P. Lakshmi Srinivasa Rao, M. A. Rasheed, S. Zaheer Hasan, P. H. Rao, T. Harinarayana
Petroleum Research Wing, Gujarat Energy Research and Management Institute, Gandhinagar, Gujarat
Correspondence to: P. Lakshmi Srinivasa Rao, Petroleum Research Wing, Gujarat Energy Research and Management Institute, Gandhinagar, Gujarat.
Email: |  |
Copyright © 2014 Scientific & Academic Publishing. All Rights Reserved.
Abstract
Coalbed Methane which offers significant environmental benefits over other fossil fuels has now become an important component of fulfilling energy demands. The production of coalbed methane has become an important industry, providing clean burning fuel. As an estimate the world has 13 trillion metric tons of coal in place. The development cost of CBM is usually lower than the conventional natural gas. The gas produced from coal beds is higher in methane concentration. The geological structure and the properties of coalbed together determine the gas content supplemented by physical and chemical parameters. Ethane and other higher hydrocarbons adsorb strongly than methane, consequently they may not be desorbed at first. Depending upon the maturity, coals have been being characterized as lignite, sub bituminous, bituminous and anthracite. The process of conversion of organic matter to coal generates methane. The amount of methane depends upon the quality and depth-the higher the energy values of coal and deeper the coalbeds-the greater the volume of gas. The reserve estimate and the production forecast differs because of the dual reservoir characteristics-one is the coal matrix, the micropores and the second is open fractures, the macropores-resulting into the reservoir heterogeneity. Geochemical studies like Isotope (biogenic & thermogenic), chromatography (quantity of hydrocarbons) maturation (macerals), deposition environment (bio markers), organic matter type and richness (TOC), proximate and ultimate analysis and mineralogy are used to assess the quality and quantity of hydrocarbons present in the core samples. The objective of this paper is to review the role of geochemistry in CBM exploration with some case studies.
Keywords:
CBM, TOC, Isotope, Biomarker
Cite this paper: P. Lakshmi Srinivasa Rao, M. A. Rasheed, S. Zaheer Hasan, P. H. Rao, T. Harinarayana, Role of Geochemistry in Coalbed Methane - A Review, Geosciences, Vol. 4 No. 2, 2014, pp. 29-32. doi: 10.5923/j.geo.20140402.01.
1. Introduction
A large amount of Carbon Dioxide (CO2) has been generated during the early stage of coalification process and methane is generated in later stages (Rightmire, 1984). The production of methane (Figure 1), adsorption capacity depends on thickness, rank and maceral composition (Levine, 1992). Methane in coal or Coalbed Methane (CBM) can occur as abiogenic, biogenic (microbial) and thermogenic (Scott, 1993). In general, thermogenic gases have been associated with high ranking coal whereas; microbial gases are thought to be produced at early stages of coalification process (Patience, 2003). A detailed study leading to the geological and chemical parameters along with the process of generation of hydrocarbon gas is the essential tool in CBM exploration (A Boruah, 2013). Chemical analyses play an important role in assessment of the quantity, quality and maturation of hydrocarbon gases (adsorbed and gas in place) present in the coal cleats and buts. Proximate and ultimate analyses are the convention laboratory techniques being used in CBM exploration to trace the percentage of ash, moisture, volatile, fixed carbon, hydrogen, oxygen, nitrogen and sulphur in coals (Melegy and Salman, 2009).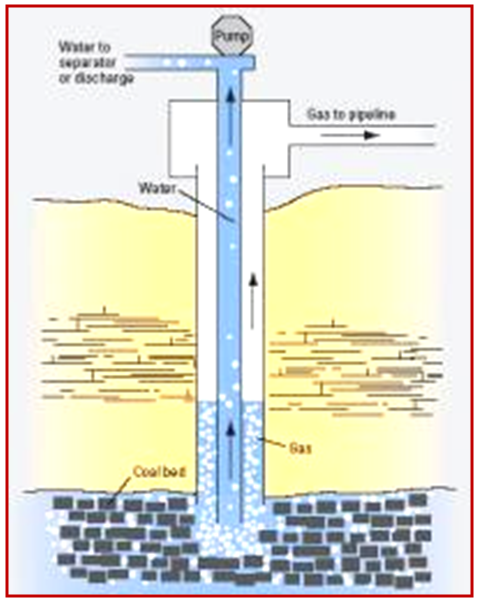 | Figure 1. Coalbed methane recovery diagram (adopted from USGC courtesy: http://serc.carleton.edu/research_education/nativelands/crow/coalbedmethane.html) |
