Swidiq Mugerwa1, Jolly M. Kabirizi1, William Nanyenya1, Moses Matovu2, Everlyne Komutunga2, Drake N. Mubiru2, Ambrose Agona2, Emmanuel Zziwa3
1National Livestock Resources Research Institute, P. O. Box 96, Tororo, Uganda
2National Agricultural Research Laboratories-Kawanda, P. O. Box 7065, Kampala, Uganda
3Department of Agricultural Production, College of Agricultural and Environmental Sciences, Makerere University, Uganda
Correspondence to: Emmanuel Zziwa, Department of Agricultural Production, College of Agricultural and Environmental Sciences, Makerere University, Uganda.
Email: |  |
Copyright © 2012 Scientific & Academic Publishing. All Rights Reserved.
Abstract
The LIFE-SIM models were used to estimate methane emissions from cows maintained under different feeding strategies. Feeding strategies with adequate protein requirements led to production of more milk and also increased methane emissions. The amount of methane produced per kilogram of milk was lowest in feeding strategy that involved supplementing animals with fresh maize stover and sweet potato vines after feeding Napier grass. The study revealed that there is a great possibility for development of feed management strategies to mitigate methane emissions from cattle through enhancing animal production and reducing the amount of methane produced per unit of milk.
Keywords:
LIFE-SIM, Methane-milk ratio
Cite this paper: Swidiq Mugerwa, Jolly M. Kabirizi, William Nanyenya, Moses Matovu, Everlyne Komutunga, Drake N. Mubiru, Ambrose Agona, Emmanuel Zziwa, Simulation of Methane Emissions from Stall Fed DairyAnimals under Different Dietary Strategies in Uganda, Frontiers in Science, Vol. 3 No. 1, 2013, pp. 1-5. doi: 10.5923/j.fs.20130301.01.
1. Introduction
Uganda’s livestock sector is amidst a major transformation, fueled by high demand for meat, milk and milk products which is likely to double in the near future[1]. The major driving force behind this demand for livestock products is a combination of population growth, urbanization and income growth. The challenge is to enhance animal productivity without adversely affecting the environment and key to this is reducing methane (CH4) emissions from livestock[2]. Methane is the second largest anthropogenic greenhouse gas (GHG), which contributes 14.3% of total anthropogenic GHG emissions estimated in 2004[3]. The concentration of CH4 has increased by about 1,059 ppbv (i.e. from 715 to 1,774 ppbv in 2005) since 1750[3]. Agricultural emissions of CH4 account for about 60% of the total CH4 from anthropogenic sources, of which 25% arises from enteric fermentation in livestock[4]. Methane, a colorless, odorless gas, is produced predominantly in the rumen (87%) and to a small extent (13%) in the large intestines[5]. Rumen CH4 is primarily emitted from the animal by eructation. The conversion of feed material to CH4 in the rumen involves the integrated activities of different microbial species, with the final step carried out by methanogenic bacteria[6]. Primary digestive microorganisms (bacteria, protozoa and fungi) hydrolyze proteins, starch and plant cell wall polymers into amino acids and sugars. These simple products are then fermented to volatile fatty acids (VFA), hydrogen (H2), and CO2 by both primary and secondary digestive microorganisms. Acetate, propionate, and butyrate, which are the major VFA, are then absorbed and utilized by the host animal. The major producers of H2 are the organisms which produce acetic acid in the fermentation pathway[7]. Although H2 is one of the major end products of fermentation by protozoa, fungi and bacteria, it does not accumulate in the rumen. It is used by other bacteria, mainly the methanogens which are present in the mixed microbial