Jonelle Cornette1, Clairmont Clementson2, David Fredericks2
1Research Assistant, University of Guyana, Turkeyen, East Coast Demerara, Guyana
2Research Scientist, National Agricultural Research and Extension Institute, Mon Repos, East Coast Demerara, Guyana
Correspondence to: Clairmont Clementson, Research Scientist, National Agricultural Research and Extension Institute, Mon Repos, East Coast Demerara, Guyana.
Email: |  |
Copyright © 2018 Scientific & Academic Publishing. All Rights Reserved.
This work is licensed under the Creative Commons Attribution International License (CC BY).
http://creativecommons.org/licenses/by/4.0/

Abstract
The increase of greenhouse gasses and chemical fertilizer use due to the increasing energy needs and industrial revolution have contributed to climate change concerns prompting the need for alternative environmentally friendly and renewable sources. Water Hyacinth is a rapid growing water weed whose management presents a challenge to the drainage system and a breeding area for mosquitoes thereby enabling the spread of diseases. This research seeks to highlight an opportunity to derive economic and environmental benefits from water hyacinth thereby mitigating against environmental management issues. It characterizes biochar produced from water hyacinth at three pyrolysis temperatures and examined its suitability as a soil amendment along with its energy and carbon capture potential. Increasing the pyrolysis temperature transformed the biomass matrices into a lighter and porous structure causing a decrease in density. There was an increase in ash and fixed carbon content while volatile matter decreased as pyrolysis temperature increased indicating a higher concentration of organic matter are available for carbon sequestration at higher pyrolytic temperatures. Further, the presents of micro-nutrients and high pH makes biochar from these water hyacinth samples suitable as a liming agent and a soil amendment for vegetables, legumes and grains in open-field or shaded conditions. Additionally, the biochar is recommended as a fertilization and soil improvement additive to aid Guyana’s agricultural production expansion into its Hilly Sand and Clay Region.
Keywords:
Water hyacinth, Biochar, Soil amendment, Carbon sequestration, Drainage control
Cite this paper: Jonelle Cornette, Clairmont Clementson, David Fredericks, Environmentally Sustainable Management of Water Hyacinth (Eichhornia crassipes) in Guyana, International Journal of Agriculture and Forestry, Vol. 8 No. 1, 2018, pp. 4-9. doi: 10.5923/j.ijaf.20180801.02.
1. Introduction
The increase in greenhouse gas emissions from energy needs worldwide has prompted significant research into the development of renewable energy sources. These include the conversion of biomass into biofuels and other value-added renewable products. Biomass is any organic matter produced by photosynthesis existing on the earth’s surface. It is the naturally occurring energy-containing carbon resource that is large enough in quantity to be used as a substitute for fossil fuels (Balat et al., 2009). One such biomass derivative, biochar, has been proven to be a valuable resource for carbon sequestrate also a medium for the immobilization of organic contaminants in soil (Cao et al., 2009). It reduces the need for chemical fertilizer, resulting in decreased emissions from fertilizer production, increases soil microbial life which results in more carbon storage in soil while retaining more nitrogen. Biochar is a fine-grained porous substance obtained from the pyrolysis of biomass. Pyrolysis is a relatively simple technique in which organic material is heated in the absence of oxygen. During the pyrolysis process, the