2. Methodological Approach
The mineralogical composition of ashes is determined through X-ray diffraction (XRD) techniques. Proximate analyses are used to find the proportion of ash, moisture, volatile and fixed carbon and ultimate analyses & elemental (C, H, S, N, O) analyser are used for determination of carbon, hydrogen, oxygen, nitrogen and sulphur content. With regard to elemental chemistry, an increase in carbon content and a decrease in oxygen content are the most prominent Characteristic for CBM (Figure 2). Optical Characteristics and elemental composition of coal samples represent the coal ranks from Lignite to Anthracite. Petrographically (Optical), it is easy to distinguish the type of coal on the basis of maceral composition. Maturation indicators like vitrinite, liptinite and inertinite maceral studies are interpreted using the polarising microscope. Liptinite macerals are present upto high volatile A bituminous rank and are absent from medium volatile bituminous coal. At the Anthracite rank, coal is uniform and distinguishing vitrinite from inertinite is impossible. Carbon isotope studies are carried out using the Continuous Flow Isotope Ratio Mass Spectrometer (Gas Bench) to find the source of hydrocarbons, biogenic or thermogenic. These isotopic figure prints are commonly utilized in gas origin classification. Carbon and Hydrogen isotopic values distinguish between thermogenic, biogenic and mixed gas accumulations. These isotopic values can also distinguish between major types of microbial methane generation pathways. Extractable Organic Matter (EOM) is obtained by solvent extraction of powdered sample without acid pre treatment. The EOM is separated into saturates, aromatics and resins plus asphaltenes using standard High Pressure Liquid Chromatography. Separated fractions are used to identify the biomarkers by Gas Chromatograph-Mass Spectrometer (GC-MS) analyses. The depositional environment is identified with selected biomarkers like pristane, phytane, sterane, hopane and norhopanes by using GC-MS. Adsorbed hydrocarbons are quantified with the Gas Chromatograph after desorption of hydrocarbons with acid treatment. 0.5 ml of the gas is injected into the Gas chromatograph equipped with Porapak Q column and flame ionization detector. The carrier gas is Nitrogen with flow rate of 30ml/min and fuel and combustion gas for FID is hydrogen and zero air with flow rates of 30ml/min and 300ml/min respectively. Injector and detector temperatures are maintained at 120℃ and 200℃ respectively. The column temperature program is as follows: initial temperature is 60℃ for 3 min, temperature program rate of 10℃/min, final temperature is 120℃. The calibration of GC is done by external standards with known concentrations of methane, ethane, propane, i-butane, n-butane. The quantitative estimation of light gaseous hydrocarbons constituents in each sample is made using peak areas and the correction for moisture content is applied. The %RSD for C1- C4 is < ± 20%.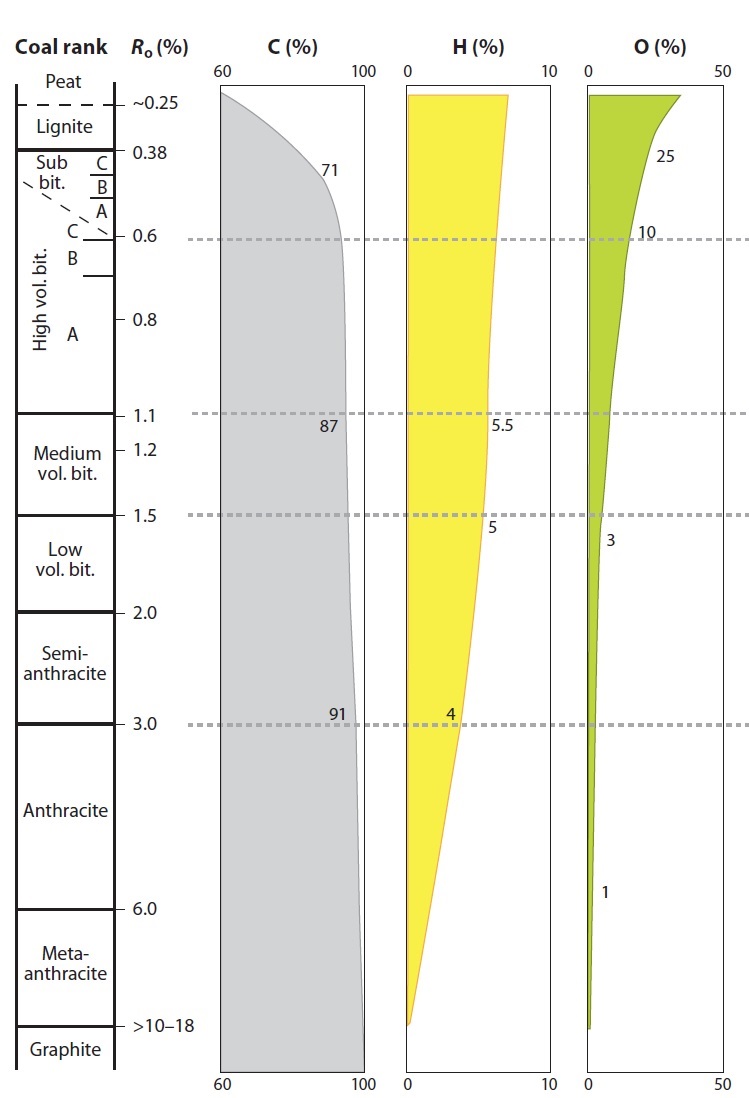 | Figure 2. Changes in selected chemical properties of coal throughout coalification (Modified after Stach et al., 1982) |
3. Discussion
