Pavana Kumara B., John Paul Vas, Shreeranga Bhat, Karthik Madhyastha N.
Department of Mechanical Engineering, St Joseph Engineering College, Mangaluru, India
Correspondence to: Pavana Kumara B., Department of Mechanical Engineering, St Joseph Engineering College, Mangaluru, India.
Email: |  |
Copyright © 2017 Scientific & Academic Publishing. All Rights Reserved.
This work is licensed under the Creative Commons Attribution International License (CC BY).
http://creativecommons.org/licenses/by/4.0/

Abstract
Natural fiber reinforced composites are gaining tremendous attention among researchers and academicians off late. The ecofriendly nature and sustainability in addition to low cost and easy availability are the main reasons. Recently several studies are focussing on the vibration characteristics of natural fibre reinforced composites. The present work deals with the study of vibration characteristics of hybrid composite material in which chemically treated natural fibers like bagasse and banana are used as reinforcing element. The composite is fabricated by hand layup technique and its vibration properties are studied where vibration test was executed by using resistance strain sensors to detect the dynamic strain in the composite plates. Graphs are drawn to compare the composite material with different proportion of bagasse and banana fibre. Finally, the project is clinched based on the comparative results obtained.
Keywords:
Epoxy, Banana fibers, Bagasse fibers, Hybrid composite
Cite this paper: Pavana Kumara B., John Paul Vas, Shreeranga Bhat, Karthik Madhyastha N., A Study on the Vibration Characteristics of Bagasse-Banana Fibre Hybrid Composite, International Journal of Composite Materials, Vol. 7 No. 5, 2017, pp. 150-154. doi: 10.5923/j.cmaterials.20170705.04.
1. Introduction
Over the last thirty years composite materials, plastics and ceramics have been the dominant emerging materials. The volume and numbers of applications of composite materials have grown steadily, penetrating and conquering new markets relentlessly. Modern composite materials constitute a significant proportion of the engineered materials market ranging from everyday products to sophisticated niche applications. While composites have already proven their worth as weight-saving materials, the current challenge is to make them cost effective. The efforts to produce economically attractive composite components have resulted in several innovative manufacturing techniques currently being used in the composites industry. The composites industry has begun to recognize that the commercial applications of composites promise to offer much larger business opportunities than the aerospace sector due to the sheer size of transportation industry. Thus, the shift of composite applications from aircraft to other commercial uses has become prominent in recent years. The introduction of newer polymer resin matrix materials and high-performance reinforcement fibres of glass, carbon and aramid, the penetration of these advanced materials has witnessed a steady expansion in uses and volume. The increased has resulted in an expected reduction in costs. High performance FRP can now be found in such diverse applications as composite armouring designed to resist explosive impacts, fuel cylinders for natural gas vehicles, windmill blades, industrial drive shafts, support beams of highway bridges and even paper making rollers [1]. For certain applications, the use of composites rather than metals has in fact resulted in savings of both cost and weight. Some examples are cascades for engines, curved fairing and fillets of pre-existing structures that must be retrofitted to make them seismic resistant, or to repair damage caused by seismic activity. Whilst the use of composites will be a clear choice in many instances, material selection in others will depend on factors such as working, lifetime requirements, number of items to be produced (run length), complexity of product shape, possible savings in assembly costs and on the experience & skills the designer in tapping the optimum potential of composites. In some instances, best results may be achieved using composites in conjunction with traditional materials. Fibres are a class of hair-like material that are continuous filaments or are in discrete elongated pieces, like pieces of thread. They can be spun into filaments, thread, or rope. They can be used as a component of composites materials. They can also be matted into sheets to make products such as paper or felt. Fibres are of two types: natural fibre and manmade or synthetic fibre.Many researches have been carried out to study the vibration characteristics of natural fiber reinforced polymer composites. They say that these natural fibres are stumpy cost fibres with low density and high specific properties. These are eco-friendly and non-abrasive [2]. Further safety is also considered for which maximum amplitude of the vibration must be in the limited [3]. Hence vibration analysis has become very important in designing a structure to know in advance its response and to take necessary steps to control the structural vibrations and its amplitudes.The objective of present work is to collect Bagasse and Banana fibre and keep it for chemical treatment. Further fabrication of natural fibre reinforced polymer using hand layup process. Finally testing the composite material fabricated to study its vibrational characteristics.
