pubmed/15322383. |
[10] | Mohandas, N., & Gallagher, P. G. (2008). Red cell membrane: past, present, and future. Blood, 112(10), 3939–48. doi:10.1182/blood-2008-07-161166. |
[11] | Padmavathi, P., Reddy, V., Kavitha, G., Paramahamsa, M., & Varadacharyulu, N. (2010). Chronic cigarette smoking alters erythrocyte membrane lipid composition and properties in male human volunteers. Nitiric Oxide, 23(3), 181-186. |
[12] | Pretorius, E., du Plooy, J. N., Soma, P., Keyser, I., & Buys, A. V. (2013). Smoking and fluidity of erythrocyte membranes: a high resolution scanning electron and atomic force microscopy investigation. Nitric Oxide: Biology and Chemistry / Official Journal of the Nitric Oxide Society, 35, 42–6. http://doi.org/10.1016/j.niox.2013.08.003. |
[13] | Wang, N., Butler, J. P., & Ingber, D. E. (1993). Mechanotransduction across the cell surface and through the cytoskeleton. Science (New York, N.Y.), 260(5111), 1124–7. Retrieved from http://www.ncbi.nlm.nih.gov/pubmed/7684161. |
[14] | Yang, Q., Li, W., Liu, Z., & Su, L. (2011). Nanoindentation Experiment and Modeling for Biomechanical Behavior of Red Blood Cell, 227–231. |
[15] | Zhang, W. L. (2008). Design, Modeling, Fabrication, and Characterization of a MEMS Device for Measuring the Mechanical Compliance of a Biological Cell. |
[16] | Zuk, A., Targosz-Korecka, M., & Szymonski, M. (2011). Effect of selected drugs used in asthma treatment on morphology and elastic properties of red blood cells. International journal of nanomedicine, 6, 249–57. doi:10.2147/IJN.S15802. |