natural polymeric constituents (i.e. lignin, cellulose, fats and starches) are thermally broken down into three different fractions: bio-oil (condensed vapors), char (solid fraction) and non-condensable gases. The solid or char fraction obtained from biomass pyrolysis is often considered a waste product and combusted to provide the necessary heat for the pyrolysis process. However, recent research suggests that char can be used as a soil amendment, hence termed biochar, to substantially increase soil fertility (Lehmann et al., 2006). The use of biochar for remediation is not a new concept since it has been found to be an ancient practice in areas of the fertile Amazon Basin. The terra preta soils of the region received large amounts of charred organic debris from the former inhabitants. As a result, carbon stocks have remained in the soil, even thousands of years after abandonment (Kwapinski et al., 2010; Lehmann et al, 2006).In Guyana water hyacinth (Eichhornia crassipes) has impeded water flow in irrigation and drainage canals resulting in eutrophication and provide or enhance breeding places for disease-carrying mosquitoes. It exhibits rapid growth and can spread quickly making it very difficult to control once it becomes fully established. Integrated control of water hyacinth can ensure economic and environmental benefits while keeping the population of this weed in check. This study proposes the use of water hyacinth to produce biochar which can then be used as a soil amendment and carbon sequester. It’s suitability as an organic fertilizer is premised on its low carbon: nitrogen ratio (C:N) of about 1:24.3 with a lignin content of only 9% compared with C: N ratio of about 1:80 and lignin content of 17% for other biomass such as wheat straw (Mallik et al., 1990). The weed is a good absorber of nitrogen, phosphorus and potassium from water. Further Jafari (2010) posited that on a zero-moisture basis, water hyacinth soil amendment contains as high as 75.8% organic matter, 1.5% N, and 24.2% ash. Additionally, with the ash containing 28.7% K2O, 1.8% Na2O, 12.8% CaO, 21.0% Cl, and 7.0% P2O5. Hence the general objective of this study is to assess the suitability of water hyacinth (Eichornia crassipes) biochar as a soil amendment. The specific objectives are: (i) To characterize the physico-chemical properties of water hyacinth (Eichornia crassipes) prior to biochar production, (ii) To characterize the physico-chemical properties of water hyacinth (Eichornia crassipes) after biochar production, and (iii) To determine the biomass to biochar ratio and value of biochar as a fertilizer.Biochar production by pyrolysis of biomass effectively removes carbon from the atmospheric carbon cycle, transferring it to long-term storage in soils. Biochar, therefore, could help in the global challenge of carbon dioxide (CO2) mitigation, as it results in a net removal of carbon from the atmosphere (Lehmann and Joseph, 2009), hence, the recalcitrant weed then becomes a valuable resource.
2. Material and Methods
Fresh water hyacinth was manually collected from the Dennis Street drainage canal in the Sophia area (latitude: 6.8169°; longitude: -58.1267°) of Georgetown, Guyana. This canal has an accumulation of surface runoff groundwater along with industrial and residential wastewater that provides an ideal environment for the growth of the water hyacinth plant. This canal is often clogged up with water hyacinth that prevents the free flow of water.The water hyacinth was taken to the Institute of Applied Science and Technology within the University of Guyana’s Turkeyen compound where they were cut into small pieces of approx. 