2. Methodology
2.1. Materials Used
The composite specimens were manufactured using epoxy resin Araldite LY 556 as matrix along with Aradur HY951 hardener. The matrix was reinforced with Banana fibers and Sugarcane bagasse.
2.2. Fabrication
The Sugarcane bagasse and Banana fibres were collected from various sources and treated with Sodium Hydroxide (NaOH) and Acetic acid solutions. The fibres were soaked for 24 hours in each of the two solutions and dried under sunlight. Dry fibres are cleaned and shredded to obtain short fibres of approximately same size. Dry fibres thus obtained were mixed thoroughly with epoxy resin in various proportions and poured into the mould cavity made of wood. The shape and size of the mould were in accordance with the dimensions of specimens required. Table 1 provides details of the specimens prepared.Table 1. Specimens prepared 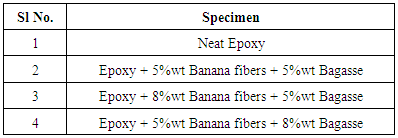 |
| |
|
2.3. Test Description
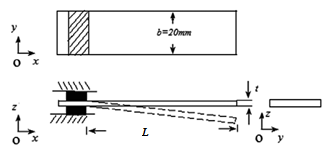 | Figure 1. Vibration testing setup |
The prepared specimens were tested for their vibration characteristics. The vibration test was executed by using resistance strain sensors to detect the dynamic strain in the composite plates. The resistance strain gauges (120 Ω) were