Ricardo A. Narváez C.1, Richard Blanchard2, Alfredo Mena3
1National Institute of Energy Efficiency & Renewable Energy (Instituto Nacional de Eficiencia Energética y Energías Renovables, INER), Quito, EC170102, Ecuador
2Centre for Renewable Energy Systems Technology, Loughborough University, Loughborough, Leicestershire, LE11 3TU, United Kingdom
3Energy Researching Corporation in Ecuador (Corporación para la Investigación Energética, CIE), Quito, Pichincha, EC170118, Ecuador
Correspondence to: Ricardo A. Narváez C., National Institute of Energy Efficiency & Renewable Energy (Instituto Nacional de Eficiencia Energética y Energías Renovables, INER), Quito, EC170102, Ecuador.
Email: |  |
Copyright © 2012 Scientific & Academic Publishing. All Rights Reserved.
Abstract
The purpose of this paper is to assess the feasibility of energy production from rice husk in the Province of Los Ríos, located in Ecuador. The overall aim is to determine the most convenient energy generation technological path, considering the amount of resource available, between direct biomass burning for heat production and electricity (on-grid) production through steam turbine usage, pyrolysis gasification and plasma gasification, together with silica gel recovery from the rice husk ash. The decision is made based on the technology state of the different options, the energy production rate and the financial analysis which has a scope of ±30%. This purpose requires developing a basic process design with output streams forecast, cost estimation and financial analysis for each one of the options analysed. A survey identifies three zones in the study area where rice resource is concentrated with the potential to develop and install suitable power plants. The pyrolysis gasification technology presents the most promising solution and a scenario including silica gel recovery increases the profitability of potential projects in all cases.
Keywords:
Agricultural Waste Biomass, Rice Husk, Biomass Energy, Biomass Burning, Pyrolysis Gasification, Plasma Gasification, Bioenergy in Ecuador
Cite this paper: Ricardo A. Narváez C., Richard Blanchard, Alfredo Mena, Use of Rice Crops Waste for Energy Production in Ecuador, Energy and Power, Vol. 3 No. 3, 2013, pp. 27-36. doi: 10.5923/j.ep.20130303.01.
1. Introduction
Significant amounts of agricultural wastes and residues are produced each year. This has been estimated to be 3,758 million tons per year[1] and presents opportunities to use some of this material for energy generation. Rice is a major world food stuff and about 672 million tons of rice grain were produced in 2010[2]. In addition, for every ton of rice grain about 1.5 tons of straw are produced and interestingly about 200 kg of rice husk. Rice husk is the coating of raw rice that is removed in rice milling and is a mixture of cellulose, hemi-cellulose and lignin but has a high ash content of approximately 20%[3]. In addition; rice husk ash is typically 80-90% silica[4] and could represent a co-product to an energy production system that uses rice husk as the fuel feedstock. In this study a critical techno-economic review is performed on energy technologies that can utilize rice husk waste. The aims of this paper were to quantify the availability of rice husk in the study region Los Rios, Ecuador, to compare different technological solutions to producing energy and undertake an economic evaluation of these. Consideration was also given to creating added value by producing coproduct silica from the post thermo conversion ash residue.The first part of the paper describes the rice producing region of Los Rios, by identifying the resource potential and identifying suitable locations for energy production from rice crop waste. Subsequently an analysis of different energy producing technologies was performed to evaluate the efficacy of heat production for drying and three electricity production methods, namely combustion, pyrolysis- gasification and plasma gasification. Following this the potential for rice husk to produce a co-product were investigated. This focused upon the production of silica, the major component of rice husk ash. A financial analysis was then performed on the different electricity generation technologies with and without silica production for comparative analysis. Conclusions are drawn on the most promising systems.
2. Los Rios Rice Production Systems
Los Rios is an administrative region in central Ecuador. A closer analysis of the study region shows access to electricity at around 80% to 86% with higher figures for the southern area[5]. Los Ríos hosts 778 115 inhabitants according to[6], see Figure 1. Three case study rice producing regions were identified in Los Rios and are shown in Figure 1 namely:Northern Zone: San Carlos – QuevedoMiddle zone: VentanasSouthern Zone: Babahoyo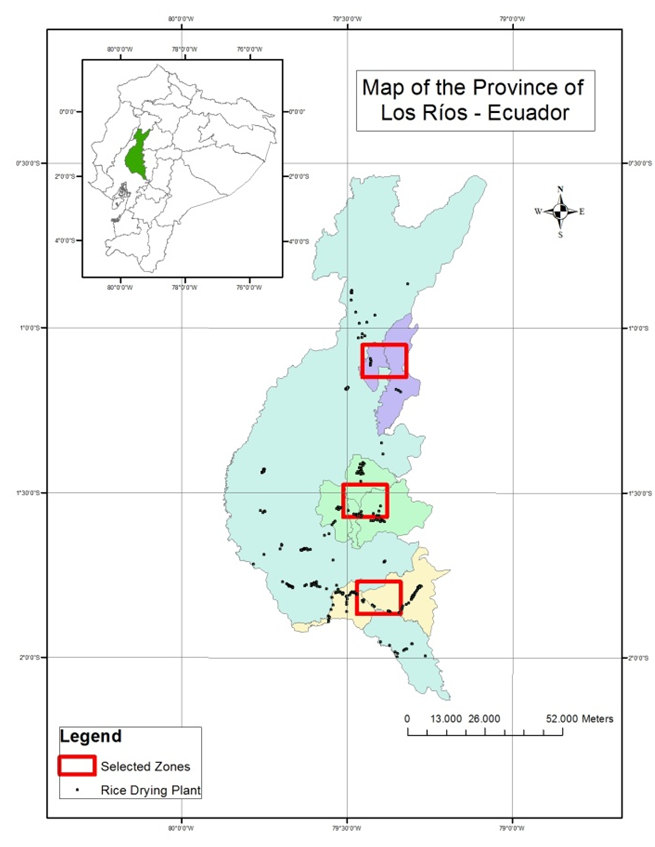 | Figure 1. Zones Summary Map |
2.1. Resource Evaluation
The resource location was the main parameter taken into account for defining appropriate zones for energy production facilities using rice husk. However, additional parameters were investigated using a geographical information system (GIS) to help refine the potential locations as described in the following sections.