ecosystem[7]. CH4 production can be calculated from the stoichiometry of the main VFA formed during fermentation as follows: CH4 = 0.45 acetate – 0.275 propionate + 0.40 butyrate[6]. Thus, the molar percentage of VFA influences the production of CH4. Acetate and butyrate production results in CH4 production, while propionate formation serves as a competitive pathway for H2 use in the rumen. With an increased molar proportion of propionate, the molar proportions of acetate and /or butyrate are reduced resulting into decreased methane production.Since methane emissions in ruminants account for a 2% to12% of gross energy loss of feeds[8], animal nutrition research has in the past focused on finding methods to reduce methane emissions because of its associated inefficiency in energy utilization but not because of its role in global warming[2]. However, because the gas can affect climate directly through its interaction with long-wave infrared energy and indirectly through atmospheric oxidation reactions that produce CO2, a potent greenhouse gas, more recent attention has been given to its potential contribution to climatic change and global warming[8]. Thus, mitigating methane losses from livestock has two important benefits. Firstly, less methane means a lower concentration of greenhouse gases in the atmosphere. Secondly, less methane means increased efficiency of livestock production and increased income for farmers. Technologies for mitigating methane production from ruminants can be broadly grouped into those that increase productivity of the animal (improved nutrition strategies) so that less methane is produced per unit of meat or milk, and those that directly modify the rumen fermentation so that less methane is produced in total[5]. Because technologies that directly modify the rumen fermentation are rarely practiced by smallholder dairy farmers in Uganda, efforts to reduce methane emissions from livestock need to focus on identification and promotion of dietary strategies that mitigate methane losses per unit of product (maintenance, lactation, or growth). The objective of the study was therefore to evaluate the contribution of different feeding strategies on methane emissions per unit of milk produced by smallholder dairy animals in Uganda.
2. Materials and Methods
The study was conducted in Nakabbale village located in Irongo sub-county in Iganga District, Uganda. The district lies astride the Lake Victoria Crescent agro-ecological zone. The zone lies between longitudes 32 and 34o East of the Greenwich Meridian and between latitudes 0 and 1o North of the equator. Rainfall is bimodal in nature and varies between 1000 and 1200 mm annually; the first rainy season beginning in March and ending in June followed by a short dry spell. The next rains start in August and end in November. The contribution of eight feeding strategies on methane emissions from stall-fed dairy cows was evaluated. The feeding strategies (FS) included FS-A composed of Napier grass-NG (70 kg), Sweet potato vines-SPV (20 kg) and Calliandra–CA (10 kg). FS-B composed of Diary meal–Dm (0.5 kg), NG (70 kg), SPV + CA (20 kg) and maize bran-MB (5 kg). FS-C was composed of NG (150 kg), SPV (20 kg), CA+Leucaena-L (3 kg) and MB (4 kg). FS-D consisted of NG (50 kg), SPV (35 kg), CA+L (3 kg) and MB (1 kg). FS-E had SPV+sweet potato peels-SPP (80 kg), CA (1.7 kg), NG (30 kg) and MB (2 kg). FS-F had NG (30 kg), SPV+SPP (80 kg), CA (7 kg) and MB (2 kg). FS-G was composed of NG (90 kg), MS (30 kg) and SPV (50 kg) while FS-H was composed of MB (3 kg), NG (40 kg), SPV (60 kg) and Lablab (15 kg). In all the feeding strategies, Napier grass was offered as the basal feed.For each feeding strategy, three smallholder dairy farmers