Bernd Johannes1, Sergey Bronnikov2, Yuri Bubeev3, Michael Arz1, Sarah Piechowski1, Jörn Rittweger1
1Institute of Aerospace Medicine, German Aerospace Center (DLR), Linder Hoehe, Cologne, Germany
2S.P Korolev Rocket and Space Corporation “Energia”, Moscow, Russia
3Institute for Biomedical Problems (IBMP) of the Russian Academy of Sciences, Moscow, Russia
Correspondence to: Bernd Johannes, Institute of Aerospace Medicine, German Aerospace Center (DLR), Linder Hoehe, Cologne, Germany.
Email: |  |
Copyright © 2019 The Author(s). Published by Scientific & Academic Publishing.
This work is licensed under the Creative Commons Attribution International License (CC BY).
http://creativecommons.org/licenses/by/4.0/

Abstract
A newly developed tool to facilitate learning processes in complex perceptual-motor tasks was tested for its efficiency characteristics. The tool is based on a demanding manual control task, in which a target object has to be manipulated with six degrees of freedom. During the self-sufficient learning process the task difficulty is increased from very easy to the complex level of a manual spacecraft docking on a space station. The type and the difficulty of the next task were defined by a regulation system based on individual performance. The tool was successfully applied to 22 participants in a laboratory setting. No performance differences were found between genders. Age was correlated with training duration but slower learners also succeeded eventually. The tool provides improvements in the learning process for operational tasks like controlling a robot arm or to perform a docking maneuver. Beside the foreseen practical application in space this is a time effective and economic way to educate participants in ground based research requiring such complex skills.
Keywords:
Perceptual-motor performance, Intelligent self-sufficient tutor, Simulation-based skill acquisition
Cite this paper: Bernd Johannes, Sergey Bronnikov, Yuri Bubeev, Michael Arz, Sarah Piechowski, Jörn Rittweger, Individual Learning Curves in Manual Control of Six Degrees of Freedom, International Journal of Applied Psychology, Vol. 9 No. 2, 2019, pp. 47-51. doi: 10.5923/j.ijap.20190902.01.
1. Introduction
This paper presents the results of a first validation study for the new training tool (termed “6df”) to learn, refresh, and practice the manual control of objects with six degrees of freedom (DoF). The fundamental features and aims of the tool have previously been published [1]. Here, we report new results from a study in 22 participants. The ability to control the docking maneuver in space manually has become an important feature for manned space flight. The present tool has been developed to investigate and support the acquisition and maintenance of manual docking skills of spacecraft during long duration missions in space [2]. It focuses on the learning of cognitive, perceptual and motor skills in the manual control of objects with six DoF. It has been shown that the manual control in the “docking” maneuver is extremely challenging [3]. The six DoF in this task consist of moving an object in three dimensions in space (3 DoF of ”translation”) and to rotate (3 DoF of ”orientation”) it around these dimensions. Notably, manipulation of six DOF, as in space, is rarely necessary on Earth. A major challenge of the task is to generate an internal perceptual three-dimensional image based on a two-dimensional screen. Both, the trajectory of the flown object and its orientation in space have to be monitored continuously, while the target object remaining within sight of the camera monitor. This often requires sideward flying, which is unusual on Earth and rather demanding. When intending to change the flight path the operator has to take into account the actual orientation of the flown object. This is extremely difficult because we are used to control along a horizontal plane (right-left) and we are unfamiliar with the free movement along the vertical (up-down) axis or simultaneous movements around rotatory axes. In addition, dizziness and motion sickness may be evoked by the perception of these movements.The usual way to control six DoF is by having two hand controllers: the left hand for translation and the right hand for orientation. The control of objects under space conditions is so difficult because left and right hands have to be controlled independently, whereas the way of controlling differs enormously.Based on the principles for the development of training programs for high-performance skills [4, 5], we used a concept which favors part-task instead of whole-task training for complex skills especially for inexperienced trainees.
