Mukesh Gohel1, H. B. Sirajwala2, Anusha Chacko3
1Department of Biochemistry, B. J. Medical College, Ahmedabad, 380016, India
2Department of Biochemistry, Medical College, Baroda, 390001, India
3Department of Biochemistry, GDERS Dental College, Siddhapur, 384151, India
Correspondence to: Mukesh Gohel, Department of Biochemistry, B. J. Medical College, Ahmedabad, 380016, India.
Email: |  |
Copyright © 2012 Scientific & Academic Publishing. All Rights Reserved.
Abstract
Diabetes mellitus (DM) comprises a group of common metabolic disorders that share common phenotype of hyperglycemia. Understanding the pathogenesis and preventing long-term complications have been major goals of research in diabetes mellitus. Emerging scientific evidences has disclosed unsuspected influence between iron metabolism and type 2 Diabetes mellitus. Present study was undertaken to assess level of serum free iron concentration in type 2 Diabetes mellitus patients with good and poor glycemic control and find out correlation between serum free iron concentrations with glycemic control. A cross sectional study consists of 150 patients out of them 50 patients having type 2 DM with good control (Group II), 50 patients with type 2 DM with poor control (Group III) and 50 normal healthy control (Group-I) were selected. Statistically significant increase in free iron concentration in group III cases compare to Group I and Group II. There was a statically significant positive correlation between free iron concentration and FBS, PP2BS and Glycated Hemoglobin. In conclusion, Serum free iron concentration was higher in patients with type 2 diabetes mellitus with poor control. Also there was a positive correlation with serum free iron concentration and glycemic control. These suggest important role of iron in metabolic derangement in diabetic patients and its complications.
Keywords:
Type 2 Diabetes Mellitus, Serum Free Iron Concentration, Glycemic Control
Cite this paper: Mukesh Gohel, H. B. Sirajwala, Anusha Chacko, Serum Free Iron Concentration in Patients with Type 2 Diabetes Mellitus with Good and Poor Control and Its Correlation with Glycemic Control, International Journal of Diabetes Research, Vol. 2 No. 2, 2013, pp. 33-38. doi: 10.5923/j.diabetes.20130202.03.
1. Introduction
Diabetes mellitus (DM) is now one of the most common non-communicable diseases globally. It is the leading cause of death in most countries. Complications from diabetes, such as coronary artery and peripheral vascular disease, stroke, diabetic neuropathy, amputations, renal failure and blindness are resulting in increasing disability, reduced life expectancy and enormous health costs for virtually every society. It is a chronic, incurable, costly, and increasing but largely preventable non communicable disease which is responsible for millions of deaths annually, debilitating complications and incalculable human misery. Diabetes is undoubtedly one of the most challenging health problems in 21st century[1].Diabetes mellitus (DM) comprises a group of common metabolic disorders that share common phenotype ofhyperglycemia[2]. Hyperglycemia not only defines the disease but is the cause of its most characteristic symptoms and long-term complications[3]. Because the development of complications is linked to the accumulation of glycation adducts in tissue proteins. The core of the issue is glycemic control. Amongst the various markers of glycemic control, glycated hemoglobin has now been established as the most reliable[4]. Optimal monitoring of glycemic controlinvolves plasma glucose measurements and measurement of hemoglobin A1c. These measurements are complementary: the patient’s glucose measurements provide a picture of short-term glycemic control, whereas the A1c reflects average glycemic control over the previous 2 to 3months [2]. Glycated hemoglobin is formed by the glycosylation of hemoglobin. Its value represents the glycemic status of a person over the last two to three months. HbA1c should thus be kept to less than 7% for patients in general and to less than 6% for individual patients. A1c is the primary target for glycemic control[5].Iron is a major component of earth’s crust, but its own chemistry limit utilization and also sets basic for its toxicity. The central importance of iron in the pathophysiology of disease is derived from the ease with which iron is reversibly oxidized and reduced. This property, while essential for its metabolic functions, makes iron potentially hazardous because of its ability to participate in the generation of powerful oxidant species such as