20–40 mm, air dried 18 days with average weather conditions of 30° /22°C (daytime/nighttime) temperatures, 78% humidity and 35% cloud cover in daylight. The biomass was then placed in sealed (50kg) polythene bags and labelled WH300, WH450, and WH550 samples referring to the pyrolysis temperatures of 300°C, 450°C and 550°C used. These temperatures were chosen because different classes of complex organic polymers tend to decompose at varying peak temperatures based on structural composition. The biomass was ground/milled to pass through a 1 mm sieve with all respective equipment calibrated. The moisture content, ash content, volatile matter and fixed carbon of the grounded water hyacinth biomass was determined according to American Society for Testing and Materials (ASTM) testing codes E1756-01: Standard Test Method for Determination of Total Solids in Biomass, E1755-01: Standard Test Method for Ash in Biomass, E872-82: Standard Test Method for Volatile Matter in the Analysis of Particulate Wood Fuels and 870-82: Standard Test Method for Analysis of Wood Fuels respectively.To produce the biochar samples the crucibles were firstly sanitized, air dried then oven dried at 105°C for 1 hour. They were then removed, cooled in desiccators and weight recorded to the nearest 0.1 mg (crucible weight). 100g of ground samples in triplicate were placed in crucibles and this weight was recorded as the initial weight. The furnace was initially heated 250°C then at approximately 5°C/min to 300°C. The crucibles with raw biomass was placed in the furnace and carbonized for 40 minutes. Crucibles were removed from the furnace, cooled to room temperature in desiccators and final weight (final weight) was recorded to the nearest 0.1 mg. Biochar yield was then calculated using the formula: Biochar Yield (%) = (Final weight -weight of crucible) / (Initial weight – weight of crucible) × 100% The volume of biomass to biochar ratio was assessed by first determining their specific volume by the relationship, v = ρ-1 where the specific volume (v) of a substance is the reciprocal of density (ρ). This being an intrinsic property per unit mass.Biochar was then produced at carbonization temperatures of 450 and 550°C following the same procedure. These temperatures fall within the range of slow pyrolysis and lignin decomposition.After carbonization, the biochar was tested according to standards recommended by the International Biochar Institute (IBI) Biochar Standard version 2.0. The moisture content, ash content and volatile matter analysis of the biochar were conducted in triplicate using the ASTM D1762-84: Standard Test Method for Chemical Analysis of Wood Charcoal. The fixed carbon content and bulk density analysis were done according to ASTM E870-82: Standard Test Method for Analysis of Wood Fuels and ASTM D7481-09: Standard Test Methods for Determining Loose and Tapped Bulk Densities of Powders using a Graduated Cylinder respectively.Data collected were tabulated and statistical analyses were done to establish whether there was correlation between the physicochemical properties of the biomass and biochar, and further the trends of the biochar properties for different pyrolysis temperatures.Organic Carbon analyses were performed using Dichromate Method at the Guyana Sugar Corporation Inc. (GuySuCo) laboratory (La Bonne Intention, East Coast Demerara, Guyana) while macro and micro nutrients were determined using Kjeldahl Acid Digestion, Inductively Coupled Plasma Optical Emission Spectroscopy (ICP-OES) along with Inductively Coupled Plasma Mass Spectroscopy (ICP-MS) methods and pH was conducted using the DIN ISO 10390:2005 method at Activation Laboratories Limited (41 Bittern Street, Ancaster, Ontario L9G 4V5).
3. Results and Discussion