2. Methods
2.1. Subjects
For the first validation of the newly developed 6df tool, participants were recruited amongst the DLR workforce in Cologne, Germany. No re-imbursement was provided; the only incentive was the space affinity of the task. The 16 male and 6 female participants were 17 to 60 years old and had a body mass index 24.0 ± 3.0. They worked in three native languages (German 20; Russian 1; English 1). Professionally they were mixed from mean and high academic degrees. The experiment “6df” was ethically approved (Nr.: 2014239) by the local committee in NRW, Germany.
2.2. The Task
The task simulates the manual control of a virtual spherical object in space with six DoF. A chain of successive semi-transparent ellipsoid rings displays the required path towards the target object. The three dimensional path of ellipsoids has to be traversed while looking from inside the moving object to outside via a camera monitor with a visual adjustment grid (visor). The arrangement of the ellipsoid rings requires also rotating around the moved object’s three body axes. The visualized pathway provides guidance to the controlled object from its current position to the target and helps the trainee to not deviate from the approach corridor. Upon any collision with the corridor the task was aborted and rated as unsuccessful. Up to a certain level of training a secondary display presented a view at the task situation from outside. For the final stages this secondary display and the ellipsoid corridor were omitted. In the present application the functionalities of controllers and flight dynamics reflect the characteristics of the regular Soyuz spacecraft and the Russian docking training system TORU (Ru: telemetric operations with hand control).Performance results were presented for purposes of feedback immediately after finishing a task as a green-yellow-red coded table. Additional feedback was sometimes also given by the instructor replaying the tracking performance and commenting the work.
2.3. Training Program
The training started in a familiarization phase with the different functionalities of the two hand controls for translation and orientation. Basic elements of the control were practiced separately: moving along the object axes, oblique to the moved object axes, curves, final approach, and contact (capture, docking). The degrees of freedom that had to be controlled were increased systematically. Whether a trainee progressed to the next level was based on the performance of the trainee. When the performance was not sufficient the trainee either received the same task or a different one of same difficulty level. When serious errors occurred the task difficulty was decreased, the subject stepped back in the learning course.The education process was supported by an illustrated text script and video lessons. They provided a general overview and explained the six DoF and their relation to the hand controls, and basic aspects of maneuvering an object in space (e.g. how to fly a curve). For the single tasks html-pages included short text instructions, pictures showing the initial position, relevant action moments during the task, and the final position. The texts and audio files were prepared in different languages (English, German and Russian). The instruction text consisted of a description of the task, how to control it and a general lesson learned. One session consisted of eight tasks and/or was time limited to 45 minutes.The performance assessment is based on the well-established method of the Russian docking training system [6]. The methodology is in detail described in [7]. In addition a control input analysis was developed similar to methods described by Mueller et al. [8] and Dude et al. [9].The hand controllers were produced by Koralewski Industrie Elektronik oHG (Hambühren, Germany). The software was developed by SpaceBit GmbH (Eberswalde, Germany).
2.4. Training Success Evaluation
The learning phase ended with a phase of at least three sessions at the docking level for skill stabilization. This was needed because the subjects participated afterwards in a psychophysiological experiment where a solid docking skill was necessary. In nearly all cases a P300 occurred in response to a secondary stimulus as sign of free cognitive capacity during the standard docking flight [10]. After the initial phase of the study it was invited to participate in an original series of training tasks, exactly as supplied to Russian cosmonauts onboard the ISS from 2008 to 2011. The Russian training system TORU, provided by the Rocket and Space Corporation “Energia”, is used in continuously developed versions for the regular training of cosmonauts since start of the ISS up today. The version from 2008 to 2011 was used for joint Russian-German psychophysiological experiments [11] and could be applied in this study.