2.1.1. Rice Production Indexes
The base information for rice production was taken from[7]. It shows the amount of land utilized for rice cropping activities in any given political division plus the database of rice drying plants within geographical zones inside the region. The geographical analysis identified concentrations of rice production in specific zones of the study region. This same information can then be used to define the annual availability of rice husk per zone. This is made by correlating the rice cropping information with the capacity of all the rice drying plants located in the same political subdivision. In addition, it is necessary to convert this information to obtain rice husk figures due to the base information is given in tons of raw rice or rice cropping hectares per year. In order to correlate the local production with the availability of rice husk, the factor 0.22 kg rice husk/ kg raw rice is considered[8]. The figure 2.85 tons of raw rice per hectare is taken as the production for rice in the study region[7].
2.1.2. Transportation Facilities
This particular parameter considers if power plants are close to the place where the resource is more abundant in order to minimize transportation costs. Considering any potential project has to work with rice husk, a solid fuel, then road transportation is required with the possibility of river transportation The proposed limit is that potential sites must be located close to the main routes (equal or lower than 5 km). This same parameter is useful to filter the database to eliminate rice drying installations that are located far from the main routes shown in the routes GIS database[9] in the region (above 5 km). Like the previous parameter, this one is considered with the purpose of avoiding high transportation costs.
2.1.3. Transmission Lines Availability
The third factor considered was the availability for grid connection in sitting a facility. Again this was principally due to attempting to avoid costly transmission line construction so as to lower investments costs for the project[10].
2.2. Energy Production Facilities Capacity
The facilities capacity was defined based on the information available in the official rice reports provided by the Local Agriculture Authority[7]. In addition, using the rice drying plants database, facilities were identified with available storage structures for rice husk at the same rice drying facilities. This enabled the identification of the zones shown in Figure 1 and establishes the rate of husk fuel availability. Besides this information, a correlation with the harvesting time for the product was required in order to establish the daily load for each facility and the production program during a year.
2.3. Time Line for Resource
The available daily load for energy generation had to be defined and it was related to the electric energy generation regardless the technology to be applied. It was based in the yearly rice production together with the seasonal reports presented in[7]. The production plan included a fully operation plant (7008 working hours per year) and a time considered for maintenance (1752 working hours per year). This maintenance period is coincident with the dry season in the country that incidentally causes electricity shortages. Operating the plants with this strategy would require them to operate during July and August outside the rice harvesting periods.In Ecuador there are two rice harvests annually. The analysis of the harvesting data available shows the harvest in winter is approximately three times bigger than the summer harvest[7]. The time from November to January is considered for maintenance, so the plants are not planned to operate during this time. Figure 2 shows the harvest timeline (or non normalized rice husk production) and the case proposed for rice husk management (normalized). Field observations indicate storage facilities are already available. Therefore, storage matters do seem not to be a matter for concern because structures are already present.The normalization proposed is to process 250 ton per day of rice husk. This assumption is considering 75% of the resource that is collected for energy purposes (68,625 ton rice husk per year). The program requires part of the resource to be stored after winter harvest.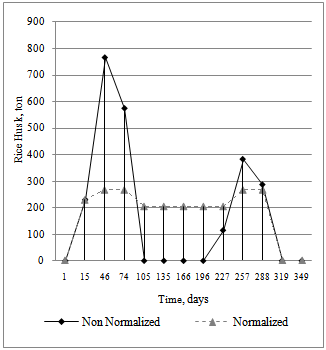 | Figure 2. Time Line for Rice Husk: Production & Potential Consumption |
The site summary locations and capacities are presented as follows in Table 1.Table 1. Hosting Potential Power Plants Zones Summary |
| Zone | Resource expected, ton/ yr | Facility Capacity ton/ day (ton/ h) | Babahoyo | 26,266 | 96 (4.0) | Ventanas | 22,646 | 82 (3.4) | San Carlos – Quevedo | 9,827 | 36 (1.5) | Remaining Areas | 9,886 | - | Total | 68,625 | 214 |
|
|
2.4. Rice Husk Properties
It was planned to collect properties necessary to estimate energy and product streams, certain transportation costs and facilities sizing such as solid transporters and gas movers. The main values are presented in Table 2.Table 2. Rice Husk Properties 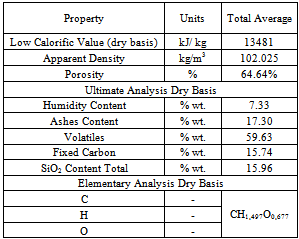 |
| |
|
2.5. Energy Availability Estimation
It is important to mention that rice husk properties were expressed in an intensive scale, which in other words means the figures represent composition and energy content for a single mass unit, in this particular case, a kilogram.The energy estimation for this survey requires assuming the entire rice husk available along the Province of Los Rios has similar figures for energy content and composition.Considering the energy source flow (rice husk) has been already defined on a daily basis, low calorific value is used to obtain an actual raw energy input.Raw energy inputs were calculated for the already established potential power plants zones and are presented below. The following figures are the starting point for the power production facilities designing since the final energy output that is considered as the nominal power capacity are the result of the raw energy input method each technology uses for the conversion from raw energy to electricity.Table 3. Hosting Potential Power Plants Zones Summary |