Biochar yield negatively correlated (R2 = 0.93) with increasing pyrolysis temperature (Figure 1). The highest yield of biochar (62.2%) was obtained at 300°C and decreased to (35.9%) as the temperature increased to 550°C. From this data, biochar yield (y) from water hyacinth by pyrolysis could be predicted by the regressive equation for pyrolysis temperature (x) as y = -0.1096x + 93.484.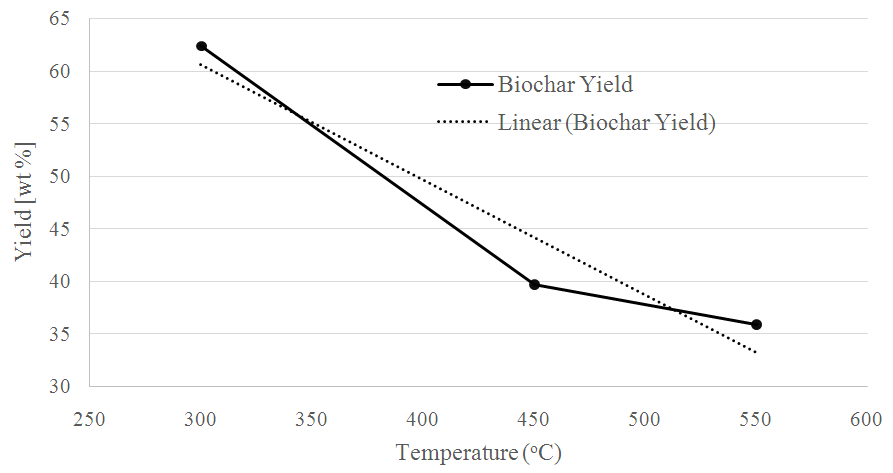 | Figure 1. The effect of pyrolysis temperature on biochar yield, expressed in wt. % of biomass feedstock |
Table 1 highlights the physiochemical properties of the ground water hyacinth biomass compared with the biochar produced from the three pyrolysis temperatures. The bulk density of the raw biomass was higher than of the biochar produced that decreased with an increase of pyrolysis temperature. Compositional changes took place during carbonization which resulted in corresponding bulk density alterations which were impacted by the temperature the material was exposed to. Organic matter decomposition caused the release of moisture, hemicellulose, cellulose, and lignin; all of which transformed biomass matrices into a lighter and more porous structure.Table 1. Bulk density and proximate analysis parameters of water hyacinth biomass and biochar 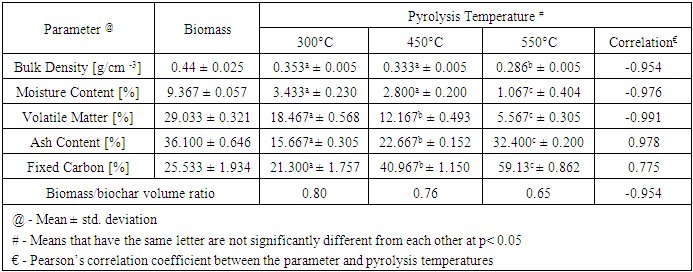 |
| |
|
The moisture content (R= -0.976) and volatile matter (R=-0.991) of the biochar decreased with increased pyrolysis temperature as more fluid particles and combustibles are driven off with greater heat intensity. This corresponds to the inference by previous biomass studies that at the lower the temperature more volatile organic compounds would be present causing it to be higher in mass (Thangalazhy-Gopakumar et al., 2010; Crombie et al, 2013). Differences in volatile material yields over the temperature range can be a result of the degree of breakdown of cellulose, hemicellulose and lignin (Lehmann and Joseph, 2009).The ash content (R= 0.978) and fixed carbon (R=0.775) of the water hyacinth biochar increased with the pyrolysis temperature. The ash content of the raw biomass was greater than the biochar produced at the 300°C and 450°C. The fixed carbon of the raw biomass was greater than the biochar produced at the 300°C. Ash content increased with rising pyrolysis temperature, which was owing to mass loss as temperature increased during carbonization leading to the build-up of high concentration of metallic elements. There was significant difference in the ash content, volatile matter and fixed carbon obtained from the pyrolysis temperatures (p≤0.05). Volatile matter followed the reverse pattern to of ash and fixed C; higher temperature pyrolysis therefore can minimize the biochar fraction susceptible to decay in soil while increasing by-products for heat and energy generation. This further suggests that certain organic functional properties enhanced at higher temperature could be acquired without diminishing C sequestration.Pyrolysis temperature and feedstock composition have a significant influence on the physical characteristics related to pore structure, surface area and adsorption properties (Antal and Gronli, 2003); (Downie et al., 2009); (Amonette and Joseph, 2009). As pyrolysis temperatures increase, volatile compounds in the feedstock matrix are lost, surface area and ash increase but surface functional groups that provide exchange sites decrease (Guo and Rockstraw, 2007). The proportions of these fractions remaining along with the ash content influence the level of reactivity of the char and the development of shifts in physical structure that define the char’s attributes (Downie, 2009). As pyrolysis temperatures increase, the poly-condensation of C