Abdu Zubairu, Sadiq Abba Gana
Department of Chemical Engineering, University of Maiduguri, Borno State, Nigeria
Correspondence to: Abdu Zubairu, Department of Chemical Engineering, University of Maiduguri, Borno State, Nigeria.
Email: |  |
Copyright © 2014 Scientific & Academic Publishing. All Rights Reserved.
Abstract
Waste agricultural biomass (corn cobs) was carbonized in a metal kiln, 90cm in height and 60cm diameter. Four different briquette charcoal grades were produced using locally sourced tapioca starch as binder at concentrations of 6.0, 10.0, 14.0 and 19.0 % w/w. Characterization test was carried out for the charcoal briquettes. The fixed carbon content of the briquette grades is 72.776, 73.958, 78.794, 81.884% w/w respectively. Similarly, the ash content for the briquette grades is 21.38, 20.70, 14.24 and 11.49 % w/w respectively. The bulk density is 425.6, 425.7, 425.0 and 358.3 kg/m3 respectively and the moisture content is 5.88, 5.34, 6.99 and 6.63 % w/w respectively. The properties of the produced briquette charcoal were compared with that of sugarcane bagasse and wood charcoal. The briquette charcoal was found to be a better fuel when compared to both sugarcane bagasse and wood charcoal, having a highest fixed carbon content and highest bulk density. The corn cobs briquettes have lower moisture content compared to sugarcane bagasse however has higher moisture content than wood charcoal. In addition, the sugarcane bagasse and wood charcoal were found to have lower ash content (4.33 % and 9.80% respectively) compared to all the five charcoal grades produced. The briquette charcoal has a mean calorific value of 32.4 MJ/kg which is significantly higher than that of both bagasse at 23.4 MJ/kg and wood charcoal at 8.27MJ/kg.
Keywords:
Biomass, Corn Cobs, Briquette Charcoal, Carbonization, Binder
Cite this paper: Abdu Zubairu, Sadiq Abba Gana, Production and Characterization of Briquette Charcoal by Carbonization of Agro-Waste, Energy and Power, Vol. 4 No. 2, 2014, pp. 41-47. doi: 10.5923/j.ep.20140402.03.
1. Introduction
Sequel to the increasing adverse environmental impacts related to the use of conventional fossil fuels, there is strong interest worldwide in the development of technologies that exploit renewable energy sources; and also, new measures to limit greenhouse gas emissions are continuously sought. Biomass, a naturally abundant domestic energy source is seen as the most promising energy alternative to mitigate greenhouse gas emissions [1]. Waste agricultural biomass is often under- utilized, more also there is rapid increase in volume and types of waste agricultural biomass produced worldwide due to intensive agricultural activities in the wake of population growth and improved living standards. In Nigeria particularly, with a population of over 170 million people, agriculture is the mainstay of the economy contributing more than 40% of the gross domestic product (GDP). In addition, agricultural sector employs more than two-thirds of the total country’s work force and provides livelihood for more than 90% of the rural population [2]. The varying categories of these agricultural wastes is becoming a burgeoning problem as rotten waste agricultural biomass emits methane and leachate while open burning by the farmers to clear the lands (a practice very widely practiced in Nigeria) generates CO2 and other local pollutants. Generally the agricultural wastes in Nigeria could be grouped in to two major classifications; namely the crop residues and the agricultural industrial residues. The major crop residues in Nigeria are the sugarcane trash; straws of millet, corn, wheat, sorghum; maize stalks and cobs; cotton stalks; leaves; roots; barks; branches different types of fibrous materials. The common agricultural industrial residues include timbering residues; oilseeds shells such as groundnut, palm kernel and coconuts; rise husks; cotton wastes; cassava peels; sugarcane bagasse etc.The aim of this work is to use one of the most ubiquitous agro-waste: the corn cobs to produce and characterize briquette charcoal, and to draw comparisons with the properties of a selected biofuel.