| Zone | Facility Capacity ton/ day (ton/ h) | Raw Energy Input, MW | Babahoyo | 96 (4.0) | 14.979 | Ventanas | 82 (3.4) | 12.732 | San Carlos – Quevedo | 36 (1.5) | 5.617 | Total | 214 (8.9) | 33.328 |
|
|
3. Heat Production for Rice Drying Plants
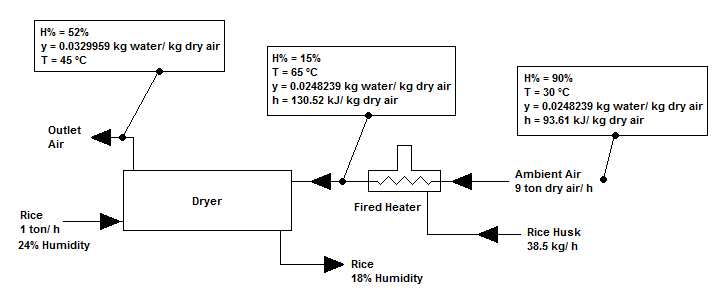 | Figure 3. Rice Drying Process Flow Diagram |
In this point it is proposed to design two fired heater options for small scale rice drying plants (1 ton raw rice/ h and 2 ton raw rice/ h) in order to substitute fossil fuels for rice husk in the zones where the resource is not to be used for electricity purposes. A process flow, Figure 3 is included in order to present the data used for the calculation. The designing was made by using the Wimpress method[11] for furnaces evaluation and Fourier equation derived equation in parallel. Both methods deliver the heat transfer area or heating exchange area (area where the heat is transported to a fluid) and the results are considered valid as long as the figures are similar. Results are presented below:Table 4. Fired Heaters Transfer Areas |
| Capacity,ton raw rice/ h | Transfer Area (Wimpress Method, m2) | Transfer Area (Fourier Method, m2) | 1 | 4.6 | 4.1 | 2 | 8.2 | 8.3 |
|
|
The financial analysis revealed the technology change does not create positive revenues due to the low price paid for fossil fuels in Ecuador even if the rice husk can be obtained with no cost for this scale. The figures for this process are presented in Table 5.Table 5. Results for Heat Production for Rice Drying Purposes Financial Model |
| Capacity | ton raw rice/ h | 1 | 2 | Working Hours | h/ yr | 160 | 300 | LPG for Agriculture | $/ ton raw rice | 1,94 | 1,94 | Savings from Fuel | $/ yr | 310,4 | 1164 | IRR, 5 yr | % | Negative | Negative | NPV, 5yr; 0% debt ratio | $ | -7609.34 | -11803.78 |
|
|
4. Direct Biomass Burning for Electricity Generation
This stage is part of the evaluation of the electricity generation case. The proposal includes a steam cycle with a steam generator, turbine, condenser with cooling tower and recycling pump. The power rating was obtained by applying referential efficiency values for each piece of equipment obtained from their technical brochure. Raw energy is liberated in the steam generator through combustion and transferred to the working fluid which is water in this case. The steam generator is formed by a fired heated boiler therefore energy is transferred from the flames radiation and also from the hot combustion gas stream by convection. This mechanism allows a partial energy loss to the environment with the combustion gases and the high temperature equipment surfaces. An efficiency value of 82% referred to the ASME PTC 4 – 1998 was taken from the equipment technical brochure method for this stage of the process. Since the steam flow rate is required to obtain the steam turbine energy output, an energy balance for the steam generator was developed. The steam carries the energy to a steam turbine which shaft is attached to an asynchronous generator. The steam turbine takes the energy from high enthalpy steam and transforms it to mechanical energy. Steam leaves the turbine at its saturation conditions. This process includes mechanical and heat losses that can are included in the efficiency value of this equipment. The efficiency is obtained from the steam turbine working curves developed by the manufacturer. The efficiency nominal value considered for this particular case (77%) was obtained from the working curve of the steam turbine selected by using the steam flow rate previously calculated. Steam conditions are presented below.Table 6. Steam Turbine Working Conditions |
| Rice Husk Load, ton/ h | Steam Flow, kg/ h | Enthalpy Overheated Steam at 280°C 15 bar, kcal/ kg | Enthalpy Outlet Steam at 1,2 bar X=97%, kcal/ kg | 4.0 | 16726 | 716.7782027 | 622.73053 | 3.4 | 14217 | 1.5 | 6272 |
|
|
The next steam cycle stage required the working fluid condensation since it consumes less energy to be carried to the steam generator when it is on liquid state. Condensation requires a heat exchanger connected to a cooling tower. Steam turns into liquid water inside the heat exchanger since the heat is transferred to a cool water stream that comes from the cooling tower. The cooling tower receives a warm water stream from the heat exchanger and reduces its temperature. This process puts into contact the warm water stream with an air stream from the environment and the result is the water temperatures lows down since the heat reaches the water- air contact area and part of the water is evaporated and dragged to the air stream. The amount of steam that was dragged by the air stream is released to the environment together with the energy removed from the steam cycle working fluid. The condensed water is pumped back to the steam generator in order to close the loop. This process was validated by simulating the processes for each one of the fuel rates previously defined with Aspen HYSYS® and RETScreen®.The power output estimation is presented in Table 7.Table 7. Direct Burning Results |
| Rice Husk Load, ton/ h | Gen Output, kW | Aspen® HYSYSSimulation, kW | RETScreen® Simulation, kW | 4.0 | 1296 | 1385 | 1475 | 3.4 | 1101 | 1178 | 1254 | 1.5 | 486 | 519 | 523 |
|
|
5. Pyrolysis Gasification for Electricity Generation