CBM is an eco-friendly natural gas stored in coal seams that has been generated during the process of coalification. CBM occurs within the coal seam mainly as adsorbed gas within the molecular structure of coal, small amount as free gas in fractures and in dissolved form in water. CBM is distinct from conventional gas reservoir, as the methane is stored within the coal by a process called adsorption. Unlike much natural gas from conventional reservoirs, CBM contains very little heavier hydrocarbons such as propane or butane and no natural gas condensate. It consists primarily of methane and may occur as a mixture of methane, carbon dioxide and water which are the products of coal formation.Light gaseous hydrocarbons (methane, ethane and propane) present in the CBM are estimated by using Gas Chromatograph, which gives hydrocarbon index of CBM. For CBM gas hydrocarbon index value should varied from 120 to more than 1000. Wet gas index (C2+) varies from 0.0 to 48.3%. Different genetic types (Abiogenic, Biogenic & Thermogenic) of natural gases have different compositions, mechanisms and isotope values. Istotopic values of Carbon and hydrogen are used to identify the source of coalbed methane. δ 13C1 and δ 13C2 values are considered as an important geochemical indicator for Catagorizing the genetic types of coalbed gas.Biological markers are used to assess the depositional conditions of coal. Sterane, Phytane, pristane and isopreniod distribution are consistent with origin from higher plants. pristane/phytane (Pr/Ph) ratio of coals has high than 1.0 and this ratio explains oxidizing conditions accompanied with deposition of the coals. Significant amounts of benzohopanes and gammacerane are present in some coals and they are reported to occur most commonly in marine environments.Most of the groundwater contains at least six major constituents: calcium, magnesium, sodium, potassium, bicarbonate and sulphate. Water associated with CBM is geochemically modified by the process of sulphate reduction, bicarbonate enrichment and calcium, magnesium depletion to contain primarily sodium and bicarbonate and chloride where influenced by water of marine association. A low sulphate/bicarbonate ratio invariably occurs with CBM.
4. Case Studies
4.1. Coalbed Methane in Hancheng, Ordos Basin
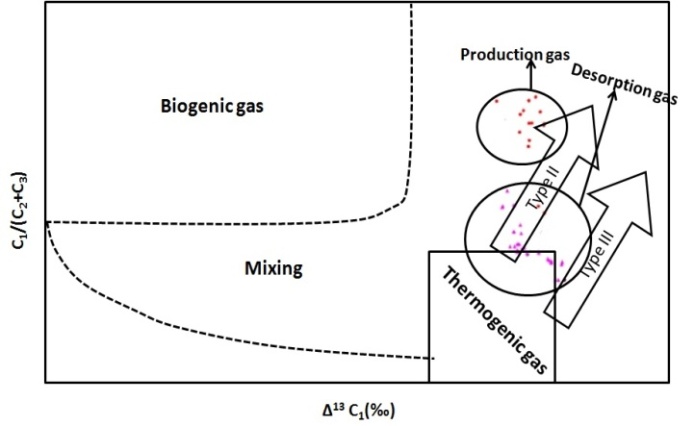 | Figure 3. Relationship between C1/(C2+C3) ratio and the methane (adopted from Xingzhi Ma et. al., 2011) |
Hancheng coalbed methane field is the second largest coalbed methane field in commercial development of China. Xingzhi Ma et al. (2011) collected throughout the Hancheng, Ordos Basin, China. For this study they measured parameters like CH4%, C2H6%, CO2% and δ13C values of methane by using Gas chromatograph and mass spectrometer. The δ13C1 values increase with the depth and Ro values increasing. A positive correlation was established between δ13C1 values and Ro. Carbon isotope values of methane, ethane and propane of a gas, thermally generated from a Type III source rock (δ13Ckerogen= –26.0‰) at a maturity of 1.0 to 1.2Ro%, have been determined with the help of the modeling approach proposed by Berner and Faber (1996). Berner and Faber model is a plot have been drawn in between C1/(C2+C3) vs. δ13C1 (Figure 3) to find the source of hydrocarbons, whether they are biogenic or thermogenic origin. Finally they concluded that coal bed methane is secondary thermal cracking gas with the alteration of isotope fractionation effect during desorption-diffusion process.
