Umair Rahim, Baskaran Kasi
Department of Electrical and Electronics Engineering Infrastructure University Kuala Lumpur, Kajang, Malaysia
Correspondence to: Umair Rahim, Department of Electrical and Electronics Engineering Infrastructure University Kuala Lumpur, Kajang, Malaysia.
Email: |  |
Copyright © 2016 Scientific & Academic Publishing. All Rights Reserved.
This work is licensed under the Creative Commons Attribution International License (CC BY).
http://creativecommons.org/licenses/by/4.0/

Abstract
The Ultra-Wideband (UWB) communication systems has emerged as a technology that offers a great promise to fulfil the growing demands for short-ranged, high-speed wireless communications. Hence, this paper proposes the use of a compact patch bevel shaped microstrip ultra-wideband antenna design which has the capability to operate between a 3.1 GHz to a 10.6 GHz frequency range. An in depth investigation is conducted on the proposed antenna type whereby the partial ground plane and radiating patch, with parameters in frequency domain and field radiation patterns is examined. Apart from that, the measured S11 features that display the aptitude of an antenna as effective radiating elements, along with time domain analysis are also probed into. As a concluding outcome, the simulation results demonstrated a judicious agreement with the measurement results, signalling a good ultra-wideband linear transmission performance being achieved in the time domain.
Keywords:
Ultra-wide band (UWB), FR4, Microstrip, Patch antenna, Frequency domain, Time domain
Cite this paper: Umair Rahim, Baskaran Kasi, A Compact Ultra-Wideband Antenna with Time Domain Analysis, Electrical and Electronic Engineering, Vol. 6 No. 4, 2016, pp. 68-73. doi: 10.5923/j.eee.20160604.03.
1. Introduction
An antenna is defined as electrical device which converts electric energy into radio waves, and vice versa. Which is very useful with a radio transmitter or radio receiver. The antenna becomes an important part of electrical devices in wireless communication system in the late1888; Heinrich Hertz (1857-1894) were first who explained the radio waves [1].The Ultra-wideband (UWB) communication systems has emerged as a technology that offers great promise to fulfil the growing demand for short-range high-speed wireless communications [2]. Since the release of the UWB frequencies by the Federal Communication Commission (FCC) [3] ranging from 3.1GHz to 10.6GHz in 2002, UWB communications systems have received much attention to develop UWB antennas for emerging applications. UWB has promised to offer higher data rates, saturation of the frequency spectrum, low power and wide bandwidth are some of the reasons that increased the interest in the UWB during the past few years [4-7]. UWB, in comparison with narrowband systems, the antennas have greater impact in UWB system because of the optimized antennas due to its extremely wide bandwidth, the antennas transmit pulsed signals not continuous wave signals. Standard parameters in the frequency domain such as return loss, gain, radiation efficiency, etc. have been defined for narrowband antennas. Therefore analyzing UWB antennas only in the frequency domain is not enough as pulse distortion is an important parameter that should be controlled. Thus it is required to study the antenna characteristics in the time domain as well [8].In this paper, a simple and compact bevel shaped microstrip ultra-wideband antenna for UWB application is proposed. For bandwidth enhancement four different techniques are used such as partial ground, partial radiator and beveling technique on both ground and radiating patch. The return loss –10 dB bandwidth of the antenna covers 2.8 to 11 GHz which satisfies the UWB system requirement. The outline of this paper is as follow. Section 2 describes the proposed antenna geometry and configuration. Simulation and simulated results are presented in Section 3 and the conclusions are summarized in Section 4.