This option proposes the use of a gasifier, a gas filter bank and a generator set. The power output estimation started from the rice husk elementary analysis. This composition was evaluated with HSC Chemistry® to obtain carbon monoxide and hydrogen as potential combustible gases at a temperature range according to gasification process (around 1000 °C). Thermochemical simulation delivers the equation: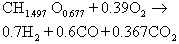 | (1) |
The syngas composition is taken from a Corporación para la Investigación Energética (CIE) pilot scale gasifier and validated by the simulation as shown in Table 8.Table 8. Syngas Composition Comparison |
| Compound | Simulation | CIE Reactor Data[10] | N2 | 44.4% | 50% | H2 | 23.6% | 18% ± 2% | CO2 | 13.3% | 10% ± 3% | CO | 18.7% | 19% ± 3% | CH4 | 0.0% | Above 3% | Total | 100.0% | 100.0% |
|
|
Reactors sizing was developing by taking kinetic data available at Basu et al[12] applied at the continuous stirred tank reactor (CSTR) equation for reactor sizing. The reduction zone was sized with the plug flow reactor (PFR) equation applied to the Bourdon equation. Reactor shape was corrected with the gas flow density considerations described at FAO[13] (under 0.9 m3/ cm2 h) obtaining a conic shape.The value considered for conversion is 75% and 29.62% for the generator set efficiency. The conversion value is the nominal figure of the pilot gasifier found on the manufacturer brochure since it is a commercial model and its capability is already fixed. This figure can be interpreted as the percentage of biomass that is transformed into syngas meanwhile the remaining biomass leaves the reactor without chemical changes. It was required to develop a mass balance of the gasifier considering the initial rice husk input, the nominal conversion efficiency of the equipment, and the chemical reaction (1) previously developed. The purpose of the mass balance was to obtain the syngas stream of each one of the analysed capacities. The energy estimation on this case required the syngas flow rate and the electrical generator manufacturer working curves database. The syngas flow rate was considered as the specific fuel consumption (SFC) and the nominal capacity of the generator set was selected for the model which SFC at full working capacity approximates to the syngas flow rate previously calculated. The findings are presented in Table 9.Table 9. Pyrolysis Estimation Deliverables |
| Fuel Rate, ton rice husk/ h | Gas Flow, Nm3/ h | Power Output, kW | 3.4 | 5510 | 2414 | 4.0 | 6496 | 2845 |
|
|
6. Plasma Gasification for Electricity Generation
Plasma gasification proposal is a generation option similar to the one considered for pyrolysis. The thermochemical simulation was also developed for this stage using a proper temperature range for plasma gasification (9000 °C) with a nitrogen plasma torch. The main difference with the pyrolysis case is the absence of carbon dioxide and a full conversion to hydrogen and carbon monoxide which enhance the gas energy content. Because the lack of data for the given temperature range, the results are based on an extrapolation made by HSC Chemistry® for the thermodynamic data and another one for the kinetic data from Basu[12] in order to size the reactor vessels. Plasma torch energy consumption calculation was based on the nitrogen ionization enthalpy (1.79 x 105 kJ/ kg-mol), a nitrogen/ biomass mass ratio of 0.3 that was considered according to the data of similar solutions developed by High Temperature Technologies®[14], and an efficiency value calculated by the model described by Venkatramani (2002) [15] (77.69%). An additional water inlet was considered in order to maximize the carbon conversion to gas which leads to a higher combustible gases generation. Because of the nature of the process, the conversion is considered close to 100%. Energy estimation was developed under the same method used for the gasification analysis. The results are presented in Table 10.Table 10. Plasma Estimation Deliverables |
| Fuel Rate, ton rice husk/ h | 3.4 | 4.0 | Gas Flow, Nm3/ h | 5703 | 6703 | Total Power Produced, kW | 4700 | 5520 | Power Consumed by Plasma, kW | 2330 | 2370 | Power Delivered, kW | 2740 | 2780 |
|
|
7. Silica Gel Production
The profitability of biomass to energy products can be enhanced where certain biomass materials, such as rice husk, allow the possibility to obtain alternative co-products from the energy production process. Rice husk is a material rich in silicon oxide which is the main component in silica gel. It is estimated it forms around 17% of total rice husk material and about 80% if the post thermal conversion ash residue. Therefore, rice husk ash has the potential of obtaining co-products that increase the profitability of energy projects regardless the chosen technology. Silica gel seems to be an appropriate co-product due to wide range of applications and value.All the thermal conversion methods described in this paper yield rice husk ash as a process stream that can be used in silica gel feedstock production. Two processes have been analysed depending on the conditions the ashes leave the energy generation stage. The temperature range expected for the plasma torch technology case allows the ashes to melt to form a liquid state therefore; the first case utilizes silica recovery a process through crystallization and temperature control. The second approach applies to the ash feedstock from the other processes where the ash does not reach melting point and is available as a solid material. The procedure chosen for the silica gel recovery was described by Rungrodnimitchai et al (2009)[16] and consists of forming an alkaline complex aided by microwave heating. The process is presented in Figure 4.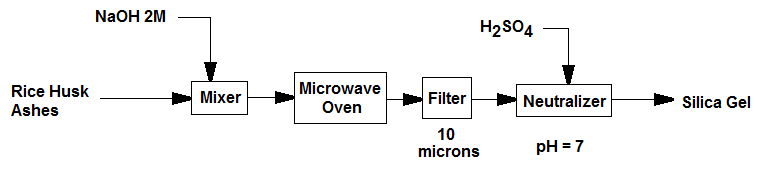 | Figure 4. Silica Gel Recovery through Microwave Heating |
