Nuamah Daniel O. B.1, Tandoh Kingsley K.2, Brako A. Blestmond3
1Department of Geophysics, University of Miskolc, Hungary
2Department of Oil and Gas Engineering, All Nations University College, Koforidua, Ghana
3Department of Geological Engineering, Kwame Nkrumah University of Science and Technology, Kumasi, Ghana
Correspondence to: Nuamah Daniel O. B., Department of Geophysics, University of Miskolc, Hungary.
Email: |  |
Copyright © 2019 The Author(s). Published by Scientific & Academic Publishing.
This work is licensed under the Creative Commons Attribution International License (CC BY).
http://creativecommons.org/licenses/by/4.0/

Abstract
Statistical applications such as correlation, factor analyses and spatial distribution plots have been applied to soils in Akuse area, South-eastern Ghana, to determine minor and trace elements sources, distribution patterns and probable causes for such distributions. Composite soil samples taken on a grid of 1km×1km from the study area showed twenty-five minor and trace elements namely V, Cr, Co, Ni, Cu, Zn, Ga, Rb, Sr, Y, Zr, Nb, Mo, Sb, Cs, Ba, La, Ce, Hf, Ta, Pb, Bi, Th and U. From the statistical mean analyses, Chromium (Cr) showed the highest mean concentration, followed by Zircon (Zr), Strontium (Sr), Barium (Ba) and Vanadium (v). Elements such as Nickel, Copper, Zinc and Cerium showed average mean values ranging from 84.3ppm-47.9ppm. The rest of the elements had lower mean concentrations indicating lesser concentrations in the study area. Elemental association in the study environment were shown by the Pearson Correlation Coefficient. Element pairs such as Cr-Ni, Zn-Rb, Ni-Ba, Zn-Ba and Cu-Zn showed strongly positive correlation coefficient, indicating their occurrence together in the study area. Others such as Zn-Zr and Ga-Zr were negatively correlated as they do not occur together. The applied factor analyses categorized the sources of the elements into natural and anthropogenic with some elements originating from both sources. Plotted Geochemical contours showed the central portion mainly covered by pyroxenites lithology had high concentrations of arsenic, barium, cerium, cobalt, gallium and nickel whilst the northwest had higher concentrations of copper, zinc and rubidium. This distribution was attributed to topographic features, changes in the geology of the bedrock and anthropogenic factors. Arsenic, Bismuth and Thorium were relatively less in concentrations as compared to other elements. The distribution of Cr, Co, Ni, Rb, Th and Ba differentiated the geology of the area and hence distinguished between felsic, basic and ultra-basic precursors. The wide range in trace element characteristics of the soil is an indication for inefficient mixing of the various source rock lithologies during the soil formation processes. The basic gneisses which cover most parts of the survey area showed relatively low concentrations in minor and trace elements as compared to areas covered by pyroxenites intrusions.
Keywords:
Trace elements, Statistical analyses, Spatial analyses, Correlation analyses, Factor analyses
Cite this paper: Nuamah Daniel O. B., Tandoh Kingsley K., Brako A. Blestmond, Geochemistry of Minor and Trace Elements in Soils of Akuse Area, Southeastern Ghana, Geosciences, Vol. 9 No. 1, 2019, pp. 8-17. doi: 10.5923/j.geo.20190901.02.
Article Outline
- 1. Introduction
- 2. Geology and Tectonic Settings
- 3. Methodology
- 3.1. Sampling Procedures
- 3.2. Analytical Procedures
- 4. Results and Interpretation
- 4.1. Statistical Analyses
- 4.2. Correlation Analyses
- 4.2.1. Large Ion Lithophiles Elements (LILE): [Rb, Cs, Ba, Sr, Th, U]
- 4.2.2. High Field Strength Elements (HFSE): [Zr, Hf, Ta, Nb]
- 4.2.3. Rare Earth Elements (REES): [La, Ce, Y]
- 4.2.4. Transition Trace Elements (TTE): [Cr, Co, Ni, Zn, V, Cu]
- 4.3. Factor Analyses
- 4.4. Spatial Distribution Analysis
- 4.5. Geochemical Pathfinder Study
- 5. Conclusions
- ACKNOWLEDGEMENTS
1. Introduction