2. Antenna Design and Configuration
The proposed compact UWB antenna configuration and its geometrical parameters are shown in Figure 1 and table 1 respectively. This proposed antenna design consists of partial radiating patch fed by a microstrip line printed on a partial ground and FR4 substrate with a total area of 40mm×30mm, thickness of 1.6 mm, permittivity of 4.4. The microstrip feed line is designed to match a 50 Ω characteristic impedance. 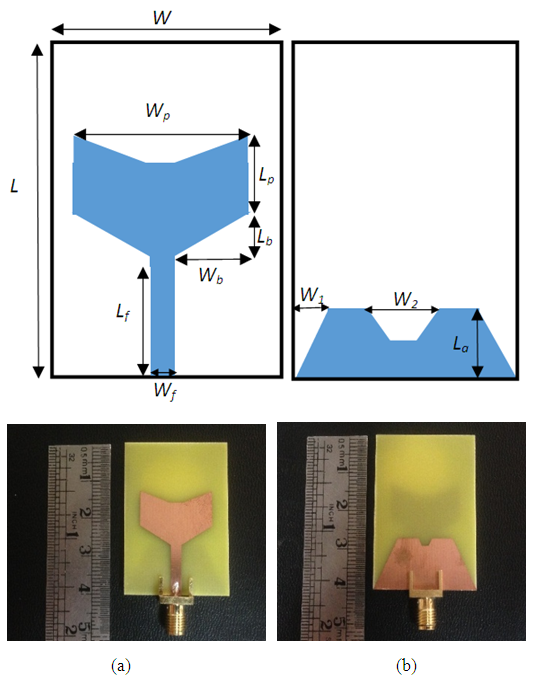 | Figure 1. Geometry and prototype of the proposed antenna (a) front view (b) back view |
Table 1. Parameters of the Antenna 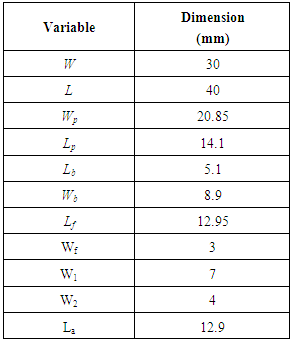 |
| |
|
This proposed antenna design consists of partial radiating patch fed by a microstrip line printed on a partial ground and FR4 substrate with a total area of 40mm×30mm, thickness of 1.6 mm, permittivity of 4.4. The microstrip feed line is designed to match a 50 Ω characteristic impedance. With the objective to increase the impedance bandwidth, four different bandwidth enhancement techniques are used. Both the radiating patch and the ground plane have bevel shapes at both ends. The CST Microwave Studio is used for Simulation to optimize design. It is done in both frequency and time domain. The different types of time domain signals are used for the UWB system. The Gaussian signal offers a good mix between time and frequency domain. It is not necessary for the frequency domain performance to ensure that the UWB antenna also performs well in the time domain. Therefore, in order to study the antenna characteristics in time domain, a Gaussian pulse as shown in Figure-2 is used as an excitation signal. The power spectrum density of Gaussian pulse is also presented. 