2. Theory
2.1. Biomass
Biomass is the third global primary energy source after coal and oil and is set to become an important contributor to the world energy mix [3, 4]. Biomass refers to non-fossil biodegradable organic material from plant, animal and microbial origin. Biomass materials include products, by-products, residues and wastes from agricultural and forestry activities; non-fossil and biodegradable fractions from municipal and industrial wastes. Classical examples are trees, grasses, agricultural crops, agricultural wastes, wood waste and their derivatives, bagasse, municipal solid waste, waste paper, waste from food processing as well as aquatic plants and algae animal wastes [3]. Biomass resources are considered renewable as they are naturally occurring and when properly managed, may be harvested without significant depletion of their sources.As an energy resource, biomass may be used directly as solid fuel for cooking, or converted via a variety of technologies such as pyrolysis, gasification and combustion into liquid or gaseous forms for electricity generation, process heating, steam generation, and mechanical or shaft power applications as well for biofuel production. In Nigeria, the huge volume of agricultural waste generated annually, coupled with the decreasing availability of wood fuel has necessitated concerted effort to look for efficient ways of harnessing these waste for energy generation. Direct combustion of raw agricultural waste as fuel feedstock has some obvious disadvantages including difficulty in controlling the burning rate of the biomass, difficulty in mechanized feeding supply, low heat density, difficulty in stock handling and transportation as well as large storage requirements. Most of these problems are associated with the low bulk density of the agricultural waste. One approach to checkmate these setbacks and efficiently utilize agricultural wastes as fuel is by their densification to produce charcoal briquettes.
2.2. Biomass Charcoal Briquetting
2.2.1. Biomass Charcoal
Charcoal is the solid residual material obtained after carbonisation or slow pyrolysis of biomass in a controlled condition, typically at a temperature range of 450 – 510℃ in the absence of oxygen to remove water and other volatile constituents from the parent biomass often in a closed vessel such as a carbonization kiln or continuously-fed reactor referred to as a retort [4]. Charcoal is porous, solid or amorphous material containing 20 to 25 percent volatile matter, 70 to 75 percent fixed carbon (85 to 98 percent total carbon), 5 percent ash, low sulphur, and with a heating value of about 28 kJ/kg. The quality of charcoal depends on both the biomass source used as a raw material and of the proper application of the carbonisation technology. Charcoal produced from hardwood like beech or oak is heavy and strong. Charcoal made from softwood, on the other hand is soft and light. The bulk density of charcoal does not only depend on the apparent density but also on the size distribution, and is in the range of 180-220 kg/m3. The charcoal yield from biomass is influenced by its lignin, holocellulose, and extractives contents. Biomass species with high lignin contents offer higher charcoal yields, reflecting the fact that lignin preferentially forms char during pyrolysis. Due to thermal cleavage of the sugar units, volatile products are formed from cellulose and hemicelluloses on heating. Char is mainly formed by lignin since it is not readily cleaved to lower molecular fragments. Increase in pyrolysis temperature leads to the volatiles forming a solid residue chemically different from the starting raw material [3].Charcoal produce from agricultural waste biomass are particularly characterised by low bulk density (76 – 180 kg/m3) and high ash content (9.4 – 22.1% on dry basis) which are grave setbacks that hinder their use [5].
2.2.2. Briquettes
A briquette often refers to a block of highly flammable solid material used as fuel to start and maintain a fire. The common types of briquettes are charcoal briquettes and biomass briquettes [6]. The efficacy of the charcoal produced from coal, wood or agricultural biomass in terms of combustion and handling characteristics could be greatly enhanced if the charcoal is converted to briquettes. Briquetting of charcoal is the process of converting the low density pulverised carbonaceous matter from the biomass material to high density and energy concentrated charcoal briquettes often with the aid of a suitable binder material [7]. A process flow for corn cobs briquetting operations is represented in Figure 1.
