Andile Tonga1, Jennifer Laifa2, Nomagugu Gxaba1, Gloria Miller3, Maria Begonia3, Gregorio Begonia3
1Department of Biological & Environmental Sciences, Walter Sisulu University, Nelson Mandela Campus, Mthatha, South Africa
2Department of Natural Sciences & Environmental Health, Mississippi Valley State University, Itta Bena, Mississippi, USA
3Plant Physiology/Microbiology Laboratory, Department of Biology, College of Science, Engineering, and Technology, Jackson State University, Jackson, Mississippi, USA
Correspondence to: Jennifer Laifa, Department of Natural Sciences & Environmental Health, Mississippi Valley State University, Itta Bena, Mississippi, USA.
Email: |  |
Copyright © 2019 The Author(s). Published by Scientific & Academic Publishing.
This work is licensed under the Creative Commons Attribution International License (CC BY).
http://creativecommons.org/licenses/by/4.0/

Abstract
Copper (Cu) is an essential metal that is needed by humans, animals and plants. The metal has been found to be an irritant to humans. The gastrointestinal effects of copper in humans include nausea, anorexia and diarrhea. Excess copper in plants cause chlorosis, stunted growth, and denaturing of macromolecules. Wild plants are crucial in providing food source for humans. But at times, the wild plants can grow in contaminated soils. Chenopodium album from Chenopodiaceae is a wild leafy vegetable that is consumed in some parts of Eastern Cape, South Africa. It is believed to be rich in proteins, carotenoids and vitamin c. The hypothesis of the study was that Chenopodium album, a leafy wild vegetable consumed by humans in the Eastern Cape, South Africa would uptake and accumulate copper in the harvestable parts. The shoot heights, root lengths, shoot and root biomass and the concentration of copper were measured from C. album growing from soils contaminated with different concentrations of copper. Results revealed that the harvestable parts of C. album have accumulated 160.074 mg per kilogram copper. The results indicate that humans that consume C. album that is growing on copper-contaminated soils are at risk of being negatively affected by copper.
Keywords:
Copper, Chenopodium album, Wild leafy vegetables
Cite this paper: Andile Tonga, Jennifer Laifa, Nomagugu Gxaba, Gloria Miller, Maria Begonia, Gregorio Begonia, An Investigation on the Effects of Copper on Chenopodium album, World Environment, Vol. 9 No. 1, 2019, pp. 13-18. doi: 10.5923/j.env.20190901.02.
1. Introduction
Traditional vegetables are known to be plants that are consumed as vegetables because of their nutritional leafy parts, which include young succulent stems, leaves and their young fruits [1]. Consumption of traditional vegetables is essential because of their nutritional richness and minerals which are useful in maintaining human health [2]. In South Africa more than one hundred different species of plants are consumed as leafy vegetables [1]. These traditional vegetables are the cheapest sources of nutrition that are available to the people mostly in the rural areas of South Africa and also in some African and Asian countries.Although traditional vegetables have these benefits, they can grow in soils that are disturbed including those that are contaminated by heavy metals. An example of a traditional vegetable is Chenopodium album known as a pigweed, imbikicane, and imifuno [3-5]. Chenopodium album is an annual plant belonging to the Chenopodiaceae [3]. In the rural areas of Eastern Cape in South Africa, it is consumed as traditional vegetable and also used for livestock feed, because the C. album leaves are nutritionally rich and contain antioxidants and other important dietary elements [6]. C. album is one of the plant species that can grow in heavy metal-contaminated soils. This poses a great health concern of heavy metal poisoning since the plant is consumed by people as a source of nutrition [7]. Copper is one of the essential elements that are required in small amounts for the activation of enzymes during the synthesis of lignin. However, the ability of C. album to colonize copper-contaminated soils is a major health concern as copper causes damage to the liver [8].C. album is a wild vegetable that can grow in copper-contaminated soils, hence it poses a potential health risk as it is largely consumed by people as a leafy vegetable. There are few studies that have been conducted about C. album and its ability to accumulate copper [9]. This study is important by alerting people about the health risks they are exposed to when consuming the plant growing in copper-contaminated soils. Moreover this provides more information about the plant being used as an accumulator of copper which will help in minimizing copper contamination from the soil. The study was done to close the gap on health risks that result from consumption of C. album growing in copper-contaminated soils. There was a study that was conducted in 2015 at the Ntabankulu area where C. album was found to be one of the wild vegetables consumed by people. The study investigated whether humans are at risk of consuming C. album when found to accumulate copper since copper is one of the heavy metals found from that area.The aim of the study was to investigate the uptake and translocation of copper by C. album. We hypothesized that C. album accumulates copper and translocates it into different organs. To reach the set aim of the study, three objectives were to (a) determine the effects of copper on the growth and development of C. album, (b) identify the parts of the plant that can accumulate copper, and (c) analyze the concentration of copper in C. album.