The study area (Figure 1) forms part of the Accra plains in south-eastern Ghana, lying between latitude 5°45 south and 6°05′′ North and Longitude 0°05 East and 0°20 West. The relief of the area is generally gentle and undulating with few highlands. The uplifted nature of the area leads to higher rates of physical erosion exposing fresh bedrock to the environment thereby facilitating chemical weathering of the rocks. Akuse’s subtropical to tropical climate also makes it very susceptible to some of the heaviest amounts of rainfall. Heavy metals are found universally in both polluted and unpolluted environments. The level of heavy metals in the environment varies between different regions (Morais et al., 2012). Although heavy metals occur naturally in the earth’s crust, they tend to be concentrated in agricultural soils because of the application of commercial fertilizers, manures and sewage sludge containing heavy metals (Zeng et al., 2008). Soils in the area are mostly affected by human activities as farming is the main source of income for the indigenes. The purpose of this research was to determine the distribution of minor and trace elements in the area, determine the probable causes for these distributions, analyze their association with some precious metals and provide valuable information regarding the origin of the rocks in the study area. The popularity of residual soil surveying as an exploration method is a simple reflection of the reliability of soil anomalies as ore guide (Gill, 1997). Mineralized parent rocks always introduce some kind of chemical pattern in residual soils originating from weathering of the rock. Weathering of ore deposits leads to redistribution of elements in secondary dispersion halos in weathered rocks, soils, vegetation, and drainage systems. This vastly increases the area in which evidence for the presence of element mineral target can be detected. Elemental redistribution could be through mechanical movement of fragments under gravity, movement through diffusion or ionic movement of solution. Chemically, the distribution of minor and trace elements with high lithophile affinity is largely controlled by their ionic radii or size. Such elements with radii similar to those of major elements can substitute for them in the common minerals of igneous rocks. The crystal structures of these minerals act as sorting mechanisms, accepting those atoms of appropriate size and rejecting others. The idea of ionic size, coupled with geochemical affinity is key to the distinction between abundance and availability. Elements that are similar in size with geochemical affinity to major elements are dispersed in small amounts in common minerals hence their minute availability in soils. Other elements that do not readily enter the common minerals of igneous rocks remain in the residual melt as crystallization proceeds. Minor and trace elements can therefore be used to determine the origin, igneous processes (Berlo et al. 2004; Norman, M, Garcia, M and Pietruszka, A 2005) and crystallization history of igneous rocks (Norman, M, Garcia, M and Pietruszka, A 2005). They also act as geochemical pathfinders as most of them are associated with precious metals. Minor and trace elements easily disperse around mineralization, creating a wide dispersion halo. As pathfinders, they must be sufficiently abundant, so that the halo created is distinguishable from the surrounding background values.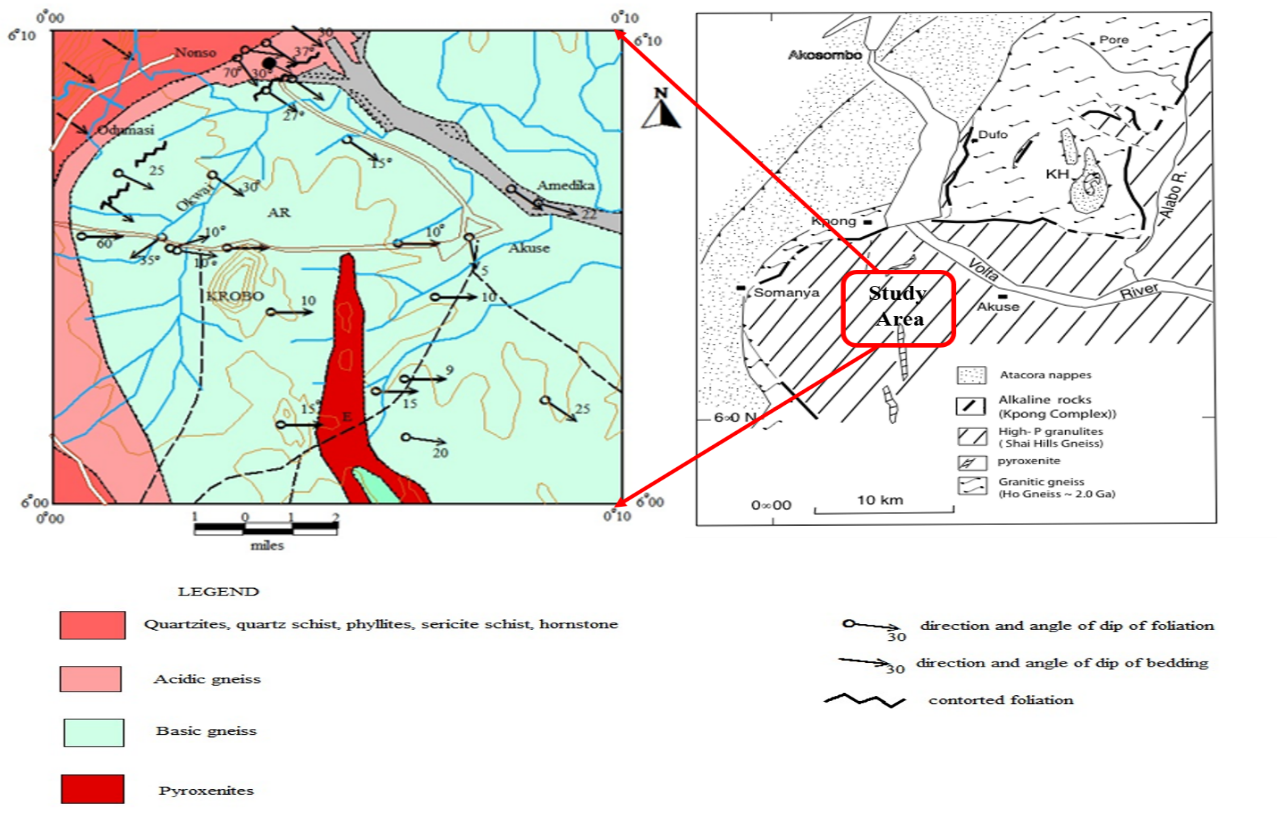 | Figure 1. Diagrammatic illustration of the study area |
2. Geology and Tectonic Settings
Tectonically, the study area form part of the Trans-Saharan belt (Figure 2) exposed in south eastern Ghana and adjoining parts of Togo and Benin, namely the Dahomeyide Orogenic Settings (Affaton, P., Rahman, M.A., Trompette, R., and Sougy, J., 1991; Castaing, C., Feybesse, J.L., Thieblemont, D. Triboulet C. and Chevremont, P. 1994; Attoh, K., Dallmeyer, R.D., and Affaton P., 1997), precisely the Accra Plains area. The principal tectonic elements of the Dahomeyide Orogen are the deformed edge of the West African Craton with its cover rocks consisting of craton verging nappes and thrust sheets bounded by ductile shear zones, the suture zone