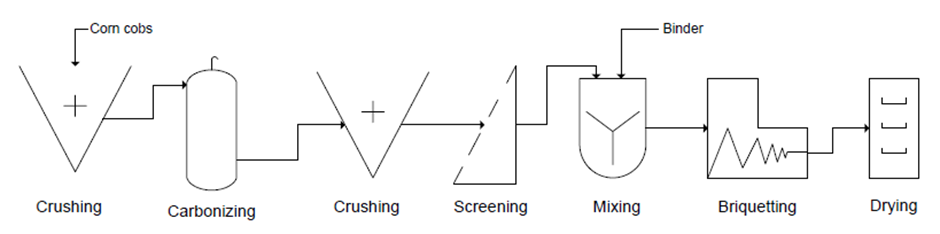 | Figure 1. Flowchart diagram of corn cobs briquetting process |
2.2.3. Binder
The fact that charcoal is a material that is totally devoid of plasticity and hence needs a sticking material or an agglomerating material to enable a briquette to be formed. The process of briquetting therefore, requires the binder to be mixed with the charcoal fines, a press to form the mixture into a cake or briquette which is the passed through a drying oven to cure or set it by drying out the water so that the briquette is strong enough to be used in the same burning apparatus as the normal lump of charcoal. The binder material helps to hold the charcoal particles strongly together in place, such that the briquette is sufficiently hard enough to hold satisfactorily in the fire.It is desirable for the binder material to be combustible, though a non-combustible binder effective at low concentrations can be suitable. Various materials tested for binder application include starch, clay, lime, magnesia, plaster of Paris (POP), tar, pitch from coal distillation, asphalt, sulphite liquor residues, molasses, resins and cement [8]. The best binder for a given application depends on the locality and the kind of biomass being briquetted as well as the purpose for which the briquette is finally intended. However, it has been widely reported that starch if the most effective binder material [6].
3. Materials and Method
3.1. Materials
● Carbonizer (metal kiln)● Corn cobs● Tapioca starch● Briquette press● Desicator● Crucible● Oven● Thermometer● Weighing balance
3.2. Methodology
3.2.1. Biomass Feedstock Preparation
Corn cobs which are traditionally considered as wastes were collected from around University of Maiduguri premises. The corn cobs were sorted and dried to reduce the moisture content of the feedstock to ensure effective carbonization. The dried corn cobs were shredded to small sizes to provide more surface area for the carbonization.
3.2.2. Carbonization
The corn cobs biomass was carbonized using the conventional drum method [7]. The carbonizer is a simple cylindrical design fabricated to provide a means of creating low oxygen environment, it was fabricated using a drum of about 90 cm in height and 60 cm diameter with an opening at the top for loading the corn cobs feedstock. A suitable metal plate was constructed and was used as cover for the top opening of the drum during firing. The schematic diagram of the carbonizer kiln is illustrated in Figure 2.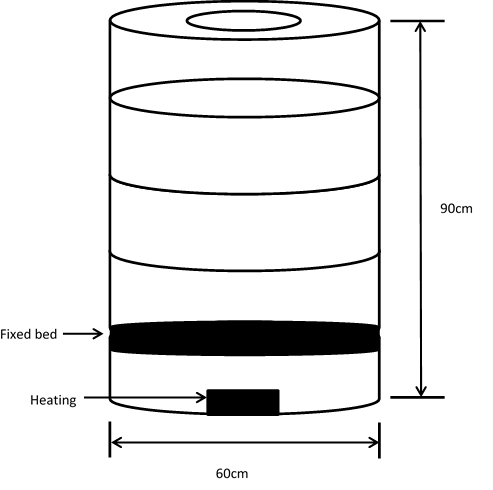 | Figure 2. Schematic representation of the carbonizer kiln |
The biomass (corn cobs) was fed to a reactor at a manageable batch of 5kg. A fire port was provided at the bottom of the drum and was light through the wicks.At the start of the carbonization process the lid was left open for approximately 10 minutes for the volatile gases to escape. The lid was then closed thereafter; properly sealed to prevent air from entering. The biomass material was left to carbonize for 45 - 60 minutes. The fully carbonized material was collected for further processing.
3.2.3. Crushing and Sieving
The carbonized biomass material was grinded to fine particles and sieved using a 200 micron sieve. The sieved pulverised charcoal was measured and divided into four (5) portions of 1kg each.
3.2.4. Binder Preparation
For the purpose of this work a tapioca starch was used as a binder because it is readily available in northern Nigeria and Borno state in particular. In addition tapioca is relatively very cheap compared to some other form of starch sources locally available. Four different binder concentrations were produced by dissolving 60, 100, 140 and 190g of tapioca starch in 0.5 litre of water [9]. At first, the tapioca starch was dissolved in 100 ml of cold water to form a paste, and 400 ml of water was put to boil. The paste was gradually mixed with the boiling water and stirred gently while hot until smooth homogeneous gelatinized starch solution was obtained.