2.5. Statistical Data Analysis
For the statistical analysis the SPSS IBMP package (V21) was used. Linear mixed effect (LME) models were developed to test the statistical significances of the independent variables as fixed effects. The subjects were included as random effects. The visual inspection of the q-q-plot was used model scrutiny. However, because the testing of the normal distribution of residuals by Kolmogorov-Smirnov did not provide desired results (also after several attempts to transform the primary data) for all relevant comparisons additionally non-parametric tests were applied. The Kolmogorov-Smirnov-Z (KS-Z) test is more conservative and was preferred due to the small sample size to be compared in gender groups, age groups, and learning speed groups. A p value of 0.05 was accepted as significance level.
3. Results
3.1. Learning Process Characteristics
The subjects performed 616 sessions during 441 laboratory days with a total of 4304 training tasks (more details see Table 1).Table 1. Frequencies of tasks and sessions 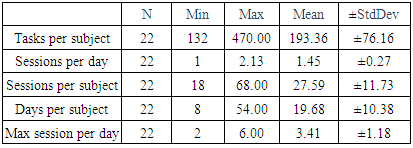 |
| |
|
Notably, subjects required between 109 and 422 single tasks to reach the proficiency required for a standard docking maneuver (level 60) and between 132 and 470 tasks to establish a certain level of reliability. The differing learning curves are presented in figure 1, with each symbol representing a single participant’s level progress over the course of training sessions. Notably, the difficulty levels are not interval scaled, but represent an ordinal scale. Especially above the first docking level (level 20) the level differences are clearly larger. This numbering allowed implementing new task types during the development of the training procedure. Apparently, two main groups of subjects can be discerned: a group of faster learners that reached standard docking level in about 15-20 sessions, and a group of slower learners that needed more than 25 sessions. Additionally there is one extreme outlier who did not reach standard docking level up until about 60 sessions. The main differentiation between the participants happens already in the very beginning of the training, lower level 15. Level 15 represents a kind of pattern drill which is needed prior the very first contact flight (occurring on level 20). This results in a certain difficulty plateau for each subject on level 15. Situational awareness as well as the skills to use the hand controller had to be stabilized as far as any input error provides angle errors near the docking point which are hardly to correct. In larger distance these angle errors are still not that critical. Any other plateau indicates individual problems and task repetitions at that difficulty level. The slower group needed more time to attain the basic skills compared with the faster participants. Beyond the critical level 15, learning curves in both groups are rather similar in shape. The outlier participant’s learning curve however is flatter from the start, requiring consistently more sessions to level up than the others.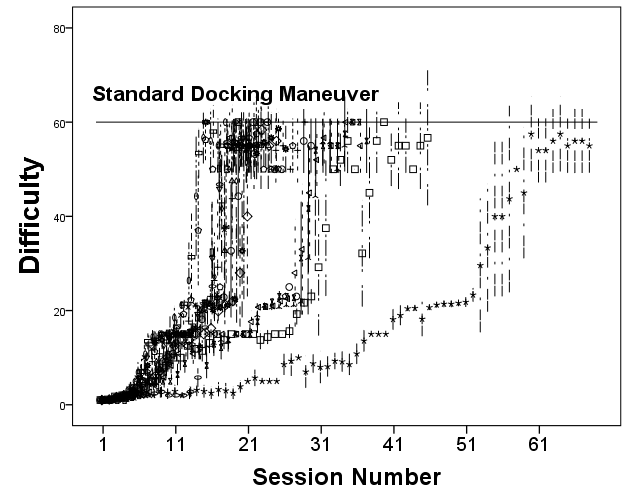 | Figure 1. Individual learning curves up to the difficulty level of a standard docking maneuver |