representing the eastern boundary of the autochthonous West African Craton and exotic rocks that form the granitoid gneiss complexes east of the suture zone (Attoh kodjopa and Nude P.M 2008). Metamorphism is generally in the amphibolite facies (Attoh, K., Hawkins, D., Bowring, S., Allen, B., 1991; Nude et, al. 2009). Dahomeyan gneiss and schist occupy most of the plains cover. Basic gneiss forms a number of large inselbergs in the north and center of the study area. The eastern belt of acidic gneiss consists mainly of grained metamorphosed rocks richer in minerals than rocks in the west. Gneisses with garnet, pyroxene and scapolite occur among more ordinary quartzo-feldspathic biotite and hornblende-bearing rocks (Attoh, K., Hawkins, D., Bowring, S., Allen, B., 1991). Eclogites have been recorded from large masses of mafic gneisses that include amphibolites and pyroxenites and contain much garnet (Nude et, al. 2009). In southeastern Ghana, the High Pressure mafic granulites have been referred to as Shai Hills gneiss (Attoh, K., Dallmeyer, R.D., and Affaton P., 1997), and are tectonically juxtaposed with the alkaline gneiss complex in the suture zone. Rocks of the Shai Hills Gneiss unit are folded into west and southwest verging nappes and crop out in inselbergs of the Accra Plains. Most of the isolated hills in the area are asymmetrical with steep, west-facing scarp slopes such as the Krobo and prominent Osu Yongwa Hills. The distinctive features of the rocks exposed on these hills are the prominent modal layering and extensive veining. The layering is discontinuous and consists of alternating garnet-rich and hornblende-rich zones giving the rock a streaky appearance and are interpreted to be shear induced. Quartz veins occur in all sizes and oriented to the tectonic layering in these rocks.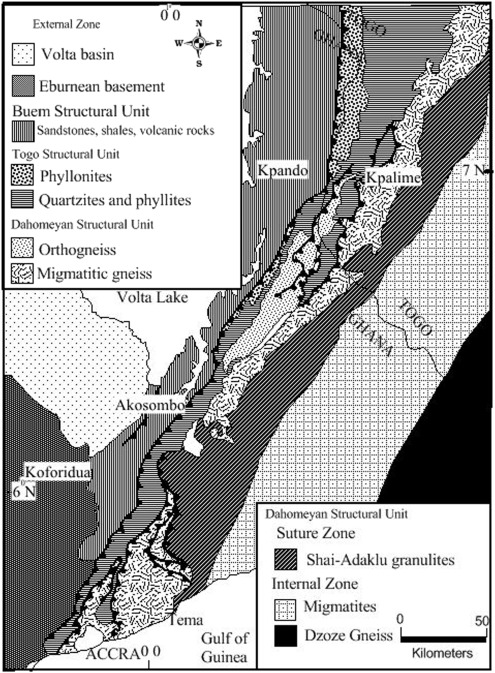 | Figure 2. Tectonic map of south eastern Ghana (modified by Nude et al, 2015) |
3. Methodology
3.1. Sampling Procedures
The sampling protocol employed in this exploratory work is soil sampling. This was determined based on the time and resources available, the indicators to measure and the data sources to collect information from. To maximize the statistical reliability of the project, a 1km x 1 km sampling grid, displayed on 1: 250,000 quadrangle maps was applied. Each grid or cell was identified by a quadrangle name, a cell column and row position. A total of 34 soil samples (Table 1, A1-F4) were taken by six groups. After locating a sampling point through global positing system (GPS) navigations, the location is input into GPS for further work. Samples were then taken at a depth range of 50-100cm intended to remove the top soil and labeled in a sample bag with an approximate weight of 2-3kg. A metal steel was labeled and dipped into the sample. Accuracy was maximized as plastic sample bags were labeled at each sample point using a permanent marker. Labelling prevented sample loss and mixing of samples from different sample locations. Important descriptions and documentations were made about the sample area and the sampled material through the filling of a field sheet with information such as site type, date and time, collector’s group name, site weather conditions, topography present, sample ID, bottom depth sampling, sampling interval, the soil horizon, soil color, soil texture, soil moisture condition and drainage conditions.
3.2. Analytical Procedures
The analytical procedure applied followed Asiedu et al. 2007. Trace element concentrations of soils were determined on powdered samples by an energy-dispersive polarizing X-ray fluorescence spectrometer (SPECTRO X-LAB 2000) at the Geological Survey Department. The well calibrated XRF used gave very accurate results with high precision, measuring most of the common metals in alloys such as iron, nickel and copper to less than 0.01%. X-Ray Fluorescence spectrometer (XRF) was employed due to it relatively high detection limit and effectiveness for locating geochemical contrast in elements. The technology combines traditional X-ray fluorescence methodology