attached on both sides of the cantilever plates. An A/D converter and computer were also used. The specimens 220 × 20 × 3 mm (L × B × T) were clamped in the form of cantilever beams with spans of 100, 150 and 200 mm. The specimens were made to vibrate by striking at the free end of the cantilever. The tests yielded the damping factor and natural frequency of the composite specimens. The natural frequency was obtained by using Fast Fourier transforms.
3. Results and Discussion
The results obtained from the tests are shown in the figures 2 to 25. In case of each specimen, the amplitude-time graph was first obtained from vibration testing. From these graphs with the help of Fast Fourier transformation method, the results were obtained in the frequency domain. Thus, it was possible to obtain the peak natural frequency in each case. Similarly, the damping characteristics were obtained from the amplitude-time graph which is tabulated in table 2. Figure 26 makes a comparison between the natural frequencies for the test specimens.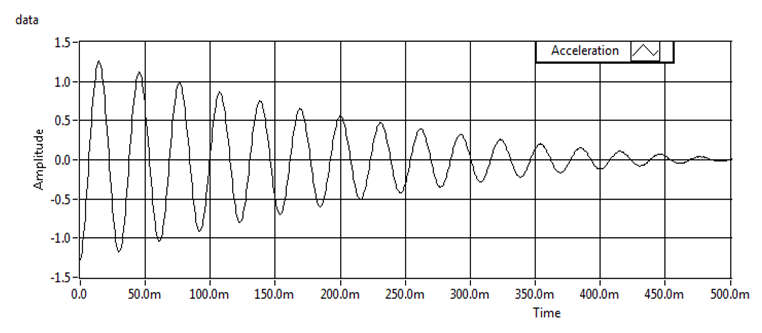 | Figure 2. Amplitude v/s time graph- Neat epoxy, clamping distance 100mm |
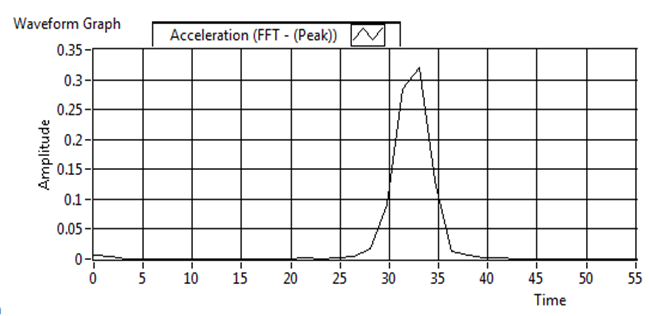 | Figure 3. Peak Natural Frequency- Neat epoxy, clamping distance 100mm |