Figure 2 demonstrates how the participants solved more than one session in the very first training days. The number of sessions per day changed significantly (F(53) = 18.79, p < 0.001) over time.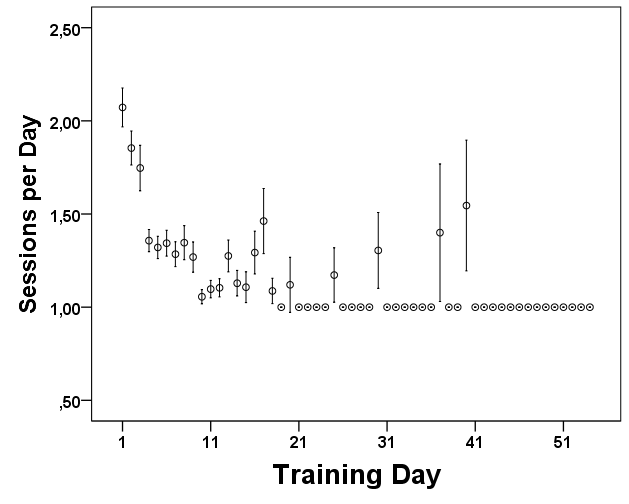 | Figure 2. Mean amount of sessions during a training day |
3.2. Group Differences
Generally, males prevail in the aviation and space personnel, and more males than females were willing to participate in this study. However, performance in 6df, and thus in mission relevant challenge, there was no gender difference found (figure 3; F(num:1, denum: 82,267) = 0.050, p = 0.824; MW-U = 39.0, p = 0.541). There were also no significant differences in the number of tasks (KS-Z = 0.783, p = 0.571), sessions (KS-Z = 0.740, p = 0.644), or training days (KS-Z = 0.870, p = 0.435), that male vs. female participants required until reaching the docking level. The difference in performance was not significant in the very first docking task (KS-Z = 0.653 p = 0.788) and neither over all tasks averaged per subject on the docking level (figure 3; (KS-Z = 0.653, p = 0.788)).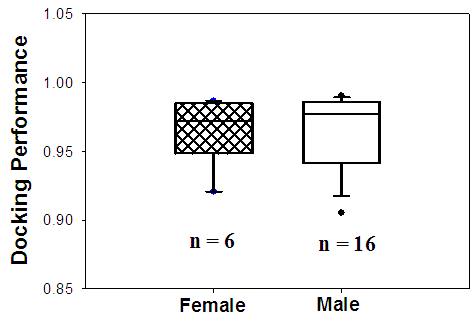 | Figure 3. 6df performance of female and male participants at final docking level; no significant differences were found |
There was a significant correlation found between the age of participants and the duration of the training curse (r = 0.631, p = 0.002). But in performance at docking level no difference was found between age half groups. Considering the performance of the slower learners versus the faster ones, three subjects (clearly separated in Figure 1) needed more than 200 tasks in more than 25 sessions to reach the docking level and were grouped together as “slower learners”. Their performance in the tasks on docking level was not significantly different from the faster learning participants (figure 4; KS-Z= 1.101, p = 0.177).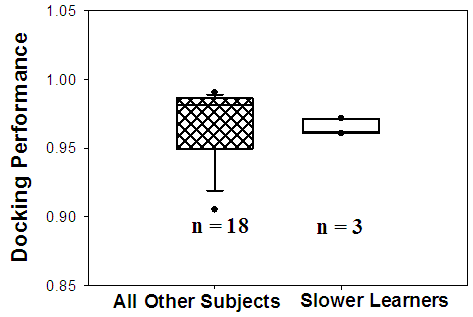 | Figure 4. 6df performance of slower learners in comparison to all other participants. At final docking level no differences were found |
Fourteen of the 22 participants accepted the invitation to an voluntary additional session, verifying their new skills in one single session using the regular Russian docking training system TORU. The performance of these participants was compared to data that had previously been obtained in space (ISS 2008-2011, Figure 5).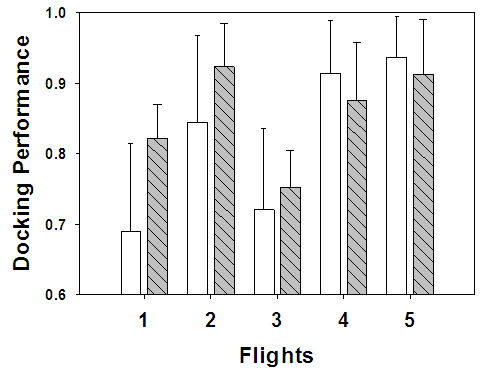 | Figure 5. Performance in regular docking training tasks of cosmonauts in space (gray, 2008-2011) and the study participants (blank). 6df-participants reached sufficient performance within five tasks |
Generally, a significant difference was found, cosmonauts performed better (F(num:1, denum: 19,731) = 7.631, p = 0.012; KS-Z p = 1.749, p = 0.004). The first and second tasks were significantly more difficult for the participants (LME-F (num:1, denum: 91,905; pairwise adjusted comparisons: flight 1: p < 0.001; flight 2: p= 0.001). However, for the last three tasks this difference was no longer significant (flight 3: p = 0.269; flight 4: p= 0.650; flight 5: p=0.305; KS-Z flight 3: p=0.184; flight 4: p=0.624; flight 5: p=0.418). The third flight was obviously more difficult for both cohorts.