2. Materials and Methods
The study was conducted in the Department of Biological and Environmental Sciences at Walter Sisulu University, Nelson Mandela Drive Campus, Mthatha Eastern Cape South Africa.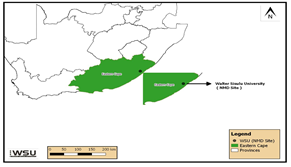 | Figure 1. Study area |
Seeds from C. album were collected from unpolluted areas around Mthatha. The seeds were checked for viability by Dr K. L. Immelman, a curator at Kei Herbarium. A voucher specimen for C. album was deposited at Kei Herbarium at Walter Sisulu University, Nelson Mandela Drive Campus, Mthatha. The soil samples were randomly collected from Walter Sisulu University Botanical garden. The top soil was removed using a garden spade and stored in black plastic bags. The soil was sieved through a 2 mm sieve and stored in plastic bags at room temperature until use. Three hundred grams of the soil was dispensed into 33 different brown paper bags. The soil was then autoclaved for an hour at 121°C for three consecutive days.Copper nitrate in a form of crystals was mixed in the soil to produce copper-contaminated soils. The soil was contaminated with copper nitrate of 10 different concentrations from 10, 20, 30, 40, 50, 60, 70, 80, 90 and 100 ppm. The copper-contaminated soils were dispensed into their appropriate pots and equilibrated for three weeks. Ten seeds of C. album were planted into the pots containing soil with different copper concentrations. The plants were grown for nine weeks to determine the root uptake and subsequent translocation of copper to the shoots. Plants were watered with 40 mL of distilled water. Daily observations were performed to check the effects of copper in plants. After nine weeks, the plants were harvested and washed with distilled water to remove the soil particles from the roots. A 30 cm ruler was used to measure the lengths of roots and shoots from each pot. The shoots and roots were separated, weighed then dried at 75°C for 48 hours. The dried plant materials were weighed then put into 250 mL Erlenmeyer flasks and covered with aluminum foil.Nitric acid-hydrogen peroxide procedure with some modifications was used to extract copper from the shoots and roots of C. album. Fifty mL of 50% aqueous nitric acid was added to a 250 mL Erlenmeyer flask containing the plant samples. The acidified samples were heated to boiling, refluxed for 15 minutes without boiling and then allowed to cool down. Another 10 mL of 50% aqueous nitric acid was added to the samples and was heated for 30 minutes. The heated samples were allowed to cool then completely oxidized in 5 mL concentrated nitric acid. The oxidized solutions were allowed to evaporate to approximately 5 mL without boiling. To initiate the peroxide reaction, 2 mL of deionized distilled water and 3 mL of 30% hydrogen peroxide was added to the concentrated digestate and then heated until effervescence subsided. Another 7 mL of 30% hydrogen peroxide was added in 1 mL aliquots. The digestate was again heated until effervescence subsided. The volume was reduced to 5 mL. After cooling, the final digestate was diluted to about 15 mL with distilled water. The digestate was then filtered through a whatman No.1 filter paper and the final volume was adjusted to 25 mL distilled water. All the samples were analyzed for copper concentrations using an atomic absorption spectroscopy (AAS).The experimental units were arranged in a completely randomized design with three replicates of each treatment. A pot was used as a replicate with five plants in each pot. The harvesting period was nine weeks. Data were analyzed using STATISTICA. One-way ANOVA tests were performed to analyze the effects of copper on height, length and biomass and also copper concentration. The Tukey Honest Significant Difference (HSD) test was used to test significant differences. The statistical criterion for significant difference was set at p>0.05.