3.2.5. Binder – Charcoal Mixing
For each of the four binder grades solution, 1kg portion of the finely pulverised char powder was added and poured into a mixing bowl while still warm and thoroughly agitated. The carbonized char powder was mixed with the binder in such a way that every particle of the carbonized material was coated with a film of the binder until thick black compound was obtained.
3.2.6. Briquetting and Drying
The starch mixed carbonized material was pressed in a manual cylindrical briquetting moulds fabricated from a recycled steel pipes. The diameter of the mould was 5 cm. The moulded briquettes were placed on clean aluminium trays [9] and were sun dried for 2 days.Four different briquettes grades were therefore produced from the four samples of the starch mixed carbonized char powder based on the binder concentration levels. Figure 3 shows a sample of the dried briquettes.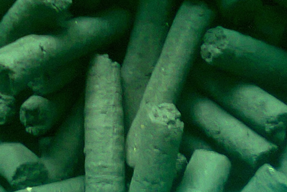 | Figure 3. Dried briquettes from corn cobs biomass |
4. Results and Discussion
4.1. Results
The five different briquette grades with binder concentration of 6.0, 10.0, 14.0 and 19.0 % w/w produced were labelled A, B, C and D respectively. The samples were analysed to tests for moisture content, ash content, fixed carbon and bulk density. The calorific value was calculated from the data obtained for the fixed carbon content. Table 1 shows the summary of the results.Table 1. Properties of Briquette Charcoal 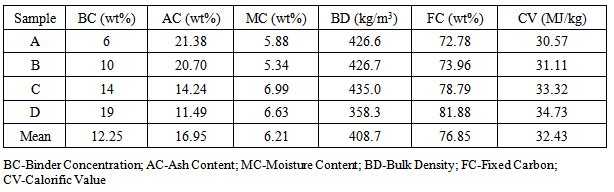 |
| |
|
4.2. Discussions
4.2.1. Briquette Yield
The briquette charcoal yield obtained were 0.87, 1.00, 1.10 and 0.74 kg briquette/kg binder for sample A, B, C and D respectively. The highest yield was recorded at 14% binder concentration as shown in Figure 4.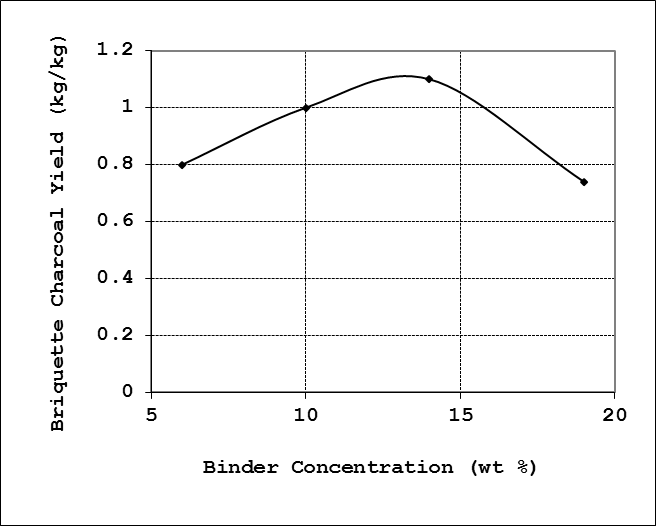 | Figure 4. Effect of binder concentration on briquette yield |
The briquette yield increases only slightly at first with increase binder concentration, and subsequently increases and then falls again with increase in the binder concentration. It could be inferred that initially, at lower binder concentrations, there is not enough binder to coat all carbonised charcoal grains, and thus there is little briquette yield. Increase in the binder concentration such that there is sufficient binder to coat all grains the briquette yield increases significantly. Subsequent addition of binder after grains have been well coated did not bring about any further gain in the quality of the dried charcoal after pressing, and thus the overall yield drops.