4. Discussion
A new educational and training tool (“6df”) was verified in a laboratory study. To permit practical application in space work and research it had to be verified for its self-sufficient applicability and the final efficiency. The education and training of operators who need to control objects with six degrees of freedom is usually a task for instructors and large and expensive simulators. The participants started the program without any prior experience with controlling six degrees of freedom. It can be assumed that this applies for other populations with similar educational and professional background. The study provided first evidence that the 6df-tool allowed the participants to acquire the necessary complex skills to control an object with six degrees of freedom, similar to a hand controlled docking maneuver in space. Only a minimal interaction with the investigator was provided.Results show that participants were eventually able to solve a manual docking task. It could be demonstrated that a subgroup of them was even able within only one training session to solve the training tasks of the regular Russian docking training system TORU. We did not find any significant differences between male and female participants, neither in learning speed nor in the performance on the final difficulty level of the standard docking maneuver. However, the gender subgroup sizes are rather small. We would like to underline also another subgroup analysis. Due to the unusual complexity one might assume that not all people can learn the control of six degrees of freedom. As an indicator the amount of needed training sessions and tasks might be used as a predictor. At least in this study the slower learners were found to have comparable performance in the end of the study. Generally, all subjects were experiencing difficulties at individual stages. The adaptive training program allowed them specifically to repeat and train these difficult moments. It can clearly be seen, that the individual differences in learning progress are visible already in the initial phase of training, still learning to understand the spacial situation and to use the very different hand controls. Future support might focus on that period. Finally, the inexpensive version of the simulator also provided the unlimited possibility to learn as long as the participants needed. We speculate that more difficult tasks (e.g. docking or catching rotating objects) might increase the learning and training effort enormously. However, for a standard docking maneuver, possibly necessary after a long duration space mission, the 6df-tool or any in future improved analog may be a helpful tool for astronauts to establish or re-establish the docking skill for a successful docking at the home station.Other applications in space would also be possible. In the recent years NASA intensified the work to develop new tools to make EVA (extra vehicular activity) more flexible and safer [12]. For the EMU (extravehicular moving unit), providing much larger range of activity in open space, a specific safety system, SAFER (simplified aid for EVA rescue) is under development [13]. For this system a quite different hand control system is foreseen. But the general required skill, to control six degrees of freedom, remains the same. It is a specific situation for space applications that the astronauts can train this skill on simulators only. These kind of “artificial” skills decrease relatively dramatically and require a permanent training or effective refreshment tools.For terrestrial use one may focus on hand controlled robot arms which have really diverse applications in medicine, industry or even disaster rescue technologies. In any case our tool may provide help to researchers in this field because it can train the certain skill in the required number of participants effectively and time efficiently. Given enough time, the learning tool leads to a sufficient skill in controlling six degrees of freedom in cohorts differing in age, gender or learning speed.