hydroxyl radical[6].Emerging scientific evidences has disclosed unsuspected influence between iron metabolism and type 2 diabetes. The relationship is bi-directional – iron affects glucosemetabolism, and glucose metabolism impinges on several iron metabolic pathways. Oxidative stress and inflammatory cytokines influences these relationships, amplifying and potentiating the initiated events. Iron induced damage might also modulate the development of chronic diabetescomplications. The extent of these influences should be tested in large scale clinical trials, searching for the usefulness and cost-effectiveness of therapeutic measurements that decrease iron toxicity. The study of individual susceptibility and of the mechanism that influences tissue iron and damage are proposed to be valuable in anticipating and treating diabetic complications[7].There is considerable current interest in the relationship between insulin and iron pool in the body. Insulininfluences the iron uptake and storage by increasing the cell surface transferrin receptors, reciprocally iron influences the insulin activity by interfering with glucose uptake and utilization. Iron causes hyperinsulinemia by decreasing the insulin uptake and metabolism by hepatocytes. Iron in its free form i.e., in non-transferrin bound form is known to induce oxidation of biomolecules through Heber-Weiss and Fenton reactions by producing harmful hydroxyl radicals[5].
2. Materials and Methods
2.1. Study Design and Subjects
This study was a hospital based cross sectional study conducted at shree sayajirao general hospital and medical college, Vadodara (India) between January 2009 to October 2010. A cross sectional study consists of 150 subjects out of them 50 patients having type 2 diabetes mellitus with good control (Group II), 50 patients with type 2 diabetes mellitus with poor control (Group III) and 50 normal healthy control (Group-I) were selected. Subjects were recruited according to simple random sampling method meeting the selection criteria.
2.2. Selection Criteria
2.2.1. Inclusion Criteria
The subjects selected for study were grouped as follows:Group I – Control group (n=50)This group consisted of age and sex matched healthy subjects. They were free from any ailment which could affect the parameters under study. They were not on any medication. They were taken from general population.Group II – Diabetes Mellitus type 2 patients with good glycemic control (n=50)This group consisted of patients with type 2 Diabetes mellitus with duration less than 8 years, Glycated hemoglobin (HbA1C) level less than 7%. They were on life style modifications and oral hypoglycemic drugs and free from clinical evidence of any chronic complication of diabetes mellitus Group III – Diabetes Mellitus type 2 patients with poor glycemic control (n=50)This group consisted of patients with type 2 Diabetes mellitus with duration more than 8 years, Glycatedhemoglobin (HbA1C) level more than 7%. They were on life style modifications, oral hypoglycemic drugs, insulin orcombination of all three and associated with one or more chronic complication of diabetes mellitus for e.g. diabetic nephropathy, diabetic retinopathy, heart disease, diabetic neuropathy.
2.2.2. Exclusion Criteria
The patients with type 1 diabetes mellitus, hemolytic anemia, hemoglobin variants, pregnancy, hepatic disease and infectious diseases like tuberculosis, sarcoidosis etc were excluded from this study.
2.3. Ethical Considerations
The Institution’s Ethical Committee approval was obtained prior to the enrolment of subjects. The objectives of study were explained to all eligible subjects for this study. Informed written consent of all subjects included in the study was obtained for involvement in study groups and for venipuncture. Emphasis was given that participation in this study was voluntary.
2.4. Questionnaire and Bio Data Collection
A questionnaire was specifically designed to obtain information which helps to select individuals according to the selection criteria of the study. The questions also focused on socio demographic data (age, sex) and background characteristics of diabetes (duration and type of diabetes mellitus, mode of anti-diabetic therapy, any complication).
2.5. Blood Sample Collection
A 5 ml of venous blood was drawn from each volunteer using a disposable vacutainer system in fasting condition (Plain, EDTA and Fluoride). Post prandial (2 hour) sample collected in fluoride vacutainer for PP2BS estimation. Serum or plasma separated within half an hour and stored at 2-8°C temperature till analysis was done.