3. Results
Effects of copper on growth and developmentC. album grown in copper-contaminated soils showed no symptoms of copper contamination. However, there were few plants that showed signs of copper toxicity such as stunted growth and the yellowing of the leaves. Although the plants were exposed to different copper concentrations for a period of nine weeks, they continued to grow and developed well.Shoot heightsThe results obtained by comparing the shoot heights of C. album (Figure 2, Table 1) showed that there were significant differences between the shoot heights of C. album grown in copper-contaminated soils because p<0.05 at 0.004.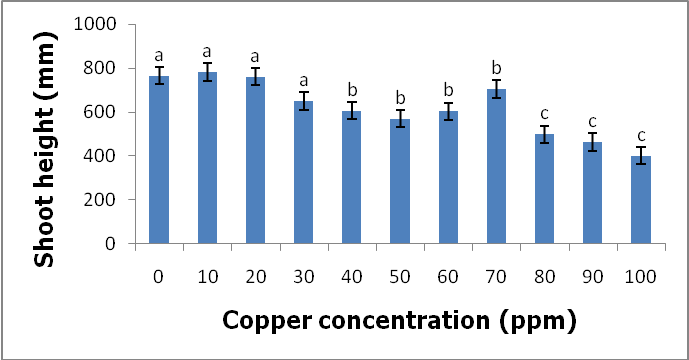 | Figure 2. Effects of copper on the shoot heights of C. album grown for nine weeks. Values and error bars represent means and standard errors of 3 replicates. Means with different letters are significantly different from each other, Tukey HSD, p<0.05 |
Table 1. One-way ANOVA from the C. album shoot heights grown for nine weeks in copper-contaminated soils  |
| |
|
Root lengthsThe results on the root lengths of C. album grown in copper-contaminated soils for nine weeks showed that the root lengths decreased with increasing copper concentration in the soil. The longest root was observed at 0 ppm with a length of 363 mm and the shortest roots were observed at 100 ppm with a length of 125 mm with the exception of 70 ppm (Figure 3).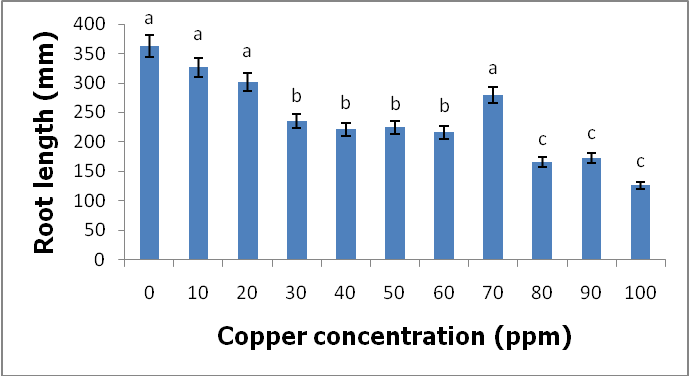 | Figure 3. Effects of copper on the root lengths of C. album grown for nine weeks. Values and error bars represent means and standard errors of 3 replicates. Means with different letters are significantly different from each other, Tukey HSD, p<0.05 |
There was a significant difference between the root lengths of C. album grown in copper-contaminated soils, p<0.05 at 0.033. Therefore, exposing C. album to different concentrations of copper for nine weeks had affected the growth of the roots (Table 2).Table 2. One-way ANOVA from the C. album root lengths grown for nine weeks in copper-contaminated soils  |
| |
|
Shoot dry weightThe results shown in Figure 4 indicated that the shoot dry weight of C. album grown in contaminated soils for nine weeks was negatively affected by the increasing copper concentrations in the soil. The maximum shoot dry weight was recorded at 10 ppm with a mass of 0.0957 g and the minimum shoot dry weight was recorded at 100 ppm with a mass of 0.0263 g.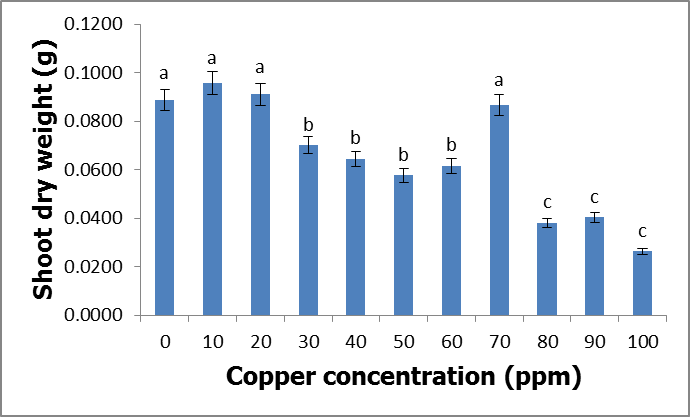 | Figure 4. Effects of copper on the shoot dry weight of C. album after nine weeks. Values and error bars represent means and standard errors of 3 replicates. Means with different letters are significantly different from each other, Tukey HSD, p<0.05 |
There was a significant difference between the shoot dry weights of C. album grown in copper-contaminated soils, p<0.05 at 0.044. Therefore, exposing C. album to different copper concentrations for nine weeks negatively affected the shoot dry weights of the plants (Table 3).Table 3. One-way ANOVA from the shoot dry weights of C. album grown for nine weeks in copper-contaminated soils  |
| |
|