4.2.2. Fixed Carbon Content
It was observed that carbon content increases almost monotonically with an increase in binder concentration. The highest carbon content of 81.88% was at 19% binder concentration, whilst the least fixed carbon content of 72.78% was recorded for 6% binder concentration. The profile of the results is shown in Figure 5.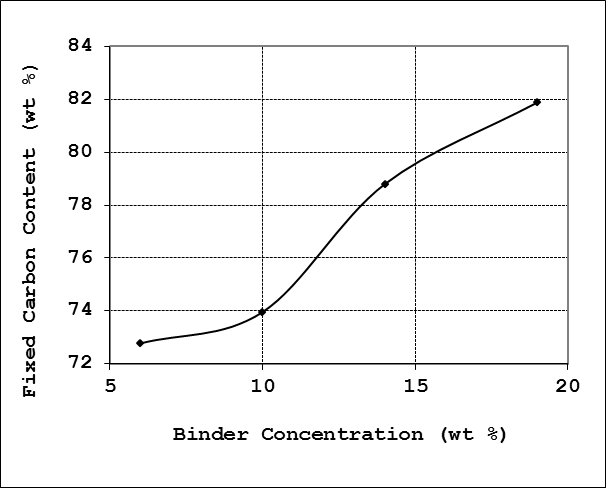 | Figure 5. Effect of binder concentration on fixed carbon content |
The results for the fixed carbon indicates that corn cobs briquettes is suitable for domestic applications according to literature recommendations of fixed carbon content requirements of 80.5%. However, for the binder concentration levels investigated in this work, all the briquette grades exhibit values for the fixed carbon content that fall short of the requirement for industrial applications of fixed carbon of 86.7% [10]. Generally, it was observed that the lower the fixed carbon content; the briquettes tends to be harder, heavier and ignites much easier.
4.2.3. Bulk Density
The bulk density of the briquette charcoal grade was observed to change only fairly slightly by increasing the binder concentration from 6% to 14%, the bulk density increases only by about 2% within this range. However, the bulk density decrease somewhat appreciably on further increasing the binder concentration to 19%. The bulk density profile as function of the binder concentration is shown in Figure 6. Generally, for a given binder concentration the bulk density is a function of the press pressure [8].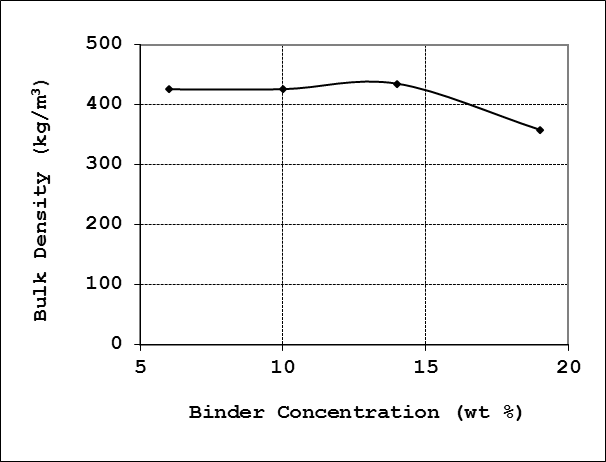 | Figure 6. Effect of binder concentration on bulk density |
The results in this work indicates that the corn cobs briquettes are generally less dense; therefore likely to ignite much easier compared to briquettes from other agricultural biomass sources such as palm kernel [7] and coffee husk [10]. All briquette grades produced in this work are however denser than that produced from bagasse, and have same bulk density range with briquettes produced from a mixture of bagasse and coffee husks [10].
4.2.4. Moisture Content
The profile for the moisture content as function of the binder concentration is shown in Figure 7. The average moisture content for the briquette produced was 6.21%. This agrees well with literature [10] recommendations of 5-10% moisture content for good quality briquettes. Generally, high moisture content leads to briquettes swelling and easy degradation of the briquettes, while briquettes with moisture content of more than 10% often shatters in the furnace in the course of firing.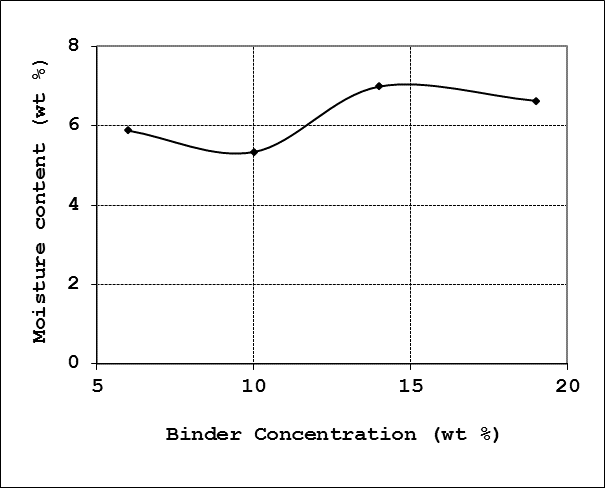 | Figure 7. Effect of binder concentration on moisture content |