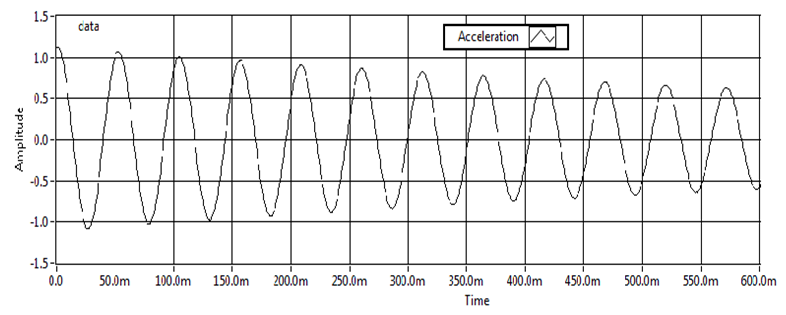 | Figure 4. Amplitude v/s time graph- Neat epoxy, clamping distance 150mm |
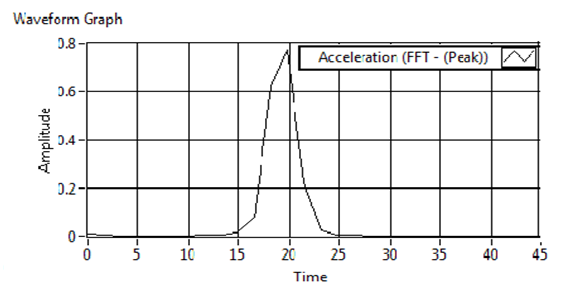 | Figure 5. Peak Natural Frequency- Neat epoxy, clamping distance 150mm |
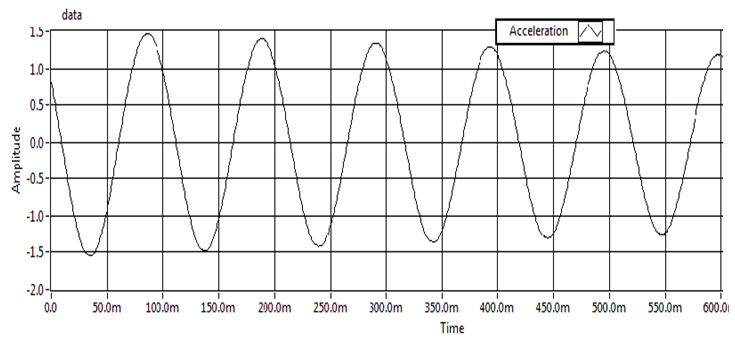 | Figure 6. Amplitude v/s time graph- Neat epoxy, clamping distance 200mm |
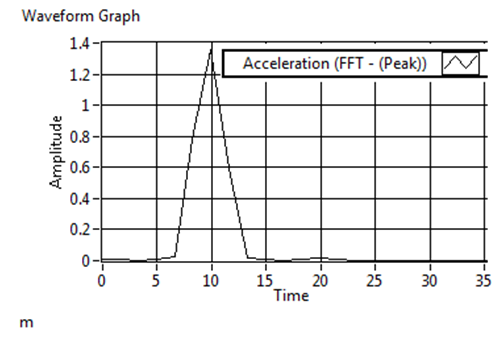 | Figure 7. Peak Natural Frequency- Neat epoxy, clamping distance 200mm |
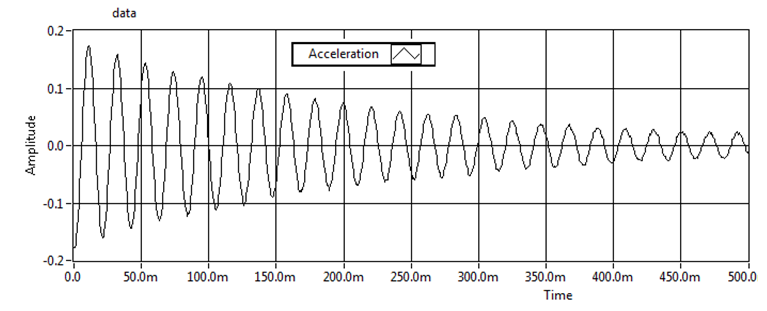 | Figure 8. Amplitude v/s time graph- 8% Banana and 5% Bagasse, clamping distance 100mm |
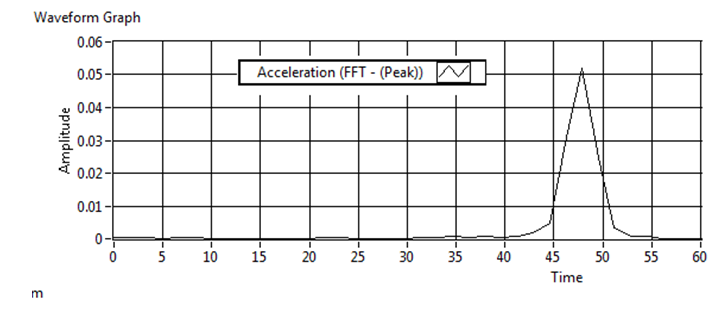 | Figure 9. Peak Natural Frequency- 8% Banana and 5% Bagasse, clamping distance 100mm |
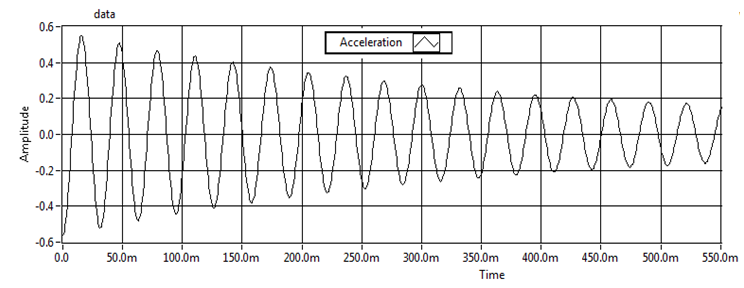 | Figure 10. Amplitude v/s time graph- 8% Banana and 5% Bagasse, clamping distance 150mm |
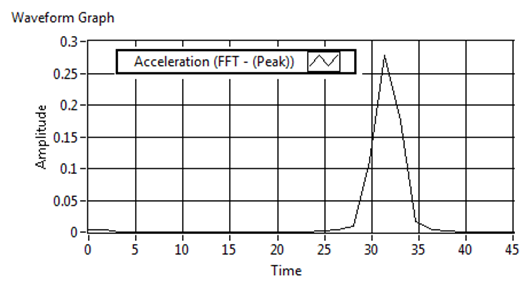 | Figure 11. Peak Natural Frequency- 8% Banana and 5% Bagasse, clamping distance 150mm |
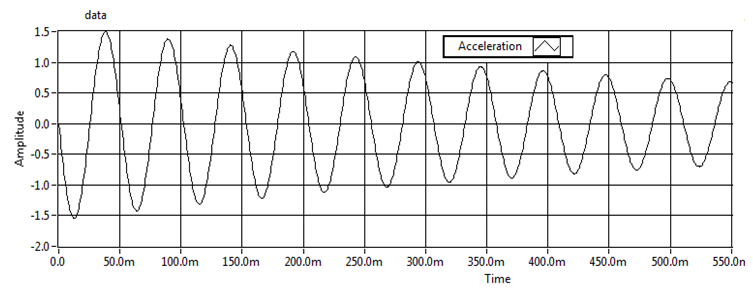 | Figure 12. Amplitude v/s time graph- 8% Banana and 5% Bagasse, clamping distance 200mm |
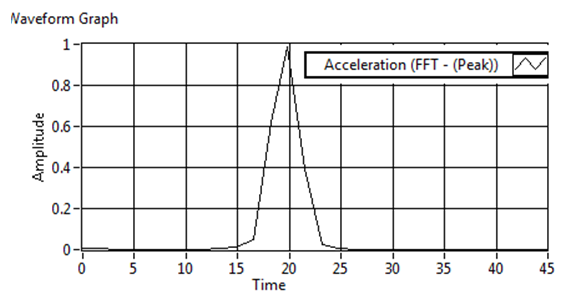 | Figure 13. Peak Natural Frequency- 8% Banana and 5% Bagasse, clamping distance 200mm |