into aromatic rings provides a structure that enhances pore development and surface area of the biochar. Biochars with large amounts C in these condensed structures formed at high temperatures (400-700°C) generally have lower functional groups for generating surface charge and ion exchange due to decarboxylation.The pH of the ground water hyacinth ranged from 6.5 to 7.4 which correspond with findings from (Cabi, 2015) that inferred Eichornia prefers a low pH, specifically under 8 for optimum growth. The average pH of the biochar samples was 8.1, indicating an enrichment of basic cations in the ashes which may be associated with alkaline species (carbonates, oxides and hydroxides) and also a reduction in the concentration of acidic surface functional groups (Yuan, Xu, & Zhang, 2011).Increase in carbon concentrations with a rise in pyrolysis temperature occurred due to higher degree of polymerization, leading to a more condensed carbon structure in the biochar (Lehmann and Joseph, 2009). The highest C content occurred at 450°C and it can be inferred that optimum carbonization took place at this temperature. The high carbon content of biochar is advantageous in terms of maximizing the amount of carbon storage and could be used as an energy resource or for soil adsorption of pollutants such as persistent organic pollutants (POPs) and inorganic pollutants.Table 2. Elemental Composition of Biochars Produced 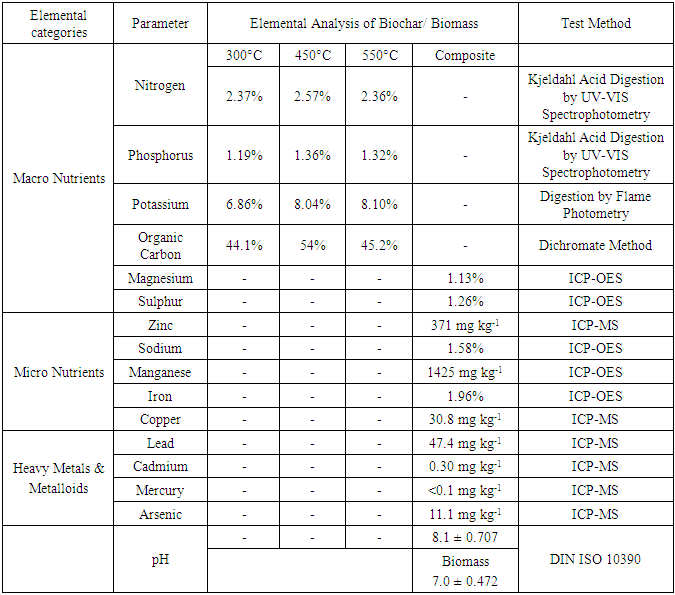 |
| |
|
The total N content for the chars declined with increased pyrolysis temperature–suggesting an ignition loss of N during pyrolysis and thus cannot be regarded as nitrogen fertilizer. This analysis was limited by the inability to test the total carbon percentage.Potassium increased with temperature increasing ions became more excited overtime as stability changed. In general, the biochars contained all or most nutrients below threshold limits and thus could be considered suitable as soil amendments in respect to the environment since plants can readily absorption them (Lehmann and Joseph, 2009).Biochar can act as a liming agent thereby increasing the soil pH. However, too much liming agent causes manganese deficiency in the soil and ultimately the crop. The chemical properties of biochar from water hyacinth are correlated with the chemical properties of its growth environment. Therefore, the growth and use of water hyacinth free from toxic elements for biochar production is encouraged. Similarly, water hyacinth proliferating in waterways near rice fields is a direct result of nutrient rich runoff from these fields. Given the nature of water hyacinth to extract and store these nutrients in its biomass, the opportunity exists to harvest, concentrate and return the nutrients to the fields through bio-char. The bio-char produced in this study could be used in open-field and shaded cultivations as an alternative to vermi-compost for crops like vegetables (lettuce and tomatoes), legumes (peas and beans) and grains (corn and rice).Further, Guyana’s economy that rely heavily on its agricultural base justifies the need for the development of its vast interior for agricultural purposes. In Guyana, there is an abundance (34,809 km2) of sand and sandy loam soils (800 Soil Series) located in the Intermediate Savannahs and the Soesdyke Highway (Hilly Sand and Clay Region) that is presently under-utilized but could be improved for agricultural production. These soils are extremely fragile, acidic, excessively drained and low in natural fertility. These soils will require an amendment to make them productive. The application of biochar to these soils will improve their quality and enhance their potential for agricultural production.