4.2.5. Ash Content
The ash content of the briquette charcoal is the amount of ash that remains after the charcoal is burned or incinerated. It was found out that the binder concentration of the charcoal affects the ash content of the briquette charcoal. From the results obtained, it was observed that the ash content of 21.38 % at 6% binder concentration was the maximum. Ash content decreases subsequently as the binder concentration increases to a minimum of 11.49% at 19% binder concentration. The profile for ash content at respective binder concentration is given in Figure 8.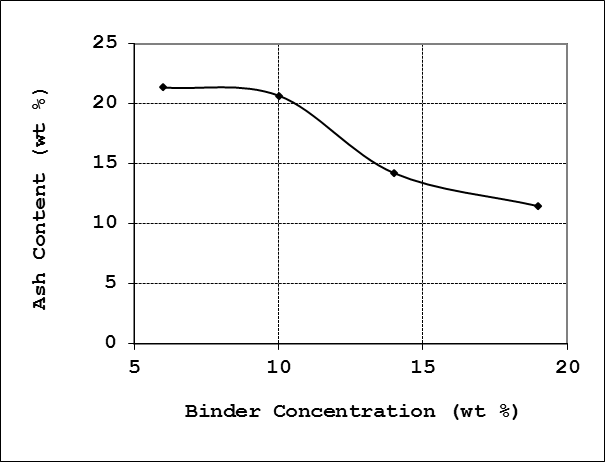 | Figure 8. Effect of binder concentration on ash content |
Literature recommended ash content for good quality briquettes of 3-4% [10]. Briquettes formulated in this work generally exhibit higher ash content than this set limit. On the other hand however, all the briquette grades produced in this work were found to have lower ash content than briquettes produced from several mixtures of wood and saw dust proportions using starch as a binder in another work [12], in which the average ash content of the briquettes produced was more than 20%.
4.2.6. Calorific Value
The calorific value also called the heating value or energy value of the charcoal briquette is the amount of heat liberated per unit mass of the briquette. Calorific values were calculated using the fixed carbon content of the charcoal briquettes according to the method presented in [12]. As with other properties the binder concentration has an effect on the calorific value of the charcoal briquettes. The average heating value for the briquette grades formulated in this work was 32.43 MJ/kg. Generally, the calorific value was observed to increase with increasing binder concentration. The profile for the heating value as function of the binder concentration is presented in Figure 9.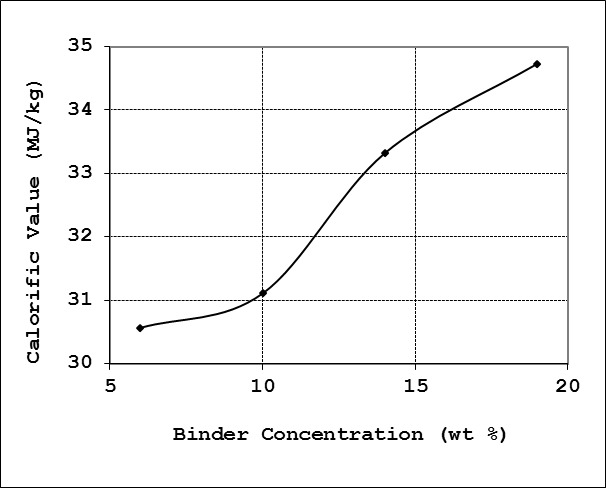 | Figure 9. Effect of binder concentration on calorific value |
All the briquette samples produced in this work were found to have higher heating value than briquette produced from bagasse and coffee husks [12] with respective heating values of 10.4 MJ/kg and 11.4MJ/kg accordingly. Briquettes produced from a mixture of bagasse and coffee husk in the ratio 3:1 also have lower heating value (11.1MJ/kg) than all the briquette grades produced in this work.