2.6. Analysis of Sample
Fasting and Post prandial (2 hour) blood sugar (FBS & PP2BS) estimated by Glucose Oxidase-Peroxidase(GOD-POD) enzymatic end point method. (Kit: Quantitative determination by glucose oxidase peroxidase method (Trinder GOD-POD) Mfg by Spinreact)[12]. Glycated hemoglobin (HbA1C) concentration was measured byImmuno turbidimetric method (Kit: Quantitative determination of glycated hemoglobin (HbA1c) in human blood by latex turbidimetry Mfg by Spinreact)[13]. Serum Free IronConcentration was done by Ferrozine Method. (Kit: Quantitative determination of Serum iron by Ferrozine method Mfg by Coral Crest biosystems)[14]. All biochemical investigation performed on fully automatic analyzer I.S.E. srl MIURA. Hemogram and Urine examination were done in pathology laboratory. Fundoscopy and Electrocardiogram were done in respective department.
2.7. Statistical Analysis
The data collected during the current study were recorded and analysed statistically to determine the significance of different parameters by using SPSS package for windows version 16.0.
3. Results
As shown in table 1, 2 and 3 mean serum free iron concentration in Group-I, Group II and Group III was 104.70, 111.36 and 223.36 μg/dl respectively. The difference between mean serum free iron concentration between group 1 and group 3 as well as between group 2 and group 3 is statistically highly significant (with p value <0.0001). But the difference between group 1 and group 2 is not significant (p value is 0.3042).Table 1. Serum free iron concentration between Group I (Healthy control) and Group II cases (Type 2 DM with good glycemic control)  |
| |
|
Table 2. Serum free iron concentration between Group I (Healthy control) and Group III cases (Type 2 DM with poor glycemic control)  |
| |
|
Table 3. Serum free iron concentration between Group II (Type 2 DM with good glycemic control) and Group III cases (Type 2 DM with poor glycemic control)  |
| |
|
Table 4. Prevalence of increased serum free iron concentration in study groups (in %) 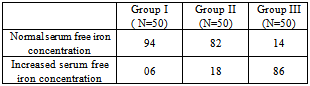 |
| |
|
As shown in table 4, the prevalence of increased serum free iron concentration among Group II and Group III cases is 18% and 86% respectively. The Group I patients had 6% cases with increased value of serum free iron concentration level.Table 5. Correlation between serum free iron concentration (μg/dl) and GHbA1 (%), FBS (mg/dl) and PP2BS (mg/dl) 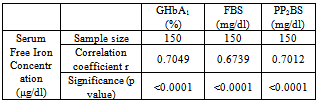 |
| |
|
Table 5 shows positive correlation between serum free iron concentration with Glycated hemoglobin (GHbA1), fasting blood glucose (FBS) and postprandial blood glucose (PP2BS) and these values are statistically significant (p<0.0001).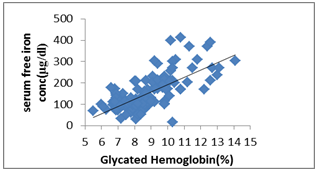 | Figure 1. Scatter dot diagram shows correlation between – serum free iron concentration (μg/dl) and Glycated Hb (%) |
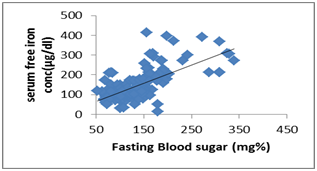 | Figure 2. Scatter dot diagram shows correlation between - serum free iron concentration (μg/dl) and FBS (mg %) |
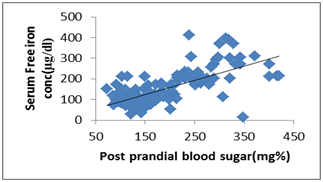 | Figure 3. Scatter dot diagram shows correlation between - serum free iron concentration (μg/dl) and PP2BS (mg %) |
Table 6. Comparison of other variables between study groups 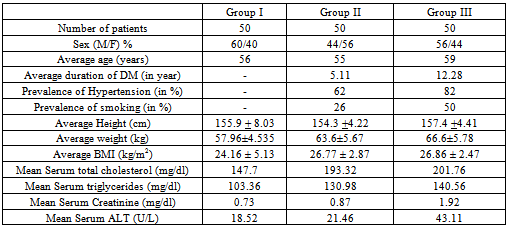 |
| |
|
4. Discussion