with small spot analysis. High intensity X-ray beams with diameters ranging from 3 mm down to a unique 10 µm offer versatile analysis capabilities. The samples were dried, crushed and sieved with 108 and 106 micrometre sieves to produce finer grains. In the laboratory, four (4) grams of each sample was mixed with 0.9 grams of wax. The mixture was homogenized with a homogenizer programmed to run 15 seconds per minute for 3 minutes. In order to prevent contamination, acetone was used to clean the sample cup thoroughly before use. Homogenized samples were sent to a manual press where pressure between 5 and 10 tonnes was applied to produce round pellets before analyses. This XRF system provided a complete elemental analyses and qualitative characterization. In all, thirty-four (34) soil samples were analysed (Table 1).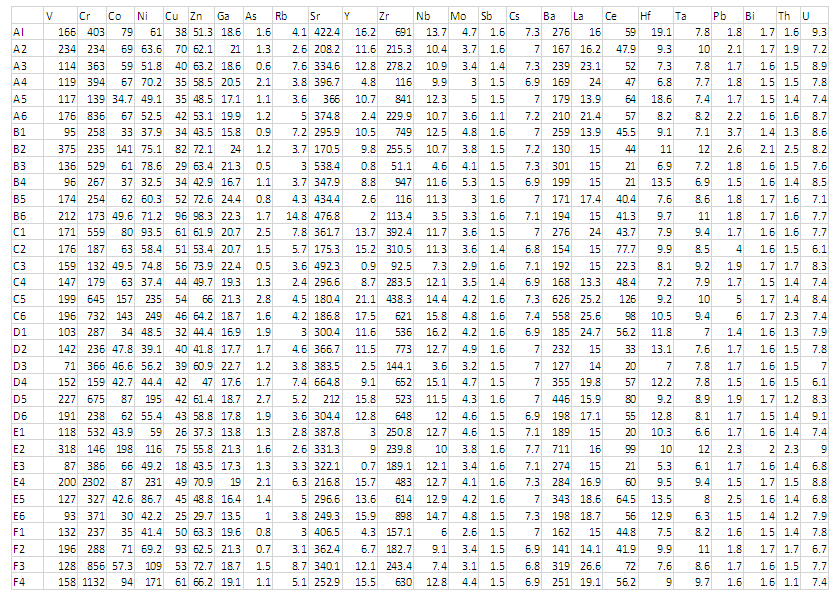 | Table 1. Trace-element geochemical data from soil samples collected from the study area in Part Per Million (ppm) |
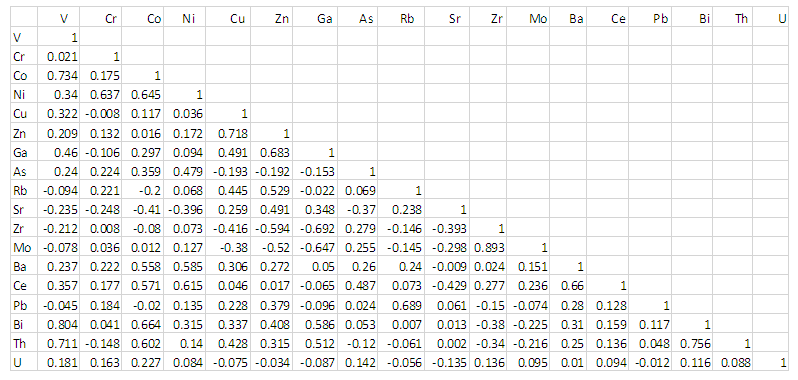 | Table 2. Pearson correlation coefficient of Trace-elements analyzed |
4. Results and Interpretation
4.1. Statistical Analyses
Table 3 shows the statistical analysis of the minor and trace elements in the study area. The table gives the mean concentration, the lower and upper limits as well as the standard deviation of each element. Chromium (Cr) shows the highest mean concentration. It is followed by Zircon (Zr), Strontium (sr), Barium (Ba) and Vanadium (v). Elements such as nickel, copper, zinc and cerium showed average mean values (84.3ppm-47.9ppm). Table 3. Statistical Analyses of elements in part per million 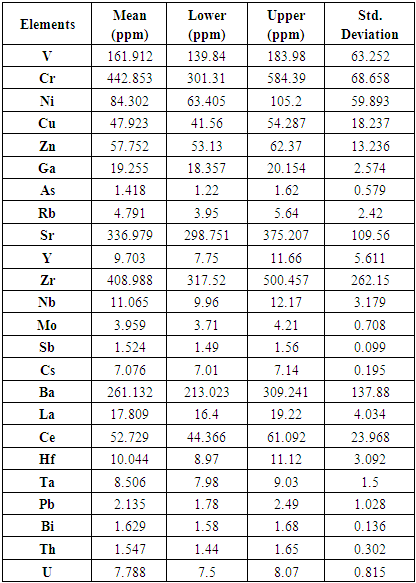 |
| |
|
The rest of the elements have lower mean concentrations indicating lesser concentrations in the study area. The higher concentration of Cr, Sr, Ba and V as indicated by their mean is attributed to the high mobility rates of these elements in the weathering environment, changes in lithologies and Contamination by human activities. Inversely, the lower mean concentrations of elements such as As, Sb, Bi and Th is as a result of lower concentration in bedrock, low mobility rate and topographic factors.
4.2. Correlation Analyses
Elemental associations of the analyzed trace elements Cr, Ni, Co, Sc, V, Cu, Pb, Zn, Cd, Ba, Sr, Zr, Ti, Rb, Hf, Th, U, Cs, Ta, Nb, Y and Ce, in the form of correlation coefficient ‘r’ (Table 2) were performed using SPSS suite. Element pairs such as Cu-Zn, Ba-Cr, Co-Ni and Ba-Ni (Figure 3) showed stronger association in the study environment whilst others such as V-Cr and Cr-Cu showed no association. A Positive correlation indicate that the elements pair occur together, thus, as the concentration of one element increases in a particular area within the study environment, there is a corresponding increase in concentration of the other element. Negatively correlated elements on the other hand has inverse relationship, thus, an increase in one element in the study environment result to a corresponding decrease in the other. While the non-correlated or sparsely correlated elements do not occur together in the study area.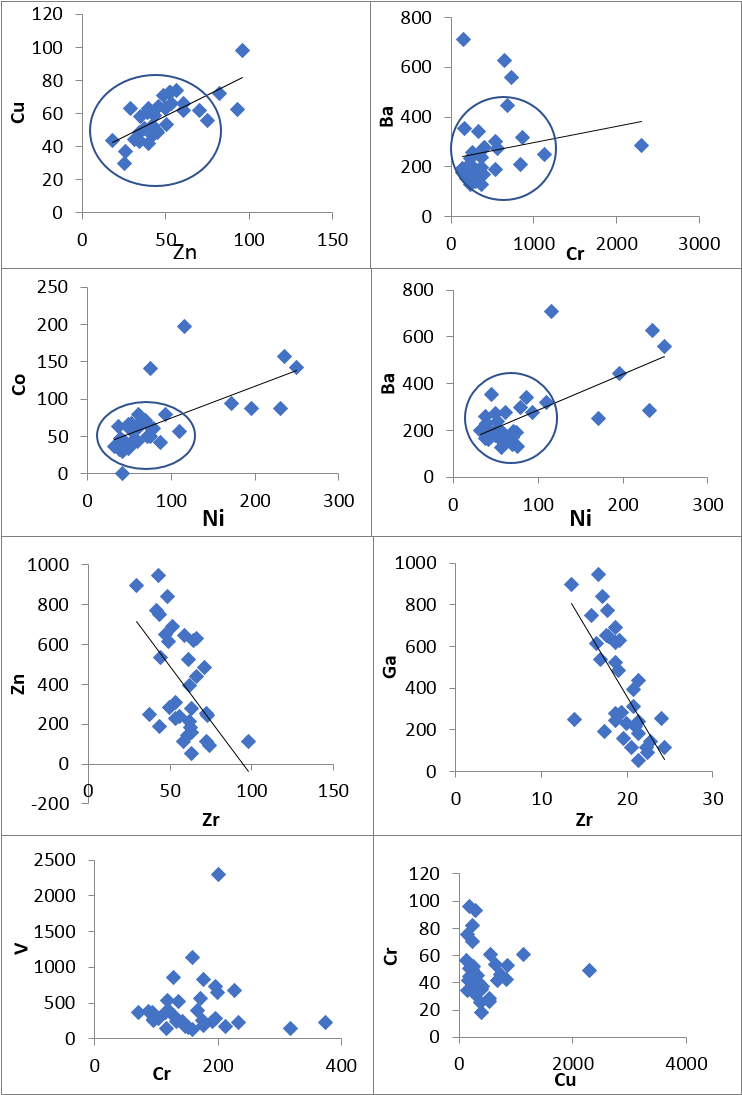 | Figure 3. Correlation plots of minor and trace elements |
4.2.1. Large Ion Lithophiles Elements (LILE): [Rb, Cs, Ba, Sr, Th, U]
These elements have high radius and low electric field. Rubidium (Rb) show significant positive correlation with zinc (r=0.529) and lead (r=0.689). Caesium on the other hand has stronger positive correlation with Cobalt and Barium but exhibited weaker positive correlation with the rest of the elements except Arsenic and Rubidium. Barium showed positive association with all the minor and trace elements except Strontium, with significant positive ‘r’ values between Cobalt, Nickel, and Cerium (r=0.558, 0.585 and 0.659 respectively). The correlation between Strontium and most of the element are poor, most of them negative. Similarly, Thorium ‘Th’ show relatively strong positive correlation with Vanadium ‘V’ (r=0.711), Tantalum ‘Ta’(r=0.590) and Bismuth ‘Bi’ (r=0.756).