The reaction enforced in the microwave heater is described as: | (2) |
The neutralization together with solution cooling enhances the silica settling process: | (3) |
Silica gel yields were developed based on its content together with the chosen processes capability and feasibility and the findings are shown in Table 11.Table 11. Silica Gel Potential Yields |
| Fuel Rate, ton rice husk/ h | Silica Gel 923 Quality, ton/ yr | Silica Gel Common Quality, ton/ yr | 1,5 | 69 | 1305 | 3,4 | 156 | 2963 | 4.0 | 186 | 3542 |
|
|
8. Financial Analysis
8.1. RE Local Supporting
The local RE development is described in the local legislation. Regulation 004-11 produced by CONELEC (National Electricity Council)[17] is the official document to qualify and award the concerning grants to renewable energy by giving specific tariffs depending on the type of generation. Among others, biomass energy projects below 5 MW are awarded with a tariff of cUSD 11.05/ kWh and cUSD 9.60/ kWh if above, for the Ecuadorian continental territory.
8.2. Economical Assessment
In addition to the technical efficacy of the energy generation systems, it was necessary to analyse the financial aspects of the proposed options for utilizing rice husk as an energy and co-product material Therefore, suitably sized pieces of equipment were defined for each case in order to estimate installation costs and total costs using multiplying factors appropriate for the industry (NREL Biomass Plants, 2005)[18]. This price considers manufacturing (60% of cost) and material (40% of cost). In addition, an extra percentage is considered in order to cover the accessories cost (10% of total) and transportation (10% of total). The pricing model included data obtained by using the software Est$ Pro®. This software estimates equipment; processes and installations costs based on manufacturing data, local salary level and population characteristics. Indirect costs were factored using the recommended ratios found developed by NREL®. The input data for the software required to be organized according to the equipment type. Equipment can be divided into process vessels which require particular designing and construction such as gasifiers and reactors; and auxiliary equipment such as pumps, compressors, electrical motors and electrical generators. Process vessels cost calculation required to size and design the gasifiers previously. It is necessary to determine the volume and the wall thickness of the process vessels in order to estimate the amount of material required for its construction. In the current survey, it was adopted a cost factor expressed as currency unit per kilogram of carbon steel occupied on the construction and installation of a process vessel in the local market conditions. This figure was obtained from the local industry. The weights of the process vessels were multiplied by the mentioned factor owing to estimate their cost. An additional material cost factor was considered on the cases where a material different from carbon steel was required.Auxiliary equipment costs were obtained from local retailers once they were previously sized.Cash flows based on energy production rates, carbon credits and co-products as revenues and operation & maintenance, fuel price and other supplies as costs were carried out using RETScreen® and considered the same general variables value for all cases. The mathematic model below can be used to estimate the cost of any industrial installation therefore it was adopted for this survey. This method allows estimating expenses if the cost detail of a similar project or piece of equipment with a different scale is available[19].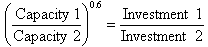 | (4) |
The model was applied for a single piece of equipment at the time so its use followed the restriction mentioned by the authors Williams et al (1947) about similarity between the base case and the scaled one.The remaining part of the financial analysis included the annual cost requirements. It was necessary to include the non-electrical energy selling incomes (silica gel earnings), fix costs and variable costs. The values obtained for internal return rates (IRR) are presented as deliverables in Table 12.Table 12. IRR for Analyzed Options |
| Assessed Options | Fuel Rate, ton rice husk/ h | Direct Burning | Pyrolysis | Plasma | IRR | IRR | IRR | Only Energy Generation | 1.5 | Negative | - | - | 3.4 | Negative | 19.90 | 10.00 | 4 | Negative | 23.70 | 11.80 | Considering Silica Production | 1.5 | 34.10 | - | - | 3.4 | 55.50 | 61.40 | 175.70 | 4 | 58.10 | 66.90 | 193.60 |
|
|
9. Discussion
9.1. General Considerations
It was recognized that the energy project development being considered in this research would require further stages of planning due to the work required to bring a project to fruition. For example, matters such as the specific location within the study zones and their proximity to populations and transport networks. Furthermore, environmental and safety management plans for managing combustible rice husk biomass and product gas would be required along with particular design detail considerations. This clearly means more detailed maps of the zones are necessary for this purpose not only due to the working technical reasons but also because of the terrain conditions or other risk factors like gas stations located nearby. Another fact that may influence the project plan is the biomass gathering itself. The information developed from the authority reports is considered reliable because those are the official figures. Nevertheless it was reasonable to think the rice husk market is going to have the same variability as the local rice market has. Developing a rice husk trading plan for the project requires