Present study was undertaken to assess level of serum free iron concentration in type 2 diabetes mellitus patients with good and poor glycemic control and find out correlation between serum free iron concentrations with glycemic control. It was carried out in Shree Sayajirao General Hospital and Medical College, Vadodara. A cross sectional study consists of 150 patients out of them 50 patients having type 2 diabetes mellitus with good control (Group II), 50 patients with type 2 diabetes mellitus with poor control (Group III) and 50 normal healthy control (Group-I) were selected.In our study, we found mean serum free iron concentration in Group III, Group II and Group I were 223.36 μg/dl, 111.36μg/dl and 104.70 μg/dl respectively. Statistically significant increase in free iron concentration in group III cases (p<0.0001) compare to Group I and Group II. However we did not observe increase in free iron in group II cases that were in good glycemic control. The prevalence of increased serum free iron concentration in Group III and Group II were 84% and 18% respectively whereas in Group I was only 6%, There was a significant positive correlation between free iron concentration and FBS, PP2BS and Glycated Hemoglobin which measures short and long term glycemic control in type 2 diabetes mellitus patients. These relationship is statically significant (p value<0.0001).Jeevan K Shetty et al has shown relationship between free iron and glycated hemoglobin in uncontrolled type 2 diabetes patients associated with complications[8]. S.P.Wolff studied free radicals, transitional metals and oxidative stress in the etiology of diabetes and its complications[10]. Zia A. Khan et al has studied glucose-induced regulation of novel iron transporters in vascular endothelial cell dysfunction and also shown increased iron indices and its association with the development of diabetes and its complications[11].Manoj Kar and A.S.Chakraborti has studied Effect of glycosylation on iron-mediated free radical reactions of hemoglobin and demonstrated that H2O2 induced iron release is more from HbA1c than that from nonglycosylated hemoglobin (HbA0). In the presence of H2O2, HbA1c degrades arachidonic acid and deoxyribose more efficiently than HbA0, which suggests that iron release is more with HbA1c compared to HbA0. Increased rate of oxidation of HbA1c in the presence of nitrobluetetrazolium is indicated by an increase in methemoglobin formation. HbA1c exhibits less peroxidase activity than HbA0. These findings on glycosylation-induced functional properties of hemoglobin suggest a mechanism of increased formation of free radicals and oxidative stress in diabetes mellitus[12].Iron is an essential nutrient with limited bioavailability. When present in excess, iron poses a threat to cells and tissues, and therefore iron homeostasis has to be tightly controlled. Iron’s toxicity is largely based on its ability to catalyze the generation of radicals, which attack and damage cellular macromolecules and promote cell death and tissue injury[9].Iron’s toxicity is largely based on Fenton and Haber–Weiss chemistry, where catalytic amounts of iron are sufficient to yield hydroxyl radicals (OH•) from superoxide (O2• ─) and hydrogen peroxide (H2O2), collectively known as “reactive oxygen intermediates” (ROIs).
In this milieu, redox active iron catalyzes the generation of not only hydroxyl radicals, but also of organic reactive species, such as peroxyl (ROO˙), alkoxyl (RO˙), thiyl (RS), or thiyl-peroxyl (RSOO˙) radicals.