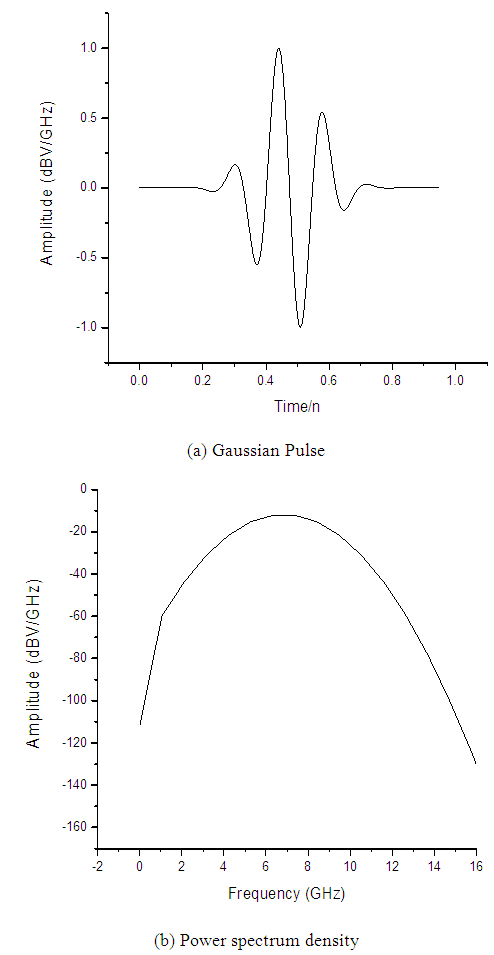 | Figure 2. Excitation signal in time domain and power spectrum density |
3. Results and Discussion
The simulated and the measured return loss S11 of the proposed antenna is shown in Figure 3.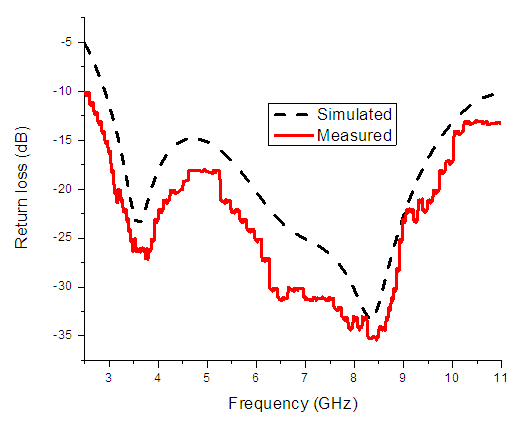 | Figure 3. Simulated and measured return loss of the proposed antenna |
It is observed that, the measured return loss curve for antenna is less than –10 dB from 3 to 10.6 GHz and shows good agreement with the simulated result and thus satisfy the UWB requirement. The distortions in the measured signal is because of system internal noise in the process of fabrication and also some effect of the external noise.Figure 4 illustrates the simulated VSWR for the proposed antenna design.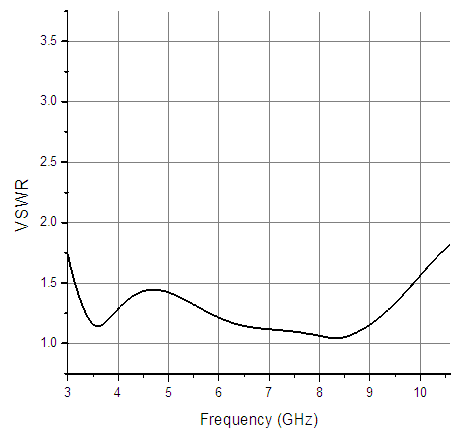 | Figure 4. Simulated VSWR of the proposed antennas |
Group delay of the designed antenna is presented with both face to face and side by side interaction as shown in figure 5. The group delay of UWB is another important criterion parameter in designing antenna, which shows the distortion in the pulse signal. It shows the rate of change of the transmission phase angle with respect to frequency. From the figure, the simulated group delay for face to face shows less variation on broader bandwidth except for sharp changes at the upper bound