Root dry weightThe results indicated that the root dry weight of C. album was adversely affected by the different copper concentrations in the soil after nine weeks of exposure. The root dry weights of C. album in different concentrations of copper from 10 ppm up to 90 ppm were fluctuating. Therefore, the pattern between the increasing copper concentrations was not clear as the maximum root weight was observed at 70 ppm with a weight of 0.0127 g and the minimum weight at 0.0030 g.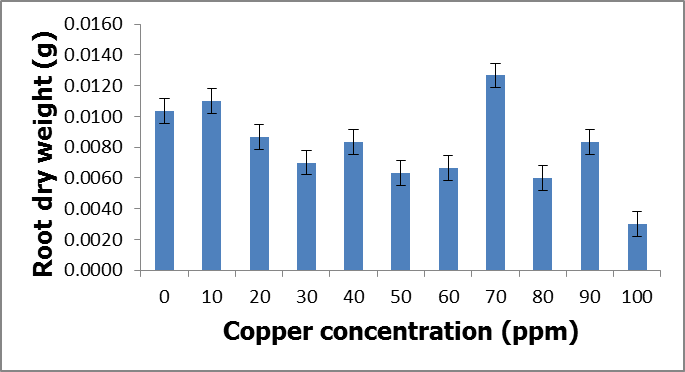 | Figure 5. Effects of copper on the root weight of C. album after nine weeks. Values and error bars represent means and standard errors of 3 replicates. Means are not significantly different from each other, Tukey HSD, p>0.05 |
The results obtained when comparing the root dry weights of C. album exposed in different copper concentrations for nine weeks were not different. Therefore, the root weights were not significantly different from each other because p>0.05 at 0.411 (Table 4).Table 4. One-way ANOVA from the C. album root dry weights grown for nine weeks in copper-contaminated soils  |
| |
|
Copper ConcentrationShoot copper concentrationWhen C. album plants were exposed to different copper concentrations for nine weeks, the plants accumulated copper and then translocated them into their shoots. The amount of copper translocated into the shoots was dependent on the copper concentrations that were available in the soil. The highest amount of copper that was accumulated into the shoots after nine weeks of exposure was observed at 100 ppm with a concentration of 160.074 mg/kg (Figure 6). The lowest amount of copper accumulated in the shoots was observed at 10 ppm with a concentration of 17.231 mg/kg. The accumulation of copper into the shoots of C. album after nine weeks increased from 20 ppm until 100 ppm.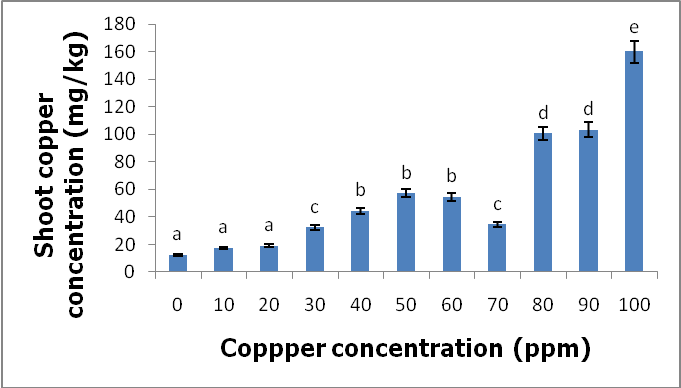 | Figure 6. Copper concentrations from the shoots of C. album grown for nine weeks. Values and error bars represent means and standard errors of 3 replicates. Means with different letters are significantly different from each other, Tukey HSD, p<0.05 |
There were significant differences between the amounts of copper accumulated in the shoots of C. album grown in copper-contaminated soils, p<0.05 at 0.000 (Table 5).Table 5. One-way ANOVA from the shoot copper concentrations of C. album grown for nine weeks in copper-contaminated soils  |
| |
|
Root copper concentrationThe roots of C. album accumulated copper from the soil after nine weeks of exposure to copper contaminated soils. The amount of accumulation by the roots of C. album varied with the different copper concentrations that were available in the soil. However, the amounts of copper that were accumulated by the roots in a period of nine weeks were fluctuating with the increasing copper concentrations in the soil. The minimum accumulated copper concentration was observed at 10 ppm with a concentration of 434.513 mg/kg (Figure 7) and the maximum accumulated copper was observed at 100 ppm with a concentration of 2175.833 mg/kg. The pattern for the metal accumulation in the roots was somehow not clear.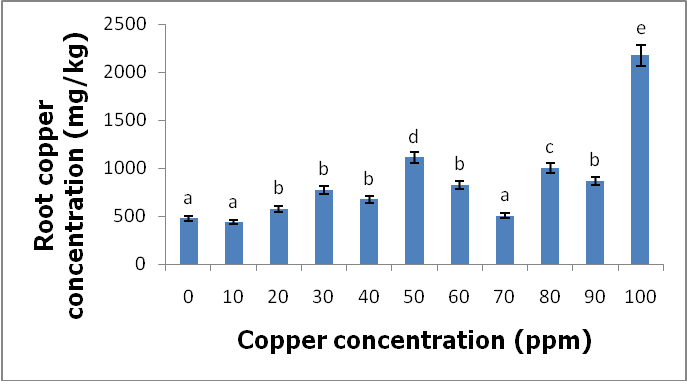 | Figure 7. Copper concentrations from the roots of C. album grown for nine weeks. Values and error bars represent the standard errors of 3 replicates. Means with different letters are significantly different from each other, Tukey HSD, p<0.05 |