Free radicals are highly reactive species and may promote oxidation of proteins, peroxidation of membrane lipids, and modification of nucleic acids. An increase in the steady state levels of reactive oxygen (and nitrogen) species beyond the antioxidant capacity of the organism, called oxidative (and nitrosative) stress, is encountered in many pathological conditions, such as chronic inflammation, ischemia–reperfusion injury, or neurodegeneration. Excess of redox active iron aggravates oxidative (and nitrosative) stress and leads to accelerated tissue degeneration. This is evident in disorders of hereditary or secondary iron overload[7].Interacting pathways linking glucose and iron metabolism1. Insulin influences iron metabolism. Insulin is known to cause a rapid and marked stimulation of iron uptake by fat cells, redistributing transferrin receptors from an intracellular membrane compartment to the cell surface. Insulin is also responsible for the increased ferritin synthesis in cultured rat glioma cells. Importantly, transferrin receptors have been shown to co localize with insulin-responsive glucose transporters and insulin-like growth factor II receptors in the microsomal membranes of cultured adipocytes, suggesting that regulation of iron uptake by insulin occurs in parallel with its effects on glucose transport.2. Iron influences glucose metabolism. Reciprocally, iron influences insulin action. Iron interferes with insulin inhibition of glucose production by the liver. Hepaticextraction and metabolism of insulin is reduced with increasing iron stores, leading to peripheral hyperinsulinemia. In fact, the initial and most common abnormality seen in iron overload conditions is liver insulin resistance. There is some evidence that iron overload also affects skeletal muscle, the main effecter of insulin action.3. Oxidative stress influences both glucose and iron metabolism. Oxidative stress induces both insulinresistance[by decreasing internalization of insulin] and increased ferritin synthesis. Iron is intimately linked to oxidative stress. Iron participates, through the Fenton reaction, in the formation of highly toxic free radicals, such as hydroxide and the superoxide anion, which are capable of inducing lipid peroxidation. For iron to act as a prooxidant agent, it must be in its free form. Iron can be released from ferritin by the action of reducing agents that convert Fe3+ into Fe2+. Glycation of transferrin decreases its ability to bind ferrous iron and, by increasing the pool of free iron, stimulates ferritin synthesis. Glycated holotransferrin is additionally known to facilitate the production of free oxygen radicals, such as hydroxide, that further amplify the oxidative effects of iron. 4. Cytokines influence iron and glucose metabolism. Cytokines simultaneously cause an increase in transferrin receptors on the cell surface, favoring tissue deposition of iron and insulin resistance[7].The Role of Iron in Complications of DiabetesThe importance of protein glycation is well known in the pathogenesis of diabetic vascular complications. Transition metals also play a role in protein glycation induced by hyperglycemia. It has been shown that glycated proteins have a substantial affinity for the transition metals, and the bound metal retains redox activity and participates in catalytic oxidation. Thus, should similar glycochelates form in vivo, reactions mediated by the chelates could be involved in the vascular complications of diabetes.[6].Role of iron in diabetic nephropathyAnimal studies provide considerable evidence for the role of iron and oxidants in diabetic nephropathy. Oxidativestress from factors such as hyperglycemia, advanced glycation end products, and hyperlipidemia further contribute to the availability of intracellular iron that can generate and viciously worsen oxidative stress and renal damage. Most importantly, the pathogenic role of iron in progression is indicated by the observation that progression can be prevented either by an iron-deficient diet or chelators[6].Role of iron in endothelial and vascular diseasePathologic mechanisms for iron in promoting vascular disease can be derived from cell culture studies, animal models, and human functional studies (vascular reactivity). In cell culture models, the addition of NTBI to human endothelial cell cultures increases surface expression of adhesion molecules and also increases monocyte adherence to the endothelium. In human studies of end-stage renal disease patients, intravenous iron therapy has been shown to increase vascular and systemic oxidative stress, promote atherosclerosis, and increase the risk of arterial thrombosis. Further, intravenous iron has been shown to cause impaired flow-mediated dilatation in the brachial artery, a surrogate for endothelial dysfunction. Conversely, improvement in vascular reactivity after phlebotomy in patients withhigh-ferritin type 2 diabetes further supports these observations. Plasma NTBI measures reactive forms of iron that result in increased oxidative stress and cell injury. Alternatively, better methods of measuring excess free/catalytic iron need to be developed and validated[6].Poor glycemic control causes increased glycation of proteins, especially hemoglobin, which releases the iron in its free state. Hence increased presence of free iron in its Fe3+ state in association with hyperglycemia might have caused decreased in the levels of protein bound thiols and increase in lipid hydro peroxides. Positive correlationbetween FBS and HbA1c as well as free iron and HbA1c, indicates hyperglycemia causing increased glycation of hemoglobin and increased release of free iron from glycated proteins like hemoglobin. This makes a vicious cycle ofhyperglycemia, glycation of hemoglobin and increase in levels of free iron. This increased presence of free iron pool will enhance oxidant generation leading damage to biomolecules and lead to complications. However, at present the exact nature of free iron pool in vivo is not clearly known, it needs further studies in this population with various study designs to know the catalytic action of free iron and its relation to glucose.Increase level of serum free iron in patient with type 2 diabetes mellitus with poor control and positive correlation of serum free iron concentration with glycemic control in our study indicate important role of free iron indevelopment diabetic complication.