political guidelines and long term agreements with the same rice producers in order to ensure they sell the biomass material to the project. The aim from the project development point of view was to reduce the trading chain to avoid excessive prices and dumping for external agents. Even if the rice husk is actually being produced; a proper agreement must ensure the product availability for the project. This is because the most sensitive variable is the biomass price. The value of USD 40/ ton (10% of the current price paid for a ton of raw rice) was considered because the harvesting cost can be paid to the producers which is an attractive option for supplying rice husk. Although the findings indicate that silica gel production can yield higher values, it is not desirable to sponsor a rice cropping expansion even greater than the current one. The reason is that this could have a negative effect because of the displacement of other agricultural products and also a lack of other diverse economic activities in the regions. Further stages of the project clearly require a biomass price analysis and strategic development. A general financial factor that can affect the calculations is the price taken for the carbon credits (USD 28/ ton CO2). This price is based on projections made for this purpose[20]. Nevertheless external economical factors are reducing their current price; the authors still maintain the projections are valid so an average price is taken.
9.2. Heat Production Option
The nature of this project makes it easier to develop than the other options described in this paper because of the lower number of agents that are involved in it. This option was technically analysed using two different methods so it can be considered that the required heating area results are reliable although only one of them considers the combustion chamber size on the calculation itself.The volatiles percentage of biomass, in particular rice husk, is relatively high so burning it requires equipment designed and operated in such a way to solve this issue. This consideration was included in the design stage by giving the exhaust gases a higher retention time inside the burn chamber. This fact caused an increase in the chamber pressure which allows for a positive augmentation of the convection heat transfer.The exhaust gases retention time was increased by making the combustion chamber larger in the design and managing the damper opening on the chimney as the operating control factor. The increase in combustion chamber size did not result in the need for a larger heat exchange tube array. The size was obtained from one of the results of the Wimpress method.However, building a larger combustion chamber resulted in increased equipment costs but, had the added advantage of ensuring the exhaust gases are the products of complete combustion. But, even with environmental benefits this option is financially non-competitive. The heat production project itself is a change of technology in part of a drying plant so another option could be adapting the fire heater to rice husk fuel. Nevertheless plants are not standard and would require analysis in each individual case. Adapting an existing furnace would mean assessing if the retention time can be increased by working at a higher pressure and controlling the damper in order to allow the volatile components to have enough time to burn. This path would reduce investment costs and it is possible the financial figures turn positive in some cases.
9.3. Electricity Generation through Direct Burning
The technical analysis on this case delivers reliable results because the chosen technology is mature and the efficiencies for the different pieces of equipment can be obtained from published sources. The simulation prepared for this option showed similar figures which ratifies the consistency of the chosen method (Aspen® HYSYS and RETScreen®). The differences are caused by the different sources of steam properties.Nevertheless the energy output is relatively low considering other analysed options. Even if the equipment is fully operational the necessity of a cooling tower means a considerable amount of energy is going to be wasted. Considering there are more options with higher conversions it was not reasonable to choose this particular technology for a new rice husk installation. Besides this consideration, the financial analysis does not encourage this option as well. The scale of the systems is too small for steam generation due to high installation costs. Thus, the amount of rice husk that can be collected in each of the locations is not enough to provide an economically viable steam power plant.
9.4. Gasification through Pyrolysis for Electricity Generation
The analysis included a review of the gasifier design as well calculating the energy produced using an internal combustion engine coupled to a generator. The gasifier reactor sizing was clearly affected by the chosen kinetic model for the desired reaction. In this case, it was required to obtain a kinetic equation from data available in[12] because of the lack of a proper kinetic model for rice husk gasification. The selection was made by taking into account the most conservative model in order to get the largest reactor volume. This action would ensure the minimum conversion to be obtained was going to be the one fixed for the mass balance in the process. The proposed solution is acceptable for this stage of the project however; a further study should include a kinetic data development.The financial analysis of the project indicates positive revenues and the internal return rate increases as the plant capacity is enlarged. These reasons allow the consideration of gasification, through this method, as a competitive option.