4.2.2. High Field Strength Elements (HFSE): [Zr, Hf, Ta, Nb]
These are elements with smaller radius and higher charges. This elemental group correlated negatively among themselves except Nb and Zr. Both Zr and Hf of this group occur together with molybdenum ‘Mo’ (‘r’ = 0.893 and 0.679 respectively). Although, titanium (ta) occur with copper, zinc and gallium (‘r’ values=0.938, 0.718, 0.602 respectively) in the surveyed area, Niobium (Nb) correlated with copper, zinc, gallium and strontium negatively and hence may not occur together.
4.2.3. Rare Earth Elements (REES): [La, Ce, Y]
This group of elements positively correlated with themselves indicating their occurrence together in the weathering environment. They also showed greater association with some transition trace element such as Co, Ni, and As. Latium (La) of this group shows weaker correlation with almost all the minor and trace elements. The element yttrium ‘Y’ of this elemental group has significant positive correlation with most of the trace elements with the most outstanding association being that with zircon and niobium (‘r’=0.704 and 0.723 respectively) of the HFSE. It also exhibited significant negative correlation with Strontium. This is so because the element strontium is mostly associated with carbonate lithologies. The REE have been, to a large extent, diagenetically incorporated into the soil grains.
4.2.4. Transition Trace Elements (TTE): [Cr, Co, Ni, Zn, V, Cu]
The abundance of transition trace elements in the analytical result is highly valuable (table 1). Chromium (Cr), Cobalt (Co) and Nickels (Ni) are enriched in the study area. The transition trace elements mostly showed positive correlation with themselves, although, no significant correlation was observed between Chromium and elements such as Vanadium and Copper. Nickel correlated positively with both Chromium and Cobalt (‘r’ =0.637 and 0.645 respectively). Zinc (Zn) also showed positive correlation with Copper and a relatively strong negative relation with some HFS elements (Zr, Nb and Mo). Similar geochemical properties such as ionic size and radius accounted for the positive correlation observed among the transition trace elements Ni, Cr, Co, and Cu.
4.3. Factor Analyses
The factor analyses applied in this study is based on Joreskog (1963) model. It is a simple, very fast and non-iterative model which does not require an estimation of communalities but based on the population covariance matrix from which the factor loadings are calculated. The raw factor loadings (Table 4) were further rotated orthogonally to produce a simple structure of uncorrelated factors (Table 5) for interpretation. Factor extraction involves making a choice about the type of model as well as the number of factors to extract. Factor rotation comes after the factors are extracted, with the goal of achieving simple structure in order to improve interpretability. Orthogonal rotation produced factors that are independent or uncorrelated with