There were significant differences between the amount of copper accumulated in the roots of C. album grown in copper-contaminated soils, p<0.05 at 0.000 (Table 6).Table 6. One-way ANOVA from the roots copper concentrations of C. album grown for nine weeks in copper-contaminated soils  |
| |
|
4. Discussion
Heavy metals occur naturally in the soil in different concentrations. These heavy metals are important in the soil as they play important roles in the plants’ growth and development when available at minimum levels in the soils. The release of these heavy metals in the environment due to human activities such as industrial waste, bactericides and fungicides lead to the increase of heavy metal concentrations in the soil. Their increase has a negative impact on the soil, agriculture and human health. Copper is an important micronutrient that is required by plants in small amount for their growth and development. When copper is available in excess amounts in the soil, it induces stress and causes damage in plants. Symptoms of excess copper in the plants include growth retardation due to the inhibition of roots elongation and leaf chlorosis [10].When plants are exposed to heavy metal-contaminated soils they tend to accumulate the elements from the soil regardless of the concentrations that are available in the soil. When the heavy metals are available at higher concentrations in the soil, they affect the fertility of the soil reducing the crop yields. Wild vegetables on the other hand are consumed as an alternative source of nutrition in the rural areas of South Africa and other African countries. In Europe some of the traditional vegetables are cultivated and used to produce flour [3].Wild vegetables grow in disturbed environments to some extent that they can grow in heavy metal-contaminated soils. This ability of thriving in heavy metal-contaminated soils raises health concerns, because plants that are exposed to heavy metal-contaminated soils tend to accumulate the elements from the soil [11]. Consumption of food contaminated with heavy metals such as copper poses serious health risks such as liver damage [8].In the present study, copper had no negative effects on the growth and development of the plants since there were no symptoms of copper toxicity such as stunted growth and leaf chlorosis. The present study is in contrast with the study by [12] which showed that copper had visible symptoms of morphological alterations such as the shortening of the stem and the chlorotic spots on the leaves.In this study the different copper concentrations were inversely proportional with the shoot heights. As the copper concentration increased from 10 ppm up to 100 ppm, the shoot heights of the C. album started to slightly decrease to the point whereby the shortest shoot height was recorded at 100 ppm. This study is in contrast with the study by [13] where the different copper concentrations had no effects on the shoots heights of the plants. From the same study by [13] the shoots of plants grown in different copper concentrations were more or less the same as