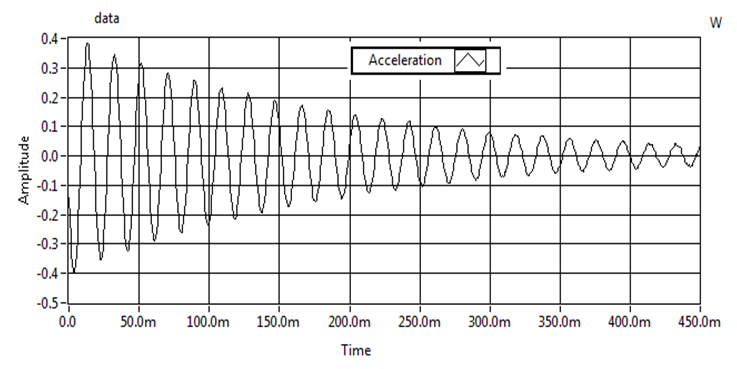 | Figure 14. Amplitude v/s time graph- 5% Banana and 8% Bagasse, clamping distance 100mm |
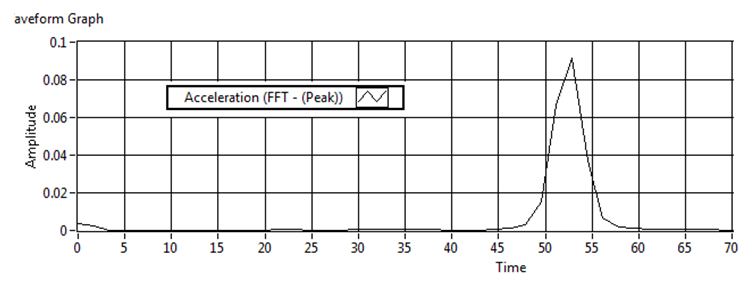 | Figure 15. Peak Natural Frequency- 5% Banana and 8% Bagasse, clamping distance 100mm |
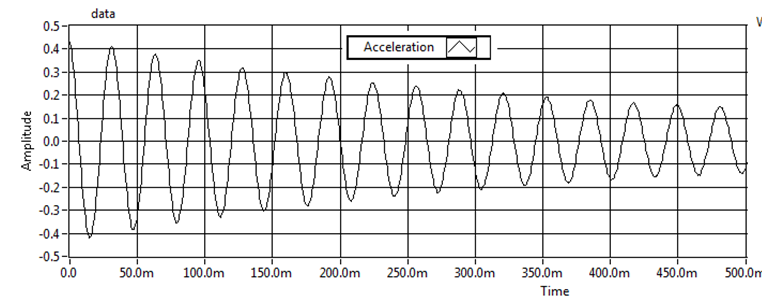 | Figure 16. Amplitude v/s time graph- 5% Banana and 8% Bagasse, clamping distance 150mm |
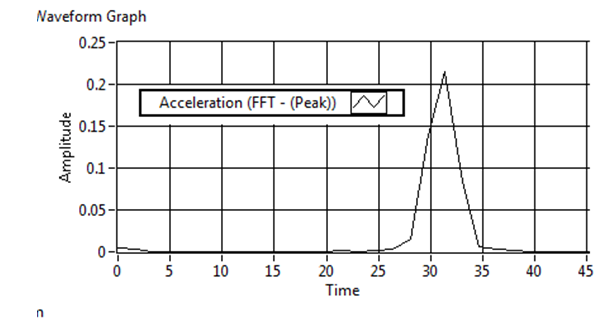 | Figure 17. Peak Natural Frequency- 5% Banana and 8% Bagasse, clamping distance 150mm |
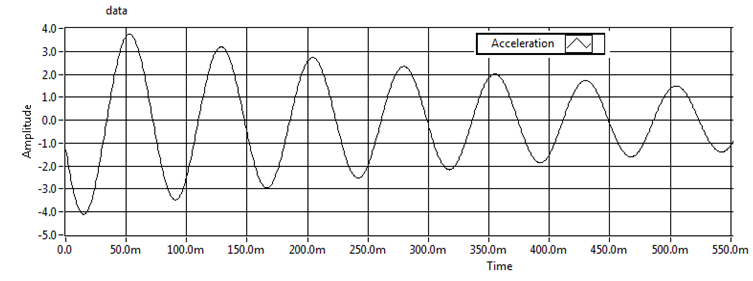 | Figure 18. Amplitude v/s time graph- 5% Banana and 8% Bagasse, clamping distance 200mm |
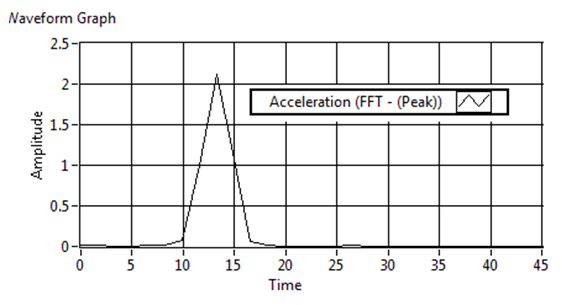 | Figure 19. Peak Natural Frequency- 5% Banana and 8% Bagasse, clamping distance 200mm |
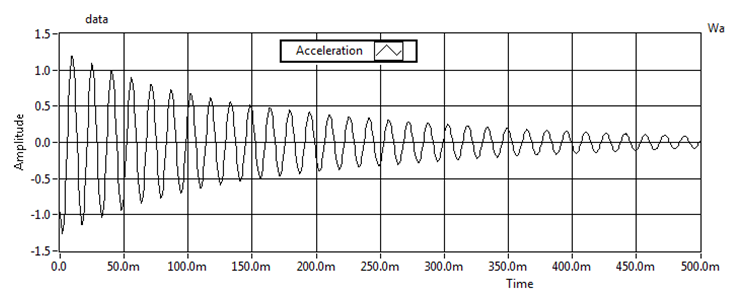 | Figure 20. Amplitude v/s time graph- 5% Banana and 5% Bagasse, clamping distance 100mm |
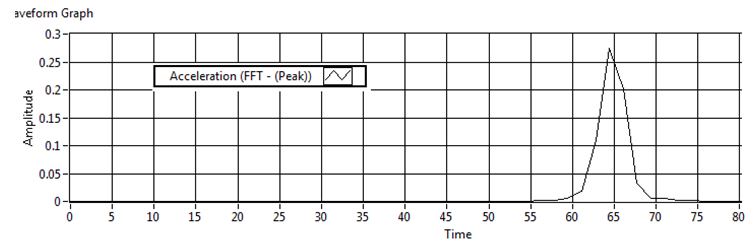 | Figure 21. Peak Natural Frequency- 5% Banana and 5% Bagasse, clamping distance 100mm |
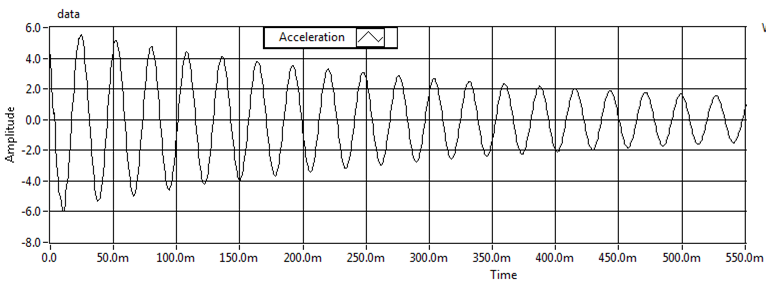 | Figure 22. Amplitude v/s time graph- 5% Banana and 5% Bagasse, clamping distance 150mm |
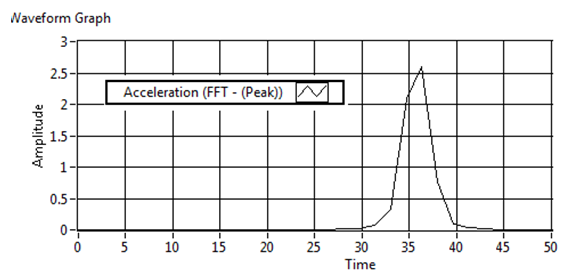 | Figure 23. Peak Natural Frequency-5% Banana and 5% Bagasse, clamping distance 150mm |