9.5. Gasification through Plasma Torch for Electricity Generation
Plasma torch gasification is a relatively new technology and consequently some of the findings require further review. Even if the energy required for converting the gas into plasma is similar to the one obtained from the data[15], the amount of gas appropriate for treating a quantity of biomass needs to be fixed. This case required a ratio of 0.3 kg N2/ kg of biomass. The only figure found in similar installations has a ratio between 0.35 and 0.72 for biomedical waste.The figure 0.3 kg N2/ kg of biomass is accepted for the current stage. However, it has to be considered that a ratio of 0.72 kg N2/ kg of biomass would mean the process losses the possibility of generating its own energy. The method clearly requires a proper study of this variable as part of the further investigations.In addition, the simulation reveals the power output can be increased by adding water to the reaction chamber. Therefore, it is a good option to increase the profitability of this type of installation due to the low cost of utilizing water in the system.One of the arguments to be discussed is similar to the one mentioned for the gasification reactor design. The plasma reactors are designed for the largest volume plus the requirement to develop an extrapolation of the thermochemical data in simulator HSC Chemistry® due to the working temperature range. Both assumptions rest reliability to the results moreover a more detailed design require to develop this type of information. Even if these considerations have to be taken into account; this stage of the project required the assumption of some facts to perform the calculation.The financial figures for plasma torch gasification are positive. However, other gasification methods deliver better results. Additionally, a process like plasma torch treatment should be used to treat biomass that is more difficult to deal with than rice husk. For example, hazardous waste materials or when material recycling cannot be done in an efficient manner. This would bring more benefits not only from the environmental point of view but also to the economical revenues for plasma technology. The scenario for rice husk proposes paying for the fuel whereas waste material fuel may have a negative cost if landfill taxation is avoided.
9.6. Silica Gel Production from Rice Husk
Whilst the primary product expected from this investigation is electricity; obtaining secondary products such as silica gel seems to increase the profitability of the project options. It is recognized silica gel production is a suitable option due to the high concentration in rice husk. This additional process was analysed under the same criteria used for assessing the energy production to complement that activity.The main contribution made for this development was finding a suitable method for ash treatment where resource consumption was the lowest possible. According to the research, the microwave heating method requires less energy although it still needs some other resources namely sodium hydroxide and sulphuric acid[16].Another method considered was particular for the high temperature plasma torch technology. There are potentially issues in handling substances at high temperatures however, the technology can be considered mature because of its similarity to glass manufacturing In addition, because the liquid state is reached the process does not require additional resources which makes the process of producing silica by this method to be more profitable. The financial figures prove adding silica gel recovery from rice husk ashes increases the profitability in a notable manner. Even the proposed technologies with discouraging economic figures turn attractive by adding this option. It has to be pointed the figures look so promising that a potential payment for using patented processes could be afforded.Indeed, due to the global price of silica gel and the amount that can be obtained from rice husk, silica production is even more profitable than the energy generation itself. This fact identified in the analysis therefore leads to the recommendation of developing silica gel production in conjunction with any energy generation method.
9.7. Validation Considerations
In this research project a number of different energy and resource recovery techniques have been investigated. In order to ascertain the reliability of the results it was necessary to validate the findings against other credible sources. The heat production equipment design is considered as reliable due to both methods producing similar results.The steam cycle calculation was validated by simulating it on Aspen HYSYS® and recalculating it when the financial calculation was made on RETScreen®. The results in the three project case study areas deliver similar figures and so the results are considered as reliable.The gasification through pyrolysis method with the use of an internal combustion engine was validated by including efficiency values from manufacturers and RETScreen® and for each case study area delivered values with acceptable similarity.Plasma torch gasification requires further validation on the efficiency values. The calculation was taken only from reference source[15]. Furthermore, some extrapolations were necessary during the kinetic and thermochemical calculation.Silica gel yields are assumed from the source quoted for the microwave heating method and delivered promising data hence considering an 85% for recovery rate seemed acceptable for the case.
10. Conclusions
The Province of Los Ríos has enough rice husk resource to develop energy projects exclusively utilizing it as a fuel source. The zones where rice husk is more abundant for electricity projects are close to the main populations cores in the region namely , Babahoyo 4 ton/ h, Ventanas 3.4 ton/ h and Quevedo- San Carlos 1.5 ton/ h.The technology review shows in all cases that they are suitable for electricity generation from rice husk. Nevertheless, the financial figures are discouraging when using steam production as part of the process (negative internal return rates).Considering the potential power rates, installation prices and financial results the pyrolysis gasification technology is the most suitable for all the rice husk project locations for electricity generation (19.9% and 23.7% of return rate against 10.0% and 11.8% for plasma torch case; around 2% more power than the pyrolysis option).When considering silica gel recovery, the plasma torch case provides the best return due to the lower supply requirement (return rates above 100% against 61.4% and 66.9% for the pyrolysis gasification case). However, even if the plasma torch technology combined with silica recovery case brings promising figures, this would mean using an exclusive type of biofuel in a technology capable of treating a more complex variety of matter. Besides this statement, it has to be mentioned that plasma torch will require a greater initial investment because of higher installation costs. This means a potential implementation of large-scale plasma torch installations would require a greater project lead time when compared with the installation of a pyrolysis gasification process.Considering this research project was focused on rice husk usage it is suggested to consider pyrolysis gasification with silica recovery as the first option. However, should a broader waste management plan be developed in Ecuador then plasma torch technology could bring options for waste management in a larger scale.