4. Conclusions
This research seeks to highlight an opportunity to derive economic and environmental benefits from water hyacinth thereby mitigating against environmental management issues. In this study biochars were produced from water hyacinth at three pyrolysis temperatures (300, 450 and 550°C), and their properties characterized. Laboratory experiments were conducted to investigate how the physicochemical properties of the biomass change due to thermal processing. Increasing the pyrolysis temperature transformed the biomass matrices into a lighter and porous structure causing a decrease in density. There significant difference in the ash content, volatile matter and fixed carbon obtained from the pyrolysis temperatures (p≤0.05). Ash content and fixed carbon content increased while volatile matter decreased with pyrolysis temperature indicating a higher concentration of organic matter available for carbon sequestration at higher pyrolytic temperatures. Further, the presents of micro-nutrients and high pH makes biochar from these water hyacinth samples suitable as a liming agent and a soil amendment for vegetables, legumes and grains in open-field or shaded conditions. Also, the biochar is recommended as a fertilization and soil improvement additive to aid the expansion of Guyana’s agricultural production into its Hilly Sand and Clay Region. Field trials should be done to compare the efficacy of this soil amendment to chemical fertilizer and other natural sources.
References
[1] | Amonette, J. and S. Joseph. 2009. Characteristics of biochar: Micro chemical properties. In Lehmann, J. and S. Joseph (Eds.). Biochar for environmental management: Science and Technology, Earthscan, London, 33-52. |
[2] | Antal, M.J. and M. Gronli. 2003. The art, science and technology of charcoal production. Industrial and Engineering Chemistry Research 42(8): 1619-1640. |
[3] | Balat, M., Balat, M., Kirtay, E. and H. Balat. 2009. Main routes for the thermo-conversion of biomass into fuels and chemicals. Part 1: Pyrolysis systems. Energy Conversion and Management 50(12): 3147–3157. |
[4] | Cabi. 2015. Eichornia crassipes (water hyacinth). Retrieved 5/11/2017 from http://www.cabi.org/isc/datasheet/20544. |
[5] | Cao, X., Ma, L., Bin, G. and W. Harris. 2009. Dairy-Manure Derived Biochar Effectively Sorbs Lead and Atrazine. Environmental Science and Technology 43(9): 3285-3291. |
[6] | Crombie, K., Masek, O., Sohi, S.P., Brownsort, P. and A. Cross. 2013. The effect of pyrolysis conditions on biochar stability as determined by three methods. Global Climate Biology Bioenergy 5(2): 122–131. |
[7] | Downie, A., Crosky, A. and P. Munroe. 2009. Physical Properties of Biochar. In: Lehmann, J. and S. Joseph (Eds). Biochar for Environmental Management: Science and Technology, Earthscan, London, 13-32. |
[8] | Guo, Y. and D. A Rockstraw. 2007. Physiochemical properties of carbons prepared from pecan shells by phosphoric acid activation. Bioresource Technology 98(8): 1513-1521. |
[9] | Jafari, N. 2010. Ecological and socio-economic utilization of water hyacinth (Eichhornia crassipes Mart. Solms). Journal of Applied Sciences Environmental Management 14(2): 43–49. |
[10] | Kwapinski, W, Byrne, C., Kryachko, E., Wolfram, P., Adley, C., Leahy, J., Novotny, E. and M. Hayes. 2010. Biochar from biomass and waste. Waste and Biomass Valorization 1(2): 177-189. |
[11] | Lehmann, J., Gaunt, J. and M. Rondon. 2006. Biochar Sequestration in Terrestrial Ecosystems-A Review. Mitigation and Adaptation Strategies for Global Change 11: 403-427. |
[12] | Lehmann, J. and S. Joseph. 2009. Biochar for Environmental Management:Science and Technology. Routledge, Abingdon, United Kingdom. |
[13] | Mallik, M. K., Singh, U.K. and N. Ahmed. 1990. Batch digester studies on biogas production from Cannabis sativa, water hyacinth and crop wastes mixed with dung and poultry litter. Biological Wastes 31(4): 315-319. |
[14] | Thangalazhy-Gopakumar, S., Adhikari, S., Ravindran, H., Gupta, R.B., Fasina, O., Tu, M. and S.D. Fernando. 2010). Physiochemical properties of bio-oil produced at various temperatures from pine wood using an auger reactor. Bioresource Technology 101(21): 8389–8395. |
[15] | Yuan, J. H., Xu, R. K., and H. Zhang. 2011. The forms of alkalis in the biochar produced from crop residues at different temperatures. Bioresource Technology 102(3): 3488–3497. |