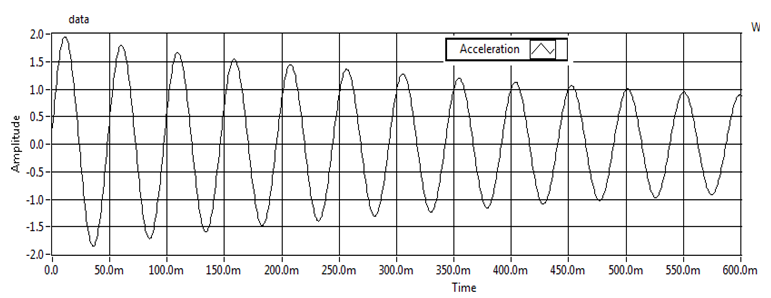 | Figure 24. Amplitude v/s time graph- 5% Banana and 5% Bagasse, clamping distance 200mm |
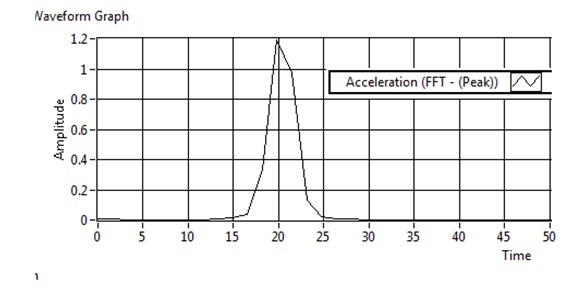 | Figure 25. Peak Natural Frequency- 5% Banana and 5% Bagasse, clamping distance 200mm |
Table 2. Vibration test results 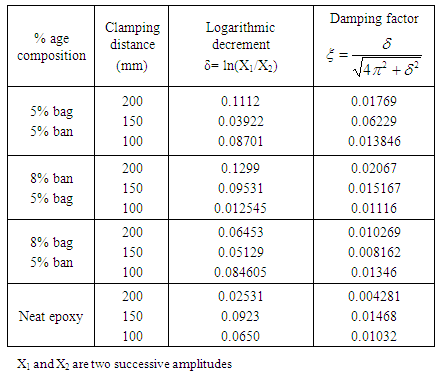 |
| |
|
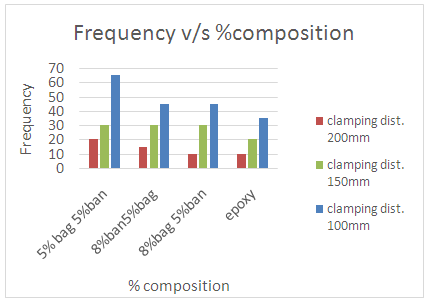 | Figure 26. Comparison of experimental results |
4. Conclusions
From the study, it can be concluded that the natural frequency of the composite increases as the clamping distance decreases. The addition of reinforcement into neat epoxy had a favourable influence on the natural frequency of the specimen because significant increase was observed. Maximum natural frequency was obtained in the case of specimen with 5%wt bagasse and 5%wt Banana fibres. However further increase in the reinforcement percentage reduced the natural frequency.
References
[1] | Punyapriya Mishra, S. K. Acharya, “Anisotropy abrasive wear behavior of bagasse fiber reinforced polymer composite,” International Journal of Engineering, Science and Technology, Vol. 2, pp. 104-112, 2010. |