were purposively selected for estimation of methane emissions as influenced by a particular strategy. This resulted into a total of 24 farmers from whom, measurements were taken. Inter and intra farm variations in animal attributes (such body weight, breed, parity) were reduced by selecting farmers with nearly uniform dairy cows based on a predetermined coefficient of variation among animal traits and condition. The 24 farmers were selected from a sampling frame provided by “Send a Cow”, a Non-Governmental Organization (NGO) seeking to improve livelihoods of the farmers in the study area. The NGO trains livestock farmers on the necessity and procedures of taking proper farm records among other activities. Because of the necessity of adequate records on animal production parameters, management practices and variability in quantity and quality of feeds, the study opted for purposive sampling of farmers who possessed sufficient knowledge and/or expertise on record taking. The feeding strategies of the 24 farmers were monitored for a period of 12 months during which data was collected on the availability in terms quantity and quality of feeds fed to animals. For each feeding strategy undertaken by farmers, the amount of feeds offered to animals was quantified on a daily and monthly basis. The different types of feeds offered to animals were recorded and their nutritive value (crude protein (CP), invitro-digestibility, neutral detergent fiber (NDF), acid detergent fiber (ADF) and dry matter (DM) established according to procedures described by[9] and[10]. The livestock feeding strategies simulation models (LIFE-SIM)[11] was used to simulate milk and methane emissions from animals maintained under different feeding strategies. Information of animal characteristics (such as weight (kg), breed, sex, age, lactation number, lactation length, weight after calving, calf weight); forage characteristics (forage availability (kg/ha), forage digestibility (%), forage protein (%)); and feed supplementation (quantity provided per day (kg), concentration of metabolizable energy (mcal/kg dm), protein level (%), e.t.c.) were fed in into the LIFE-SIM model and the model was commanded to simulate milk and methane emissions. The model simulates milk production based on two equations: | (1) |
Where: PLE: Milk production determined by energy (kg/day); EP: Energy utilized for production (Mcal ME/day); K: Milk production efficiency (0.55–0.65); VEmilk: Energy value of 1 kg of milk (Mcal NE/kg) and ME: Energy for gestation (Mcal ME/kg). | (2) |
Where: VPL: Quantity of protein required to produce a kg of milk (kg); Cas: Percentage of casein in milk (2.5–4.5%); 0.456: Biological value of the microbial protein; TO: Nitrogen Turn Over (value between 1.15 and 1.6).The model computed methane emissions per dry matter intake using the formula below: | (3) |
 | (4) |
3. Results
Table 1 revealed that Napier grass constituted the basal feed in all feeding strategies although the amounts fed varied from farmer to farmer. Farmers were noted to supplement their animals with a variety of feed resources including fresh forages, crop residues and concentrates among others. All feeding strategies practiced by farmers met the necessary energy requirements for maintenance and production. In all feeding strategies, the energy curve was close to the curve for potential milk production implying that the amount of energy availed by all dietary strategies was adequate to achieve the potential milk production of a given cow (Figure 1 and 2). However, Table 2 indicated that majority of the feeding strategies were deficient in protein. In protein deficient feeding strategies, the protein curve was far below the curve for potential