the control plants. Similar outcomes were also recorded in the root lengths even though there were visible symptoms of copper toxicity such as the black tips and yellow appearance.In the present study, the shoot phytomass of C. album decreased accordingly with increasing copper concentrations in the soil. This study is in agreement with that of [14] who pointed out that shoot phytomass decreased with increasing concentration of copper in the soil, even though minimal amounts of copper were translocated from the roots to the shoots. Copper had no effect on the root phytomass of C. album. This study is in contrast with the study by [15] which showed that root phytomass of plants grown in different copper concentrations decreased with increasing copper concentrations due to the inhibition of root elongation and growth of root hairs by copper toxicity.In the present study copper accumulation in the roots and the shoots significantly increased with the increasing copper concentrations in the soil. These results are in agreement with the study by [11] which showed that the accumulation of copper in the roots and shoots increased with the increasing copper concentrations in the soil. The results for this study showed that the roots of the C. album accumulated more copper than the shoots.Wild vegetables are a good source of nutrition and can grow in heavy metal-contaminated soils. This is in agreement with the present study where C. album was grown in copper-contaminated soils and developed well without any visible symptoms of copper toxicity. The study revealed that C. album can accumulate copper. The accumulation of copper by C. album increased with increasing copper concentrations in the soil. The study also revealed that after root uptake, copper was translocated to the shoots.
5. Conclusions
C. album growing in copper-contaminated soils for nine weeks showed no visible symptoms of copper toxicity. However, the shoot heights and root lengths decreased with increasing copper concentrations. Copper had an effect on the shoot phytomass of C. album. More specifically, as the copper concentrations increased, the shoot phytomass slightly decreased and the lowest mass recorded at 100 ppm. However, root weight was not affected by different copper concentrations. Accumulation of copper by C. album increased with increasing copper concentrations in the soil. The amount of copper accumulated in the roots was higher than in the shoots. The highest amount accumulated in the roots was 2175.833 mg/kg and the highest amount accumulated in the shoots was 160.074 mg/kg. The accumulated copper by plants was higher than the accepted amount of copper accumulation by plants and vegetables which is 10 mg/kg of copper. Copper is an essential element that is crucial to both plants and animals when available in small amounts. Consumption of wild vegetables growing in copper-contaminated soils may cause serious health problems. Soil contamination due to heavy metals is a major problem in agriculture and it is a major concern for public health because when these heavy metals are available in toxic levels they affect the growth and development of the plants. They also cause serious health problems when consumed.