frequency at 10.5GHz. The antenna gain also shows the antenna characteristics and how good the antenna is designed. It is the ratio of the power radiated by the antenna in a given direction, to the radiation intensity that would be obtained if the power accepted by the antenna were radiated isotropically [9]. The simulated results of maximum gain in dBi of the proposed antenna is as shown in Figures 6. 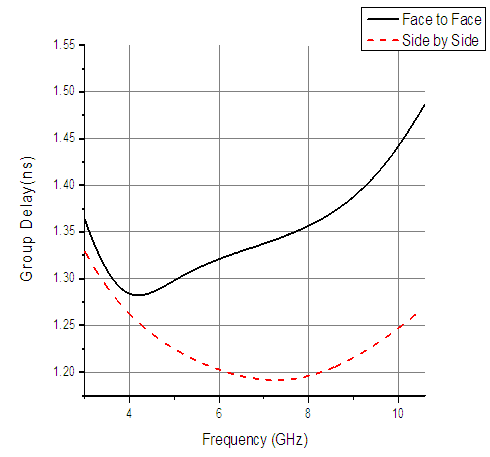 | Figure 5. Group delay of proposed antenna |
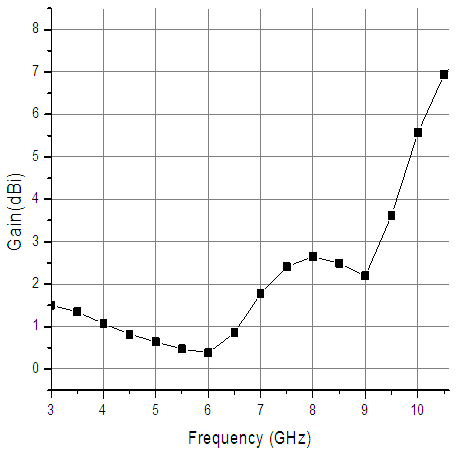 | Figure 6. Simulated gain of the proposed antenna |
Radiation pattern shows the radiation characteristics of the antenna as a function of space coordinate. The performance of linearly polarized antenna in terms of E-plane and H-plane patterns are often described. The E-plane is defined as maximum radiating plane containing the electric field vector, whereas the H-plane is defined as the maximum radiating plane containing the magnetic field vector. Figure 7 shows the simulated two dimensional E-plane and H-plane at three different frequencies. In the E–plane, the value of azimuth angle ф of 0°, 45° and 90° while for the H–plane the value of elevation angle θ of 0°, 45° and 90° are taken into consideration. The plot for radiation is utilized for three frequencies within UWB band, which are 3.5 GHz at the lower bound, 6.5GHz at the middle bound and 10.5 GHz at the upper bound. It can be seen that the designed antenna almost achieved the omnidirectional radiation pattern which prefers most.It can be