production (Figure 2), implying that the amount of protein availed by that particular feeding strategy is inadequate to achieve the potential milk production of a dairy cow. Daily methane emissions varied from one feeding strategy to another and ranged between 300 and 700 liters per animal per day but this was slightly above the range (250-500 liters/animal/day) reported by[3]. Feeding strategies that produced low milk yields (protein deficient strategies) also led to low levels of daily methane emissions. Feeding strategies with adequate protein requirements for maintenance, production and pregnancy led to production of more milk and high daily methane emissions. However, overall methane emissions per unit of milk were reduced in feeding strategies that yielded more milk. FS-A, FS-E, FS-H, FS-B and FS-F were all deficient in protein and were associated with low milk production levels, low daily methane emissions and high methane emissions per unit of milk. The amount of methane produced per kilogram of milk was lowest in feeding strategy that involved supplementing the animal with fresh maize stover and Sweet potato vines in this order, after feeding Napier grass.Table 1. Description of various feeding strategies 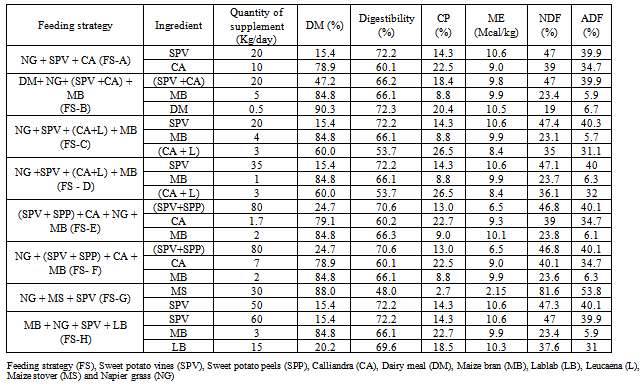 |
| |
|
Table 2. Methane emissions per kg of milk under different feeding strategies 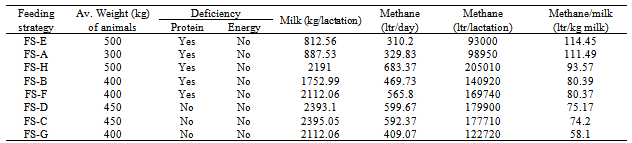 |
| |
|
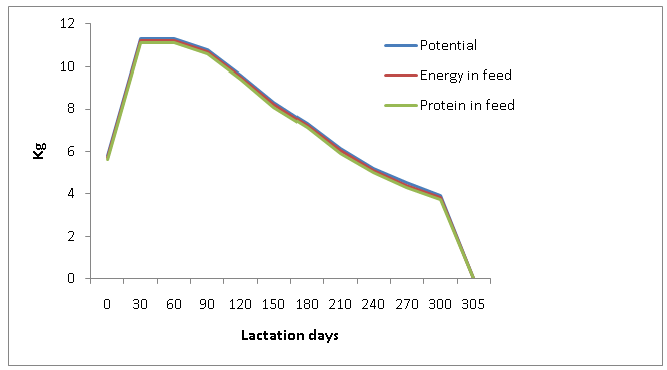 | Figure 1. LIFE-SIM output for FS-C highlighting adequate protein and energy |
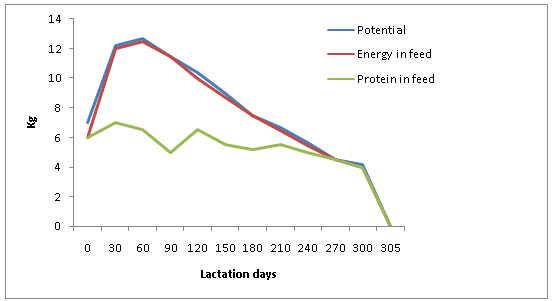 | Figure 2. LIFE-SIM output for FS-D highlighting protein deficiency and presence of adequate energy |
4. Discussion
The fact that all feeding regimes availed adequate levels of energy to meet animal requirements for efficient utilization of carbon meant that ruminal methane emissions were largely influenced by amount of dietary proteins. Feeding strategies deficient in protein were associated with high methane to milk ratio compared to strategies with adequate protein. This relationship was also demonstrated by[12] who noted that supplementation of lactating dairy cows with protein concentrates resulted in a reduction in CH4 emissions of up to 3.5 g of CH4/kg of dry matter intake. As the protein concentration of feed stuffs is increased, both milk production and methane emissions increase because feed stuffs that provide adequate requirements for improved animal performance and production also modify the rumen environment, providing the required nutrients for increased multiplication of rumen microbes including methanogenic bacteria, hence increasing methane emissions. However, increases in beneficial rumen microbes increases rates of feed passage and efficient utilization resulting into more beneficial outputs of producing more milk per unit of methane emitted. This is also consistent with findings of[13] who noted that protein supplementation of low-quality diets increases methane production but decreases methane emissions per unit of product (maintenance, lactation, or growth). Eliminating protein deficiency therefore provides an enormous opportunity for methane mitigation through lowering the volume produced per kilogram of milk produced. Among feeding strategies that met energy and protein requirements of animals (FS-C, FS-D and FS-G), FS-G, that did not involve use of maize bran produced the least amounts of methane. This is due to the fact that provision of highly rumen available carbohydrates at limited amounts causes high fractional losses of methane[5]. Therefore, strategies FS-C and FS-D where maize bran was used in small quantities (4kgs and 1kg/day) produced more methane than FS-G where only plant protein sources were used.