each other. Three factors were extracted from the data which accounted for 99.982% of the total variance in the data set, indicating that the entire variance was almost explained by these axes. The first factor load accounts for 49.124% (V, Co, Cu, Zn, Ga, Rb, Ta, Bi, and Th) of the total variance, the second for 37.848% (As, Y, Ni, Sr, Zr, Nb, Mo, Sb, Cs, Ce, Pb and U) with the third containing 13.026% (Cr and Hf). The observed factors could be explained by elemental sources in the soil. Factor 1, showed significant positive load for all the minor and trace elements in this group and contains elements with naturally occurring concentration in the study area. Tantalum ‘Ta’ showed the highest load in this factor (load value 0.982). This was followed by Cu, V and Bi respectively. Although Ni and Ce were not classified in this factor, they showed significant loadings.Table 4. Factor loadings (raw) 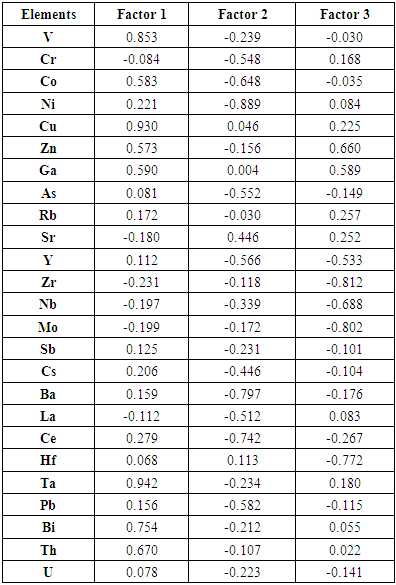 |
| |
|
Table 5. Factor loadings (rotated) 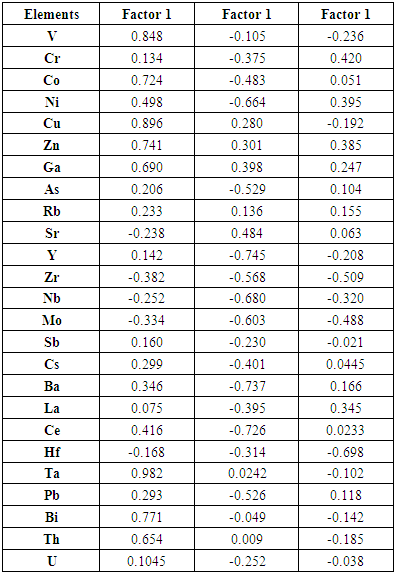 |
| |
|
The elements in this group were also significantly correlated, indicating that they are from the same source. Factor 2 contained minor and trace elements whose sources in terms of concentration in the study area can be classified as anthropogenic, hence, have varied contributions from human activities. This may be attributed to farming in the area coupled with fertilizer application and charcoal burning. They showed significant negative loads after a rotation of the factor loads and have concentrations exceeding natural background values in the area. Chromium ‘Cr’ and hafnium ‘Hf’ loaded in factor three (3) and can be explained by varied sources of both natural and anthropogenic. Figure 4 shows 2D plot of data variance and the extracted factor axes respectively. There is a reduction in the total variance in the datasets but significant outlier occurs in the variables spread.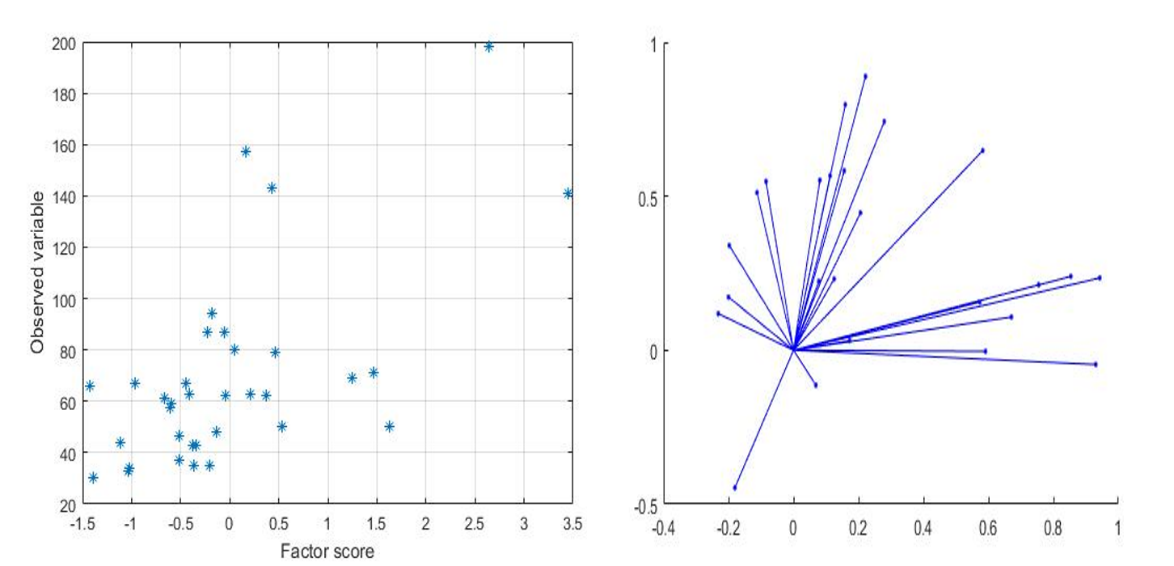 | Figure 4. A plot of factor scores and extracted factors axes respectively |
The biplot illustrates the orthogonal factor axes after factor rotation.
4.4. Spatial Distribution Analysis
Figure 5 show a plot of elemental distributions in the study area. Copper (Cu) and zinc (Zn) demonstrated almost the same pattern of distribution in the study area and may be attributed to their strong positive correlation and similar atomic size.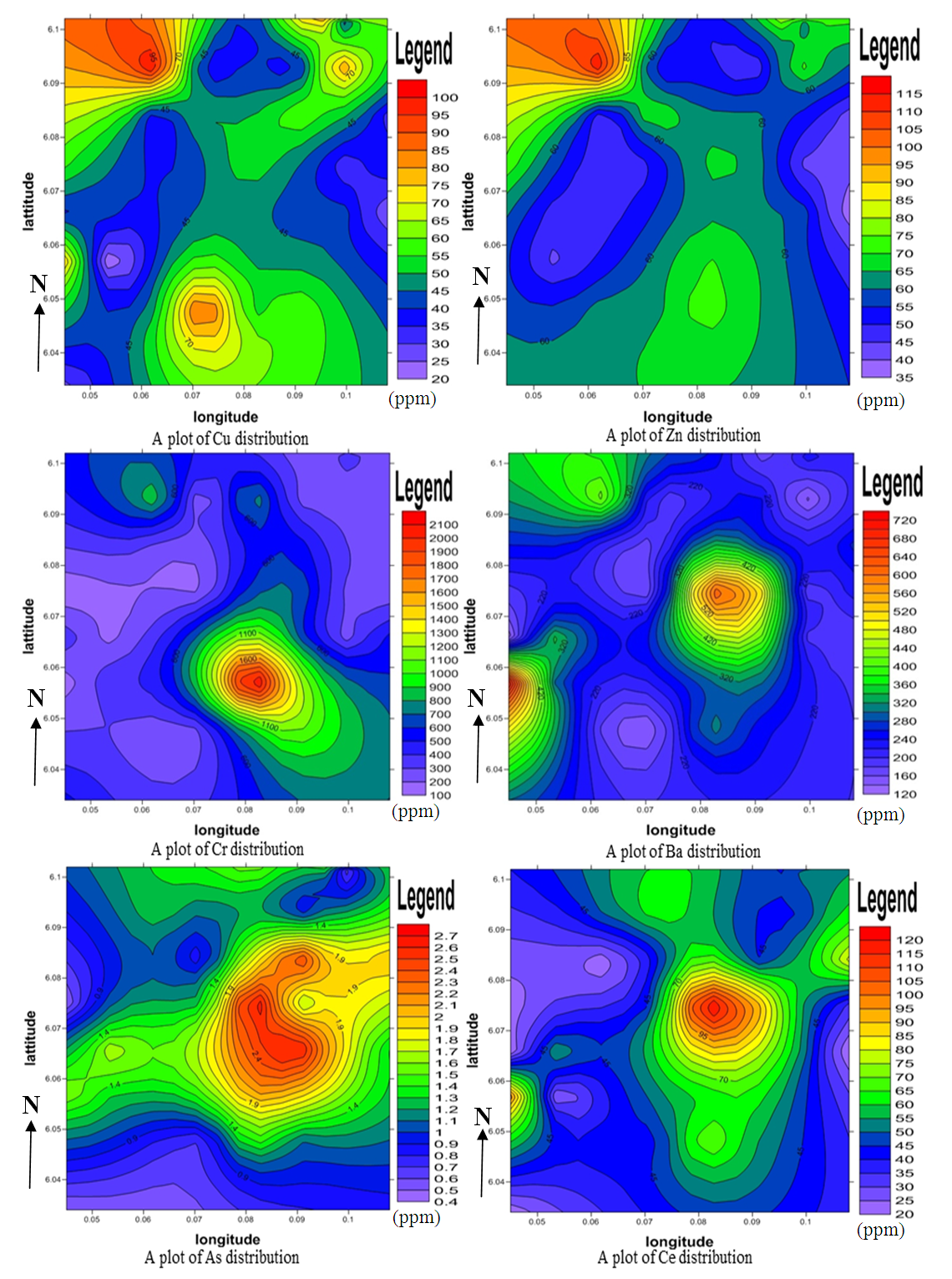 | Figure 5. A Plot of Elemental Distributions in Part Per Million (ppm) |
Higher concentrations occur in the north-west with ranges of 70ppm-100ppm and 85ppm-115ppm for Copper and Zinc respectively as indicated by their contour values. The north-east and southern portions exhibit average concentration of these elements with systematic decrease in concentration towards the central-east boundary. Chromium distribution is far less across most parts of the study area with concentration between 100-800ppm. The highest concentration occurs in the south