ACKNOWLEDGEMENTS
The very first prototype of the training tool was developed in cooperation with the Institute of Aerospace Medicine of the German Airforce. The experiment “6df” was evaluated and selected by ESA for the MARS500 project in the Institute of Biomedical Problems (IBMP), Russian Academy of Science, Moscow, Russia. Further development of the 6df hard- and software was granted by the DLR space administration to Koralewski Industrie Elektronik and by DLR’s head quarter space research department to the first author. Greatest thanks go in memory to Vyatcheslav P. Salnitski (deceased 2016), who pioneered the complex IBMP-research about the docking maneuver.
References
[1] | B. Johannes, S.V. Bronnikov, Y.A. Bubeev, A.V. Dudukin, H.J. Hoermann, T. Frett, J. Rittweger, and A.K.W. Gaillard. A tool to facilitate learning in a complex manual control task. International Journal of Applied Psychology, 2017, 7(4): 79-85. |
[2] | J.J. Asher and J.A. Sciarrino, “Realistic work sample tests: a review,” Personnel Psychology, 27, 1974, pp.519-533. |
[3] | A.S. Eliseev and B.V. Rauschenbach, “Cosmonaut’s work during control of spacecrafts,” In: V.N. Tschernigovski (Ed.) Problemy kosmicheskoi biologii. vol. 34, Moscow, Russia: Nauka, 1977, pp. 39-49. |
[4] | W. Schneider, “Training high-performance skills: fallacies and guidelines,” Human Factors, vol. 27(3), 1985, pp. 285-300. |
[5] | D.S. Wightman and G. Lintern, “Part-task training for tracking and manual control,” Human Factors, vol. 7(3), 1985, pp. 267-283. |
[6] | P.B. Komotski and V.P. Salnitski, “On the question of quality evaluation of ergonomic control processes,” In: V.N. Tschernigovski (Ed.) Problemy kosmicheskoi biologii, vol. 34. Nauka, 1977, pp. 72-82. |
[7] | B. Johannes, V.P. Salnitski, A.V. Dudukin, L.G. Shevchenko, A.E. Shebuchev, S.V. Bronnikov, 2016, “Performance Assessment in the Experiment PILOT on-board Space Stations MIR and ISS.” Aerosp Med Hum Perform, vol. 87(6), pp. 534-544. DOI: 10.3357/AMHP.4433.2016 ISSN 2375-6314. |
[8] | E. Mueller, K. Bilimoria, C. Frost, 2008, "Handling Qualities Evaluation for Spacecraft Docking in Low Earth Orbit," in AIAA Guidance, Navigation, and Control (GNC) Conference, Honolulu, HI. |
[9] | K.R. Duda, Z. Prasov, J.J. West, and S.K. Robinson, “Development of an integrated simulation platform for real-time task performance assessment,” IEEE Aerospace Conference, 2015. |
[10] | B. Johannes B, A.K.W. Gaillard, S. V. Bronnikov, Y.A. Bubeev, T.I. Kotrovskaya, J. Rittweger, 2018, P300 assessment of cognitive capacity during the control of an object with six degrees of freedom. 89th Annual Scientific ASMA Meeting, May 4 – May 11 2018, Dallas, TX, AMHP, 89(3) 255. |
[11] | Johannes B, Salnitski VP, Soll H, Rauch M, Hoermann HJ. (2008) De-individualized psychophysiological strain assessment during a flight simulation test – validation of a space methodology. Acta Astonautica 63, 791-799. |
[12] | N.C. Jordan, J.H. Saleh, D.J. Newman, 2006, The extravehicular mobility unit: A review of environment, requirements, and design changes in the US spacesuit," Acta Astronautica, Vol. 59, No. 12, 2006, pp. 1135{1145. |
[13] | P. Handley, 2014, "A Pilot Model for the NASA Simplified Aid for EVA Rescue (SAFER) (Single-Axis Pitch Task)," M.S., Mechanical and Aeronautical Engineering, University of California - Davis, Davis, CA. |