5. Conclusions
The conclusion drawn from this study is that there is a great possibility for development of feed management strategies to mitigate methane emissions from cattle through enhancing animal production and reducing the amount of methane produced per unit of milk. Provided that the energy requirements of the animals are met, utilization of protein rich forage supplements may provide a more feasible method of methane mitigation in resource poor smallholder dairy farming systems where there is a limited use of conventional protein sources.
ACKNOWLEDGEMENTS
This paper presents findings for the project titled “Mitigating the effect of climate change in smallholder dairy animals in Uganda”. The project was funded by the National Agricultural Research Laboratories.
References
[1] | Livestock Development Program, “Livestock restocking and genetic improvement”, Working paper 1, Republic of Uganda, 2002. |
[2] | Amlan Kumar Patra, “Enteric methane mitigation technologies for ruminant livestock: a synthesis of current research and future directions”, Springer Science+Business Media B.V. 2011, Environ Monit Assess, Vol.184, pp.1929–1952, 2012. |
[3] | IPCC, “Summary for Policymakers”. In Solomon, S. D., Qin, M., Manning, Z., Chen, M., Marquis, K. B, Averyt, M. T., & Miller, H. L. (Eds.), Climate change 2007: The physical science basis. Contribution of working group I to the fourth assessment report of the intergovernmental panel on climate change. Cambridge University Press, Cambridge, 2007. |
[4] | Olivier, J. G. J., van Aardenne, J. A., Dentener, F., Ganzeveld, L., and Peters, J. A. H. W., “Recent trends in global greenhouse gas emissions: Regional trends and spatial distribution of key sources”, In A. van Amstel (Ed.) Non-CO2 greenhouse gases (NCGG-4), pp. 325–330, Rotterdam: Millipress, 2005. |
[5] | Torrent, J. and Johnson, D. E. “Methane production in the large intestine of sheep”, In J. F. Aquilera (ed), Energy metabolism of farm animals. EAAP Publication No. 76.CSIC. Pages 391–394 Publishing Service. Granada, Spain, 1994. |
[6] | Moss, A. R., Jouany, J. P. and Newbold, J. “Methane production by ruminants: its contribution to global warming”, Ann. Zootech, Vol. 49, pp. 231–253, 2000. |
[7] | Hegarty, R. and Gerdes, R. Hydrogen production and transfer in the rumen. Rec. Adv. Anim. Nutr. Vol. 12, pp. 37–44, 1998. |
[8] | Johnson, K. A. and Johnson, D. E. “Methane emission from cattle”, Journal of animal Science, Vol. 73, pp. 2483–2492, 1995, |
[9] | Tilley, J. M. A., Terry, R. A., “A two stage technique for in vitro digestion of forage crops”, Grassland Society 18, 104, 1963. |
[10] | Van Soest, P. J. and Robertson, J. B., “Analysis of forage and fibrous foods. A laboratory manual for Animal science 613”, Cornell University, Ithaca, New York, USA. 1985. |
[11] | International Potato Center (CIP), “LIFE-SIM: Livestock feeding strategies simulation models”, Natural resources division, working paper No.2006-1. ISBN 92-9060-267-8, Apartado 1558, Lima 12, Peru. 2006. |
[12] | Grainger, C., Williams, R., Clarke, T., Wright, A.D.G., and Eckard, J. R. “Supplementation with whole cottonseed causes long-term reduction of methane emissions from lactating dairy cows offered a forage and cereal grain diet”, American Dairy Science association, J. Dairy Sci., Vol. 93, pp. 2612–2619, 2010. |
[13] | Birkelo, C. P., Johnson, D. E. and Ward, G. M. “Net energy value of ammoniated wheat straw”. Journal of animal Science, Vol. 63, pp. 20-44, 1986. |