below latitude 6.07E° with gradual increase from 1100ppm-2100ppm (the highest elemental concentration in the area). Barium (Ba) shows similar distribution to chromium. Relatively higher concentration occurs in the central and south-western boundaries with the rest of the area showing lower concentrations. Arsenic ‘As’ displays a spectacular distribution with most areas showing average concentration. The central portion has the highest concentration range of 1.9ppm-2.7ppm with lower concentration occurring in the south (latitude 6.05E˚ downwards). Cerium showed similar distribution trend to Arsenic with maximum concentration occurring in the central zone. A gradual increase in concentrations from average to high is observed in the south-west with the rest of the area showing lower concentrations. A comparison between the geochemical contour plots and the digitized lithologic map of the study area (Figure 1) indicate that Co, Ni and Cr have high concentrations mostly in the central portion of the study area. This is attributed to the occurrence of mafic rocks such as pyroxenites and amphibolite’s in the form of intrusions across the central area. The content of these mafic elements in the source pyroxenites intrusion is higher and might not have seen greater dispersion hence, their high concentration across the central portion. Elements such as chromium, cobalt and nickel showed a decrease in concentration in the basic gneisses covering most parts of the area of study. The north-western part of the study area shows higher concentration of Rb and Ba largely associated with high pressure acidic gneisses complexes scattered across the north and west. Gallium and molybdenum exhibited averagely high concentrations across the study area indicating high dispersion and mobility rates within the weathering environment.
4.5. Geochemical Pathfinder Study
Pathfinders are elemental associations which have a higher indicative signal for other elements. The distribution of minor elements such as zinc, copper, molybdenum, arsenic and lead in the study area may probably be associated with metallic minerals of economic value (Brian E. Davies 1971). Maximum concentrations of zinc and copper at the north-western part of the study area may be associated with sulphide and vein-type deposits. Chromium and molybdenum has distinctively high values in the middle of the area and may be associated with contact metamorphic deposits. Arsenic, a characteristic pathfinder of precious metals is higher in the central portion. Hidden prospect has strong and coincident pathfinder elemental responses showing cohesive patterns and this is highly observed in the central and northwestern areas. Geochemical anomalous trends remained open across and along strike. The geochemical responses show a prospective area for nickel sulphide and other mineralization, however the geological setting may be either a mafic/ultramafic sequence as indicated by the pyroxenites and basic gneisses.