ACKNOWLEDGEMENTS
The authors are grateful to the National Secretary of Superior Education, Science, Technology & Innovation of Ecuador (SENESCYT) for sponsoring the master study related to this project and the development of this work. The Energy Researching Corporation in Ecuador (Corporación para la Investigación Energética, CIE) for assisting during this project and the EUREC Agency, Belgium, Master program.
References
[1] | R. Lal (2005) World crop residues production and implications of its use as a biofuel. Environment International, Vol 31, 4, p575-584. |
[2] | Food and Agricultural Organization. FAO Statistical Yearbook 2012: World Food and Agriculture. Food and Agricultural Organization of the United Nations. Rome 2012. |
[3] | Ahiduzzaman M. Studies and Investigation of Energy and Value-Added Product from Rice Husk. PhD Thesis, Islamic University of Technology, Dhaka, Bangladesh, 2011. |
[4] | Subbukrishna, D.N.; Suresh, K. C.; Paul, P.J.; Dasappa, S. and Rajan, N.K.S. (2007). Precipitated Silica from Rice Husk ash by IPSIT Process. 15th European Biomass Conference & Exhibition, 7-11 May 2007, Berlin, Germany. |
[5] | Consejo Nacional de Electricidad (2011). Cobertura Eléctrica. Available:http://www.conelec.gob.ec/contenido.php?cd=1102&l=1. |
[6] | Instituto Ecuatoriano de Estadísticas y Censos (2011). Ecuador en Cifras. Available:http://www.inec.gob.ec/ecifras/?TB_iframe=true&height=530&width=1100. Last accessed 14th September 2011. |
[7] | Ministry of Agriculture, Animal Husbandry and Fishing. (2011). III CENSO NACIONAL AGROPECUARIO - PRESENTACIÓN. Available:http://www.magap.gob.ec/sigagro/index.php?option=com_wrapper&view=wrapper&Itemid=400. Last accessed 13th July 2011. |
[8] | Peña A., Echeverría M., López O. (2010). CARACTERIZACIÓN ENERGÉTICA DE LA CASCARILLA DE ARROZ PARA SU APLICACIÓN EN LA GENERACIÓN DE ENERGÍA TERMOELÉCTRICA. Quito, Ecuador: EPN, Faculty of Mechanical Engineering. p.32-115. |
[9] | Military Institute of Geography. (2011). Maps of Ecuador. Available:http://www.igm.gob.ec/cms/index.php?option=com_content&task=section&id=11&Itemid=89. Last accessed 13th July 2011. |
[10] | National Electricity Council. (2011). Geoportal Conelec. Available: http://geoportal.conelec.gob.ec/sigelec/index.html. Last accessed 13th July 2011. |
[11] | N. Wimpress, C. F. Braun & Co, Generalized Method Predicts Fired-Heater Performance, Chemical Engineering, May 22, 1978, pp. 95-102. |
[12] | Prabir Basu (2010). Biomass Gasification and Pyrolysis: Practical Design and Theory. Oxford, UK: Elsevier. p.65-116. |
[13] | FAO Forestry Department - Forest Industries Division - Mechanical Wood Products Branch (1986). Wood gas as engine fuel. Rome: Food and Agriculture Organization of the United Nations. p.8-60. |
[14] | High Temperature Technologies Corp. (2009). PLASMA ARC TORCHES FOR PLASMA REACTORS AND PLASMA FURNACES. Available: http://www.httcanada.com/arc.html. Last accessed 5th October 2011. |
[15] | Venkatramani N. Industrial plasma torches and applications. Current Science. August 10 2002, Vol 83, N. 3, p254-262. |
[16] | Supitcha Rungrodnimitchai, Wachira Phokhanusai and Natthapong SUNGKHAHO. (2099). Preparation of Silica Gel from Rice Husk Ash Using Microwave Heating. Journal of Metals, Materials and Minerals. Vol. 19 No. 2 (1), p.45-50. |
[17] | Consejo Nacional de Electricidad (2011). Regulación No. CONELEC–004/ 11. Available:http://www.conelec.gob.ec/pdfs/contenido_pdf_10017.pdf. Last accessed 14th Sep 2011. |
[18] | P. Spath, A. Aden, T. Eggeman, M. Ringer, B. Wallace, and J. Jechura. (2005). Biomass to Hydrogen NREL/TP-510-37408 Production Detailed Design May 2005 and Economics Utilizing the Battelle Columbus Laboratory Indirectly- Heated Gasifier p.19. Available:http://www.nrel.gov/docs/fy05osti/37408.pdf. Last accessed 19th October 2011. |
[19] | Perry R. et. Al., Biblioteca del Ingeniero Químico, Vol 6, México, Mc. Graw Hill, 1986 |
[20] | Thompson Reuters. (2011). CARBON 2011. Available: http://www.pointcarbon.com/polopoly_fs/1.1545244!Carbon%202011_web.pdf. Last accessed 19th October 2011. |