4.2.7. Comparison of Briquette Charcoal Properties with that of Sugarcane Bagasse
The average values of the properties of the briquettes charcoal grades produced in this work were compared to both properties of sugarcane bagasse as reported in [4] and that of wood charcoal reported in [10] and are presented in Table 2. The moisture content of the bagasse was found to be around 8.9% which is higher than the average moisture content of the charcoal briquette grades produced in this work at 6.2%. The moisture content of the briquettes is however higher than that of the wood charcoal. The heating value of the sugarcane bagasse will be adversely affected by this higher moisture content. Also it could be seen that the charcoal briquettes had a higher fixed carbon at average value of 76.9% compared to the both bagasse at 66.6% and wood charcoal at 52.6%. Thus both sugarcane bagasse and wood charcoal are less carbonaceous fuels. The ash content of both sugarcane bagasse and wood charcoal are significantly less than that of the corn cobs briquette at 16.95%. This may likely be connected to the tapioca starch used as the binding agent. The briquette charcoal will be easy to transport and store because of its higher bulk density with mean value 408.7 kg/m3 than both sugarcane bagasse and wood charcoal whose bulk densities are 289.0 kg/m3 and 349.0 kg/m3 respectively.. The charcoal briquette is about 40 % higher in calorific value than sugarcane bagasse and nearly 300% higher in calorific value than the wood charcoal.Table 2. Comparison of properties of corn cobs briquette and that of sugarcane bagasse and wood charcoal 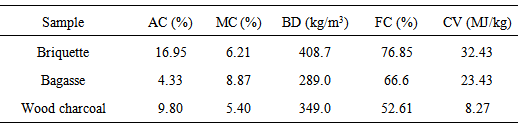 |
| |
|
5. Conclusions
The carbonization of waste corn cobs agricultural biomass was conducted using locally fabricated metal kiln. Four briquettes grades were produced, the physical and combustive properties of the briquette grades were determined. It was concluded that the conversion of waste corn cobs biomass resources into briquette charcoal is an effective means of managing this solid wastes. Furthermore, due to the abundance of waste agricultural biomass resources and as well naturally occurring binder materials which can be sourced locally, carbonized briquetting has the potential to provide employment to the teaming restive youth in northern Nigeria.
References
[1] | Bain, R.L., 2004, An introduction to biomass thermochemical conversion, National Renewable Energy Laboratory, Battelle, p. 17. |
[2] | IFAD Nigeria, 2013, Enabling poor rural people to overcome poverty, International Fund for Agricultural Development Report, Rome, Italy. |
[3] | Demirbas, A., 2010, Biorefineries, Springer Publishing Company, New York, p. 33. |
[4] | Sugumaran. P. and Seshadri, S., 2010, Biomass charcoal briquetting: Technology for alternative energy based income generation in rural areas, Shri AMM Muragappa Chettiar Research Centre, Taramani Chennai, India, p. 1 - 22. |
[5] | Zandersons, J., Kokorevics, A. and Gravitis, J., Studies of bagasse charcoal briquetting, UNU/IAS Working paper No. 58, Latvian State Institute of Wood Chemistry, p. 6 – 18. |
[6] | Forestry Department, Briquetting of Charcoal, FAO Corporate Repository. www.fao.org/docrep. Retrieved 22/05/2014. |
[7] | Ugwu, K. E. and Agbo, K. E., 2011, Briquetting of palm kernel shell, J. Appl. Sci. Environ. Manage. (15) 3 p. 447 – 450. |
[8] | James, E. M., 1908, Binders for coal briquettes, Report of the investigation made at the fuel-testing plant, St. Lous, MO., United States Geological Survey, Bulletein 343, pp. 56. |
[9] | Yael, E., Astrid, H., Sharon, K. S. and Hans, K., 2006, Charcoal dust – A campaign package of the UNESCO global action programme on education for all youth, Booklet 7.10, p. 1 – 16. |
[10] | Pallavi, H. B., Srikantaswamy, S., Kiran, M.B., Vyshnavi, D. R. and Ashwin, C. A., 2013, Briquetting agricultural waste as an energy source, J. of Environmental Science, Computer Science and Engineering & Technology. (2) 1 p. 160 – 172. |
[11] | Ugwu, K. and Agbo, K., 2013, Evaluation of binders in the production of briquettes from empty fruit bunches of Elais Guinensis, Int. J. of Renewable & Sustainable Energy, 2 (4), p. 176 – 179. |
[12] | Emerhi, E. A., 2011, Physical and combustion properties of briquettes produced from saw dust of three hardwood species and different organic binders, Advances in Appl. Sci. Resch. (2) 6 p. 236 – 246. |