4.2. Zonguldak Basin, Western Black Sea Turkey
Coal and associated rock samples from Zonguldak basin, Turkey have been extensively studied by Hosgormez during 2007. Samples were taken from coal seams and adjacent rocks present the Kozlu Formation at eight different points using headspace cans. Coal seams and siltstones within Kozlu formation have total organic content amounts of 7.5 to 85.2%. Rock-Eval pyrolysis data of coals indicate that the organic matter is predominantly vitrinite rich—Type III kerogen. The average δ13C values of kerogen of the samples are between –28.05 and –25.52‰. The δ13Ckerogen values of Mileopera and Çay coal seams range from –28.1 and –26.0 ‰ and display significant 12C enrichment with increasing hydrogen index. The headspace samples consist predominantly of methane with minor amount of C2+ gases; methane ranges between 94.06 to 98.45 % of total HC-gases. The remaining hydrocarbon gases are dominantly ethane and propane percentage of the total C2+ gases is generally lower than 4.26%. The classification diagram suggested by Bernard et al. (1978) is used to investigate the origin of gases. For this purpose, the C1/ (C2+C3) ratio versus the δ13C values of methane compound is taken into consideration and this plot was suggested that the hydrocarbon gases were the mixture of thermogenic and biogenic.
4.3. Coal Seams of Sawang Colliery, Jharkhand, India
The coal seams of Sawang Colliery, East Bokaro Coalfields are bituminous to sub-bituminous in nature and categorized as high gaseous seams (Anil M Pophare, 2008). These seams have identified as potential for (CBM) Coal samples were analysed for maceral (Inertinite, liptinite and vitrinite) percentage and found that maximum pores were confined within the vitrinite group of macerals. Samples in the East Bokaro Coal fields exhibited 0.94 to 1.24 % of vitrinite reflectance. Fuel ratios have been calculated from the proximate and ultimate analyses. Fuel ratio variation shows a restricted range from 1.53 to 1.97. All the coal samples were found to be strongly caking and forming coke buttons. Adsorption isotherm experiments have been carried out for determination of actual gas adsorption capacity of coal seams. This analysis showed that the maximum methane gas adsorbed in the coal is 17 m3/t at maximum pressure of 5.92 MPa and experimental temperature of 30℃.
4.4. Velenje Basin, Slovenia
Coalbed gases in the Velenje basin were shown variable concentrations and stable isotope composition and methane and Carbon dioxide were the major gas components. Due to large variations in concentrations and stable isotope values, it is concluded that the source of methane and carbon dioxide could be thermogenic, biogenic and mixed sources of these two. From the isotope studies (large variations), it was found that most of the gas in the lignite seam is of bacterial origin, indicating CO2 reduction processes mixed with external CO2. A crucial factor determining the formation of coalbed gases in the Velenje basin was bacterial activity. The distribution of coalbed gases (CO2 and methane) was due to various physicochemical reactions of CO2 and methane. Isotopic fractionation due to migration of methane from the lignite seam to the surface is reflected in isotopically light methane in the water saturated layers. Studies of gaseous concentrations and their stable isotopic composition allow a tentative interpretation of the origin of coalbed gas in the Velenje basin. Considering the geochemical results for coalbed gases, it can be concluded that coalbed gas from the Velenje basin is of multiple origin (thermogenic and biogenic).