5. Conclusions
The study showed that: (i) V, Co, Cu, Zn, Ga, Rb, Ta, Bi, and Th were associated with parent materials and therefore had natural sources, thus, the weathering of parent materials and subsequent pedogenesis due to the alluvial deposits. (ii) As, Y, Ni, Sr, Zr, Nb, Mo, Sb, Cs, Ce, Pb and U had anthropogenic sources (probably from over use of chemical fertilizers and pesticides, industrial and discharges, animal wastes, sewage irrigation, etc.), (iii) Cr and Hf showed varied sources of both natural and anthropogenic. Dispersion pattern of minor and trace elements observed in the study area may be attributed to topographic factors (hills and inselbergs as well as flat plains), climatic factors (temperature and rainfall in the area), anthropogenic factors and the chemical environment (whether oxidizing or reducing). The distribution of Cr, Co, Ni, Rb, Th and Ba differentiate the geology of the area and hence distinguishes between felsic, basic and ultra-basic precursors. The soil originated from felsic and ultrabasic source rocks such as pyroxenites intrusion, amphibolites and gneissic complexes. The basic gneisses showed low concentrations of minor and trace elements as compared to the pyroxenites intrusion. The wide range in trace element characteristics of the soil argue for inefficient mixing of the various source rock lithologies during the soil formation processes. The observed relatively strong correlation of most elements is attributed to similarities in geochemical behavior such as ionic size, ionic radius and mobility rates in the weathering environment. The significant correlation of mafic and felsic elements such as Ni-Ba is as a result of weathering together of different lithologies of mafic and felsic provenance. Among the factors affecting the distribution of element in the soil are the abundance of the element in the parent rock and the nature of the weathering in the Akuse area. From the geochemical responses of some pathfinder elements, the north-western part of the study area shows a higher prospective for sulphide and vein-type deposits whilst the central portion may be associated with nickel sulphide and other mineralization.Although, no previous distinct elemental background values have been determined for the study area, an increase in the occurrence of heavy metals such as Cr, Pb, Ni, Zn and Ba beyond the World Health Organization (WHO) required levels would have serious environmental consequence because these metals can cause changes in the structure and functioning of the soil ecosystem. The availability of heavy metals at higher concentrations in soils has an influence on plant growth and development. It has the capacity to interfere with the physiological and biochemical functions of plants which adversely affect them. Higher concentration of heavy metals in food crops and plants leads to toxicity in humans and animals as metals are known to cause several damages to human organs, even at lower level of exposure.
ACKNOWLEDGEMENTS
The described article was carried out as part of the EFOP-3.6.1-16-2016-00011 “Younger and Renewing University – Innovative Knowledge City – institutional development of the University of Miskolc aiming at intelligent specialization” project implemented in the framework of the Szechenyi 2020 program. The realization of this project is supported by the European Union, co-financed by the European Social Fund.
References
[1] | Asiedu D.K., S.B. Dampare, P. Asamoah Sakyi and D. Boamah, (2007). Major and trace-element geochemistry of kimberlitic rocks in the Akwatia area of the Birim diamondiferous field, southwest Ghana. African Journal of Science and Technology (AJST) Science and Engineering Series Vol. 8, No. 2, pp. 81 – 91. |
[2] | Attoh, K., Hawkins, D., Bowring, S., Allen, B., (1991). U-Pb zircon ages of gneisses from the Panafrican Dahomeyide Orogen, West Africa. EOS Transactions-American Geophysical Union 72, 229. |
[3] | Attoh, K., Dallmeyer, R.D., and Affaton P., (1997). Chronological nappe assemblage in the Pan African Dahomeyide orogeny, West Africa. Evidence from 40Ar/39Ar mineral ages. Precambrian research, pp 82. |
[4] | Attoh Kodjopa and Nude P.M (2008). Tectonic significance of carbonatite and ultrahigh-pressure rocks in the Pan-African Dahomeyide suture zone, southeastern Ghana, Geological Society, London, Special Publications 2008, v. 297, p. 217-231. |
[5] | Affaton, P., Rahman, M.A., Trompette, R., and Sougy, J., (1991). The Dahomeyide orogeny: Tectonothermal Evolution and Relationships with the Volta Basin. The West African Orogens and Circum-Atlantic Correlatives, DOI: 10.1007/978-3-642-84153-8_6. |
[6] | Berlo et al. (2004). Geochemical precursors to volcanic activity at Mount St. Helens, USA Science, 306, 1167-1169. |
[7] | Brian E. Davies (1971). Trace Metal Content of Soils Affected by Base Metal Mining in the West of England, Oikos Vol. 22, No. 3 (1971), pp. 366-372. |
[8] | Castaing, C., Feybesse, J.L., Thieblemont, D. Triboulet C. and Chevremont, P. (1994). Palaeogeographical Reconstructions of the Pan, African/brasilianoorogen: Closure of an Oceanic Domain or Intracontinental Convergence between Major blocks? Precambrian Research, 67, 327–344. |
[9] | Gill, (1997). Modern analytical geochemistry: an introduction to quantitative chemical analysis techniques for earth, environmental and materials scientists. Longman, 1997 - Science - 329 pages. |
[10] | Hanson, G.N., (1978). The application of trace elements to the petrogenesis of igneous rocks of granitic composition. Earth Planetary Science Letters 38, 26-43. |
[11] | Joreskog (1963). Statistical estimation in factor analysis; A new technique and its foundation, 20. kötet/Selected publications / University of Uppsala, Department of Statistics, ISSN 0502-7489. |
[12] | Morais S., Costa F.G., Pereira M.L., (2012). Heavy Metals and Human Health. Environmental Health - Emerging Issues and Practice DOI: 10.5772/29869. |
[13] | Norman, M, Garcia, M & Pietruszka, A (2005). 'Trace-element distribution coefficients for pyroxenes, plagioclase, and olivine in evolved tholeiites from the 1955 eruption of Kilauea Volcano, Hawaii, and petrogenesis of differentiated rift-zone lavas', American Mineralogist, vol. 90, pp. 888-899. |
[14] | Nude et, al. (2009). Petrology and geochemistry of nepheline syenite and related carbonate-rich rocks in the Pan-African Dahomeyide orogen, southeastern Ghana, West Africa. Journal of African Earth Sciences 55(3):147-157, DOI: 10.1016/j.jafrearsci.2009.03.010. |
[15] | Nude Prosper M. Daniel Kwayisi Naa A. Taki Jacob M.Kutu, Chris Y. Anani, Bruce Banoeng-Yakubo, Daniel K. Asiedu (2015). Petrography and chemical evidence for multi-stage emplacement of western Buem volcanic rocks in the Dahomeyide orogenic belt, southeastern Ghana, West Africa. Journal of African Earth Sciences, vol 112, part A, December 2015. Pp 314-327. |
[16] | Zeng, F., Mao, Y., Cheng, W., Wu, F., & Zhang, G. (2008). Genotypic and environmental variation in chromium, cadmium and lead concentrations in rice. Environmental Pollution, 153(2), 309–314. http://doi.org/10.1016/j.envpol.2007.08.022. |