seen that the radiation patterns of the base antenna are constant over the UWB frequency range. Figure 7 shows that, the base antenna has omnidirectional patterns in the H–plane and seemingly omnidirectional patterns in the E–plane.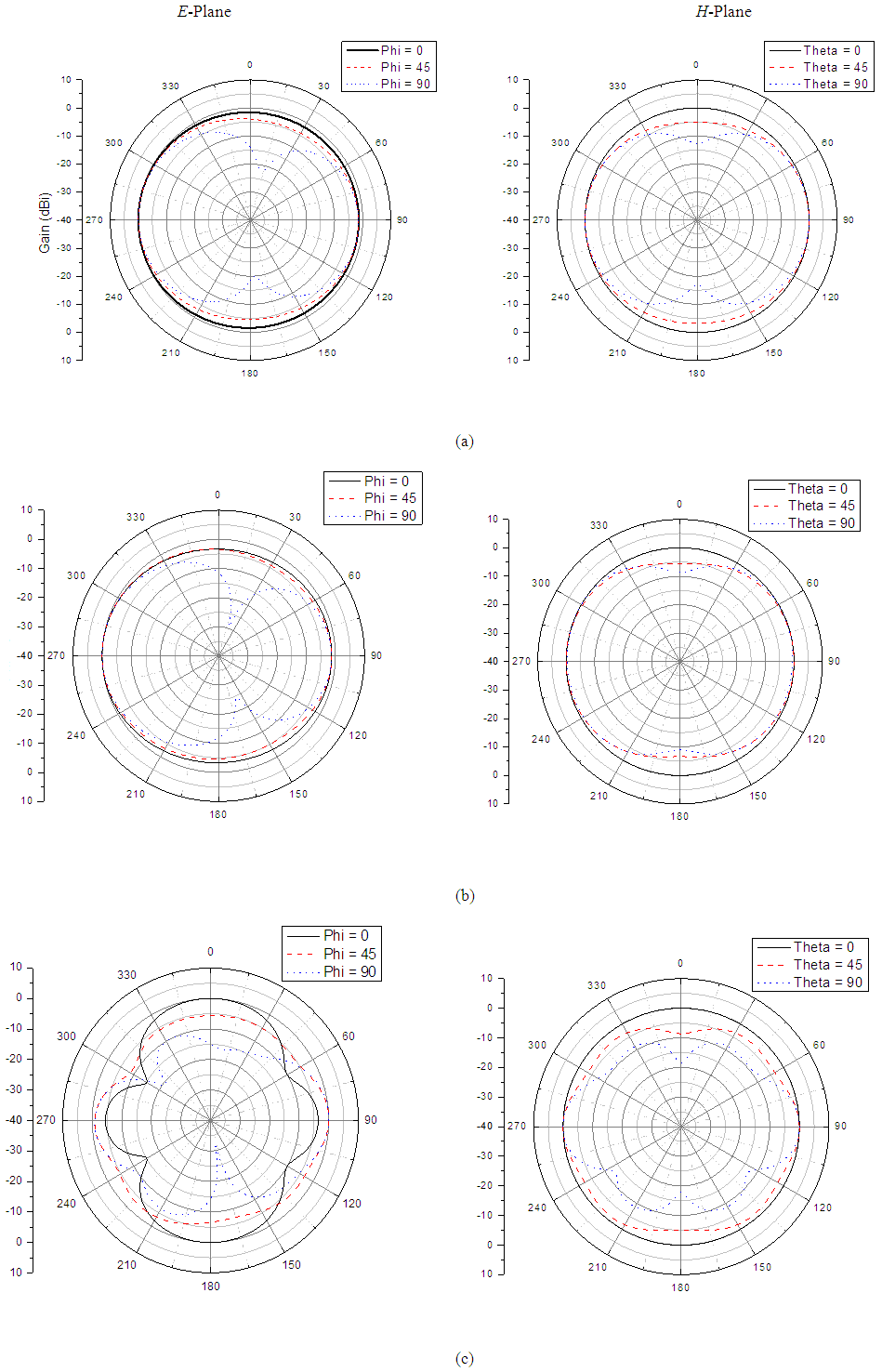 | Figure 7. Simulated E-plane and H-plane radiation pattern at (a) 3.5 GHz (b) 6.5 GHz (c) 10.5 GHz |
4. Time Domain Analysis
In order to measure the antenna efficiency in time domain analysis, the pulse base signal is excited with the Gaussian pulse. Figure 8 shows the radiated E field which is virtually place probe in simulation to study the effect of radiated signal. From this figure the antenna is fixed at azimuth angle ϕ of 90, and the probe is placed at four different positions for θ of 0°, 30°, 60° and 90°. The results shows that the proposed antenna has good potential in transmitting UWB signals with minimum distortion.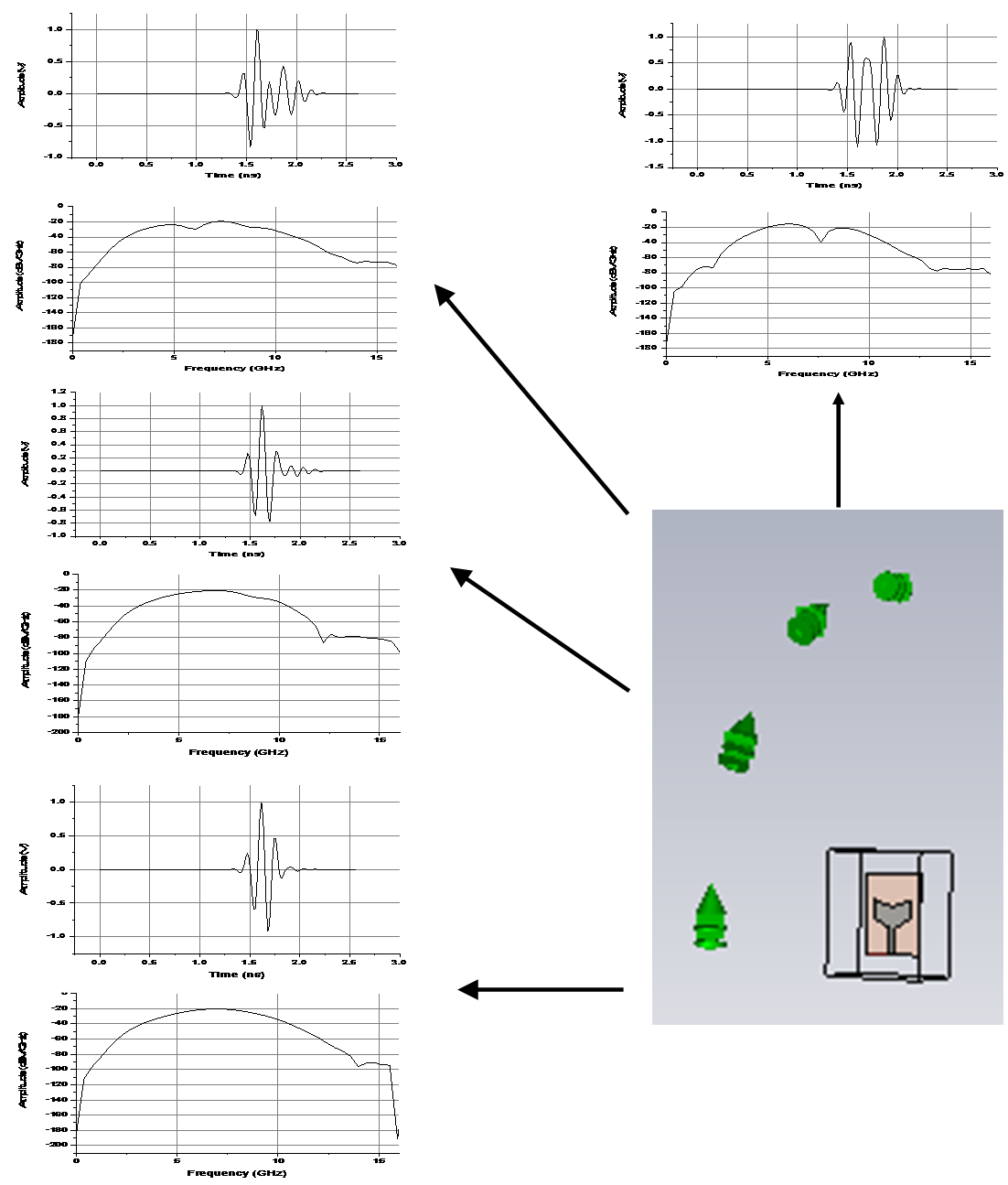 | Figure 8. Signal seen by virtual probe and respective power spectra density |
5. Conclusions
This paper starts from a compact patch bevel shape microstrip ultra-wideband antenna for UWB applications. Frequency domain and time domain analysis has been carried out for proposed antenna. Total size of the antenna is equal to 40mm×30mm and have the ability to achieving an input impedance bandwidth from 2.8 to 11 GHz. The measured results of the proposed antenna fulfil the -10 dB return-loss requirement for UWB as defined by the FCC from 3.1 to 10.6 GHz. In addition, the antenna provides omnidirectional radiation pattern in both E-planes and H-planes. Finally, the proposed antenna can be considered as a strong candidate for cost effective UWB applications due to its very wide bandwidth.