References
[1] | Van Rensburg, W.J., Van Averbeke, W., Slabbert, R., Faber, M., Van Jaarsveld, P., Van Heerden, I., Wenhold, F. and Oelofse, A., 2007. African leafy vegetables in South Africa. Water SA, 33 (3). |
[2] | Kumar, A.C.K., Sree, M.D., Joshna, A., Lakshmi, S.M. and Kumar, D.S., 2013. A review on South Indian edible leafy vegetables. Journal of Global Trends in Pharmaceutical Sciences, 4 (4), 1248-1256. |
[3] | Jan, R., Saxena, D.C. and Singh, S., 2016. Pasting, thermal, morphological, rheological and structural characteristics of Chenopodium (Chenopodium album) starch. LWT-Food Science and Technology 66, 267-274. |
[4] | Gqaza, B.M., Njume, C., Goduka, N.I. and George, G., 2013. Nutritional assessment of Chenopodium album L. (Imbikicane) young shoots and mature plant-leaves consumed in the Eastern Cape Province of South Africa. International Proceedings of Chemical, Biological and Environmental Engineering, 53 (19), 97-102. |
[5] | Njume, C., Goduka, N.I. and George, G., 2014. Indigenous leafy vegetables (imifino, morogo, muhuro) in South Africa: A rich and unexplored source of nutrients and antioxidants. African Journal of Biotechnology, 13 (19). |
[6] | Bhargava, A., Shukla, S., Srivastava, J., Singh, N. and Ohri, D., 2008. Genetic diversity for mineral accumulation in the foliage of Chenopodium spp. Scientia Horticulturae, 118 (4), 338-346. |
[7] | Girdhar, M., Sharma, N.R., Rehman, H., Kumar, A. and Mohan, A., 2014. Comparative assessment for hyperaccumulatory and phytoremediation capability of three wild weeds. 3 Biotech, 4 (6), 579-589. |
[8] | Gebrekidan, A., Weldegebriel, Y., Hadera, A. and Van der Bruggen, B., 2013. Toxicological assessment of heavy metals accumulated in vegetables and fruits grown in Ginfel River near Sheba Tannery, Tigray, Northern Ethiopia. Ecotoxicology and Environmental Safety, 95, 171-178. |
[9] | Maroyi, A., 2013. Use of weeds as traditional vegetables in Shurugwi District, Zimbabwe. Journal of Ethnobiology and Ethnomedicine, 9 (1), 60. |
[10] | Raju, D., Hazra, S. and Mehta, U.J., 2013. Natural accumulation of copper and distribution of metals in plants growing in copper mining area, Rajasthan, India. Bioremediation, Biodiversity and Bioavailability, 7 (1), 54-60. |
[11] | Fu, L., Chen, C., Wang, B., Zhou, X., Li, S., Guo, P., Shen, Z., Wang, G. and Chen, Y., 2015. Differences in copper absorption and accumulation between copper-exclusion and copper-enrichment plants: a comparison of structure and physiological responses. PloS one, 10 (7). |
[12] | Panou-Filotheou, H., Bosabalidis, A.M. and Karataglis, S., 2001. Effects of copper toxicity on leaves of oregano (Origanum vulgare subsp. hirtum). Annals of Botany, 88 (2), 207-214. |
[13] | Lin, J., Jiang, W. and Liu, D., 2003. Accumulation of copper by roots, hypocotyls, cotyledons and leaves of sunflower (Helianthus annuus L.). Bioresource Technology, 86 (2), 151-155. |
[14] | Guo, X.Y., Zuo, Y.B., Wang, B.R., Li, J.M. and Ma, Y.B., 2010. Toxicity and accumulation of copper and nickel in maize plants cropped on calcareous and acidic field soils. Plant and Soil, 333 (1-2), 365-373. |
[15] | Sheldon, A. and Menzies, N.W., 2004. The effect of copper toxicity on the growth and morphology of Rhodes grass (Chloris gayana) in solution culture. In 3rd Australian New Zealand Soils Conference, 5-9 December 2004, University of Sydney, Australia. |