4.5. Barmer-Sanchor Basin, India
CBM exploration has been carried out in 790 sq. km in Banaskantha district of North Gujarat, India by Oil and Natural Gas Commission (ONGC), India (Chakraborty et. al., 2011). Ten wells (eight core wells and two test wells) were drilled to evaluate the CBM production potential of the block. All the available core samples were subjected to chromatographic and isotopic analysis to know the composition of the desorbed gas and the genetic type of the gas (thermogenic, biogenic and mixed origin). Proximate analysis data showed that these coals are high in moisture (9-23%), very low ash (2-9%), content with with high volatile matter (30-55%) with low calorific value and were assigned to Lignite Rank. Low vitrinite reflectance (0.28 to 0.4) values were observed and indicated very low thermal maturity. The test wells in the study area did not produce coalbed gas even after dewatering for one year. Very little accumulation of gas was observed in the drilled wells. This is due to the low rank and thermal maturity, lack of cleat and fracture, high degree of under saturation, great difference between reservoir pressure and critical desorption pressure, poor permeability, these coals failed to produce coalbed gas.
5. Conclusions
Adsorbed gas analyses are the prime source of information to quantify the hydrocarbons adsorbed on coal surfaces. The detailed study through Carbon and Hydrogen isotopes is found in identifying the source of hydrocarbons. Conventional methods like proximate and ultimate analysis are extensively used to find the potential of coal samples. CBM production depends largely upon the depositional environment of coal seams, which can easily be derived from biomarker studies. The integration of all the analysed geochemical parameters, easily draw inference to evaluate the potential resource of CBM. Maceral studies are used to calculate the maturity of coals, which are used to identify the coal type.
ACKNOWLEDGEMENTS
Authors are grateful to Shri Tapan Ray, MD, GSPC and shri D.J. Pandian, Chairman, GERMI for their continuous encouragement.
References
[1] | Melegy, A and Salman, S. (2009) Petrological and Environmental Geochemical Studies on the Abandoned Maghara Coal Mine, Geolines, v. 22, pp. 44-51. |
[2] | Pophare, A.M., Mendhe, V.A. and Varade, A. (2008) Evaluation of coal bed methane potential of coal seams of Sawang Colliery, Jharkhand, India. Journal of Earth System Sciences, v. 117(2), pp. 121-132. |
[3] | Annapurna Boruah, Kumar B, Rao P H, Harinaryana T. (2013) Integrated development of both conventional and unconventional coal fuels in India, The International Journal of Science & Technology. v.5, 11-17. |
[4] | Bernard, B. B., Brooks, J. M. and Sackett, W. M. (1978) Light hydrocarbons in recent Texas continental shelf and slope sediments. J. Geophys. Res. 83, 4053–4061. |
[5] | Berner, U. and Faber, E. (1996) Empirical carbon isotope/maturity relationships for gases from algal kerogens and terrigenous organic matter, based on dry, open-system pyrolysis, Org. Geochem. 24, 947–955. |
[6] | Chakraborty, A., Tiwari, P.K., Singh, A.K., 2011, Coalbed Methane Exploration in Tertiary Lignite Basin, North Gujarat, India, The 2nd South Asian Geosciences Conference and Exhibition, GEOIndia 2011 (519). |
[7] | Hosgormexz, H, (2007) Origin and secondary alteration of coalbed and adjacent rock gases in the Zonguldak Basin, western Black Sea Turkey, Geochemical Journal, 41, 201-211. |
[8] | Levine, J. R. (1992) Influences of coal composition on coal seam reservoir quality: a review; In: Proc. Int. Symp. “Coal Bed Methane Resource Develop.” Townsville 1 1–28. |
[9] | Patience, R. L. (2003) Where did all the coal gas go? Org. Geochem. 34, 375–387. |
[10] | Rightmire C T 1984 Coal bed Methane Resource of the United States; In: Rightmire C T, Eddy G E and Kirr J N (eds) AAPG Studies in Geology 17 1–13. |
[11] | Stach E, Mackowsky M-Th, Teichmuller M, Taylor GH, Chandra D, Teichmuller R. 1982. Stach’s Textbook of Coal Petrology. Berlin/Stuttgart: Gebruder Borntraeger. 3rd ed. |
[12] | Scott, A. R. (1993) Composition and origin of coalbed gases from selected basins in the United States. Proc. of International Methane Symposium, 207–222. |
[13] | Xingzhi Ma, Yan Song, Shaobo Liu, Feng Hong, Lin Jiang, 2011, Natural Gas geochemistry: Recent developments, Applications, and Technologies, AAPG Hedberg Conference, AAPG Search and Discovery Article #90134©2011. |