References
[1] | Hertz, Heinrich, Electrical Waves, London: Macmillan and Co., 1893. |
[2] | W. Pam Siriwongpairat and K.J. Ray Liu, “Ultra-Wideband Communications Systems: Multiband OFDM Approach”, ©John Wiley & Sons, 2007. |
[3] | Breed, G., “A summary of FCC rules for ultra-wideband communication,” High Frequency Electronic, pp.42 – 44, Jan. 2005. |
[4] | K.-S. Lim, M. Nagalingam, and C.-P. Tan, “Design and construction of microstrip UWB antenna with time domain analysis,” Progress In Electromagnetics Research, vol. 3, pp. 153164, 2008. |
[5] | Ian Oppermann, Matti Hämäläinen and Jari Iinatti, “UWB Theory and Applications”, ©John Wiley & Sons, 2004. |
[6] | S.H. Choi, J.K. Park, S.K. Kim and J.Y. Park, "A new ultra-wideband antenna for UWB applications", Microwave Opt. Techno!. Lett., Vo!' 40, No. 5, pp. 399-401, Mar. 2004. |
[7] | Barbarino, S. and F. Consoli.. “UWB circular slot antenna with an Inverted-L notch filter for the 5 GHz WLAN band," Progress in Electromagnetic Research, Vol. 104, 1-13, 2010. |
[8] | Gabriela Quintero, J.-F. Zrcher, and Anja K. Skrivervik, “System Fidelity Factor: A New Method for Comparing UWB Antennas,” IEEE Transactions on Antennas and Propagation, vol. 59, no. 7, pp. 25022512, Jul. 2011. |
[9] | Constantine A. Balanis, “Antenna Theory” (2nd Edition) JohnWiley & Sons, INC, Canada, 1997. |