[2] | K. Vigneshwaran, G. Rajeshkumar & V. Hariharan, “Vibration Characteristics of Natural Fiber Reinforced Polymer Composites - A Review,” IOSR Journal of Mechanical and Civil Engineering, pp. 13-16, 2010. |
[3] | Sharayu U. Ratnaparkhi & S.S. Sarnobat, “Vibration analysis of composite plate,” International Journal of Modern Engineering Research, Vol. 3, Issue 1, pp. 377-380, 2013. |
[4] | Acharya S.K, “Weathering Behavior of Bagasse Fiber Reinforced Polymer Composite,” Journal of Reinforced Plastics and Composites, Vol. 27, pp. 1839-1846, 2008. |
[5] | Bilba K, “Sugar cane bagasse fibre reinforced cement composites. Part I. Influence of the botanical components of bagasse on the setting of bagasse/cement composite,” Journal of Cement & Concrete Composites, Vol. 25, pp. 91-96, 2003. |
[6] | Jochen Gassan, “A study of fibre and interface parameters affecting the fatigue behavior of natural fibre composites,” Composites Part A, vol. 33(3), pp. 369-74, 2003. |
[7] | Ruys D, Crosky A, Evans WJ, “Natural bast fibre structure,” International Journal Mater Product Technology, vol. 17(1–2), pp. 2-10, 2002. |
[8] | Mwaikambo LY, Ansell MP. Chemical modification of hemp, sisal, jute, and kapok fibers by alkalization,” Journal of Applied Polymer Science, vol. 84(12), pp. 2222-2234, 2002. |