5. Conclusions
Serum level of free iron concentration was higher in patients with type 2 DM with poor control. Also there was a positive correlation with serum free iron concentration and glycemic control. These suggest important role of iron inmetabolic derangement in diabetic patients and its complications.Further a large case control studies and studies at the molecular level are required to know the role of serum free iron concentration in modifying the effect of insulin and oxidative stress in diabetes mellitus and its complication.
ACKNOWLEDGEMENTS
I am greatly thankful to my respected guide Dr H. B. Sirajwala for her constant inspiration, encouragement and support during this research work.
References
[1] | A call to action on diabetes. International Diabetes federation, November 2010. |
[2] | Alvin C. Powers. Diabetes mellitus, Harrison’s Principle of Internal Medicine 16th Edition, Kasper et al; ch 323; 2152-2179. |
[3] | S Thakur, V Chauhan, RC Negi. Role of HbA1C in Diabetes Mellitus. Journal of Indian Academy of Clinical Medicine (JIACM) 2009; 10(1&2):52-54. |
[4] | H. B. Chandalia and P. R. Krisnaswamy. Glycated Hemoglobin. Current Science 25th December 2002; 83(12): 1522-1532. |
[5] | Nitin S. HbA1c and factors other than diabetes mellitus affecting it. Review Article Singapore Med Journal 2010; 51(8): 616-622. |
[6] | S Swaminathan et al. The role of Iron in diabetes and its complications. Diabetes care July 2007; 30(7). |
[7] | Jose manuel, Fernandez real et al. Cross talk between Iron metabolism and diabetes. Diabetes 2002; 51: 2348-2354. |
[8] | Jeevan K Shetty et al. Relationship between Free Iron and Glycated Hemoglobin in uncontrolled type 2 diabetes patients associated with complication. Indian Journal of clinical Biochemistry 2008; 23(1): 67-70. |
[9] | G. Papanikolaou, K pantopoulos. Iron metabolism and toxicity. Toxicology and applied pharmacology 2005; 202:199-211. |
[10] | S P Wolff. Diabetes mellitus and free radicals. British medical bulletin 2010; 49(3): 642-652. |
[11] | Zia A Khan et al. Glucose-induced regulation of novel iron transporters in vascular endothelial cell dysfunction. Free radical research 2005; 39(11): 1203-1210. |
[12] | Manoj Kar and A S Chakraborti. Effect of glycosylation on iron-mediated free radical reactions of hemoglobin. Current science March 2001; 80(6):770-773. |
[13] | Quantitative determination of glucose by glucose oxidase peroxidase method (Trinder GOD-POD); Spinreact. |
[14] | Quantitative determination of glycated hemoglobin (HbA1c) in human blood by latex turbidimetry; Spinreact |
[15] | Quantitative determination of Serum iron by Ferrozine method; Coral Crest biosystems |