Elbashir E. Shattle1, Zohor M. Elshawi2, Salim M. Khalil3, Manal A. Erteeb4, Tebra M. Eldrhube5
1University of Tripoli, Faculty of Science Chemistry Department, Tripoli, Libya
2Higher Institute of Medical Profession Department of Medicine Lab., Elzahra
3Department of Chemistry and Chemical Technology, Faculty of Science, Tafila Technical University
4Higher Institute of Sciences and Technology, Azizia
5Chemistry Department, Azzaytuna University, Tarhona
Correspondence to: Elbashir E. Shattle, University of Tripoli, Faculty of Science Chemistry Department, Tripoli, Libya.
Email: |  |
Copyright © 2023 The Author(s). Published by Scientific & Academic Publishing.
This work is licensed under the Creative Commons Attribution International License (CC BY).
http://creativecommons.org/licenses/by/4.0/

Abstract
Theoretical calculations were performed on the phosphonium compounds as corrosion inhibitors for iron with complete optimization of geometrical parameters. The semi empirical method PM3 (Parametric Method 3) in the MOPAC program, version 8.3 (2004) Cambridge Soft Corporation, supplied by P. Bischoff. This work is aimed to give more theoretical insight to the effect of phosphonium compounds as inhibitor corrosion for iron metal. Quantum chemical parameter such as: chemical softness
, the fraction of the electrons transferred
; absolute electronegativity
the electrophilicity index
and chemical hardness
, of phosphonium halide were reported. The electronic properties such as; the energy of the highest occupied molecular orbital (HOMO), the energy of lowest unoccupied orbital (LUMO), the energy gap,
= (LUMO–HOMO). These quantum chemical parameters correlated to the inhibition efficiency. The inhibition efficiency of the investigated phosphonium compound follows the trend of: allyl- triphenylphosphonium iodide < allyl triphenylphosphonium bromide < allyl triphenylphosphonium chloride < allyl triphenylphosphonium.
Keywords:
Iron, PM3, Phosphonium compounds, Inhibitors, Corrosion
Cite this paper: Elbashir E. Shattle, Zohor M. Elshawi, Salim M. Khalil, Manal A. Erteeb, Tebra M. Eldrhube, Theoretical Studies on Allylphenylphosphonium Halides as Corrosion Inhibitors for Iron Metal, American Journal of Chemistry, Vol. 13 No. 2, 2023, pp. 36-41. doi: 10.5923/j.chemistry.20231302.02.
1. Introduction
Metals Corrosion costs millions of dollars each year. Mostly, is corrosion of iron and steel, although many other metals corrode as well? The problem with iron, as well as many other metals, is that the oxide formed by oxidation does not firmly adhere to the surface of metal and causes harm defects [1]. The corrosion causes several losses to the global economy. National economies suffer a great deal of money losses because of the wear out of machines and water and petroleum pipelines. Corrosion can be defined as the degradation of a material due to an electrochemical and physical reaction with its environment. Degradation implies deterioration of physical properties of material which is weakening the material due to a loss of cross-sectional area. Another definition of corrosion is the gradual destruction of materials by chemical reaction with its environment. Corrosion occurs in the presence of moisture and oxygen. For example when iron is exposed to moist air, it reacts to form weak brown flakes. Corrosion can also occur in materials other than metals, such as ceramics or polymers. Although in this context, the term degradation is more common. Corrosion degrades the useful properties of materials and structures including strength, appearance, and permeability to liquids and gasses.There has been a growing interest in the Organic compounds contain atoms (N,O, S …etc. which can donate electrons to unoccupied d-orbitals of metal surface, to form coordinate covalent bonds and can also accept free electrons from the metal surface (back bonding) by using their anti-bonding orbitals to form feedback bonds, are respected as good corrosion inhibitors. Most of the organic compounds, which mainly contain oxygen, sulfur, nitrogen atoms and multiple bonds, were studied as corrosion inhibitors, and showed promising results. A study of corrosion inhibitors was performed by Mutombo and Hakerman on the effect of allyl triphenylphosphonium bromide behavior on mild steel in 0.50 M sulphuric acid. It was found that the phosphonium compounds act as good electron acceptors [2].
1.1. Frontier Molecular Orbital (FMO) Theory
Fukui [3] presented a novel treatment of chemical reactivity, FMO theory, which was based on the work by Coulson and Languet-Higgins. This theory has been used by Woodward and Hoffman in their original interpretation of electro cyclic ring opening in cyclobutenes. The HOMO and LUMO of molecules have been termed the frontier molecular Orbital (FMO) [4]. The original goal of FMO theory [5] was to provide simple estimates of activation energies. Since the reactants in such a reaction do not transition state, it should be possible to estimate the difference in energy between them using perturbation theory. The reactants are treated as the unperturbed system. This is a situation of a kind that was treated by Cousin and languet Higgins in their pioneering studies. The perturbation is of a second order, corresponding [6,7] to a sum of interactions between the filled molecular orbitals of each reactant and the empty orbitals of the other i.e. interaction happens between the HOMO of on reactant and the LUMO of the other Figure 1.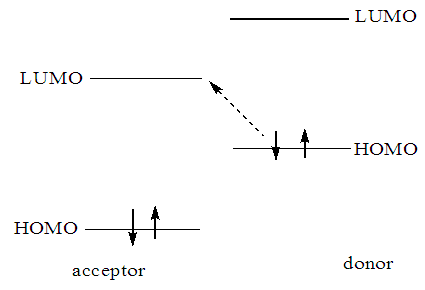 | Figure 1. Donor-acceptor interaction between donor and acceptor orbitals of two molecules |
2. Results and Discussion
Results of the calculated properties of phosphonium compounds are allyl triphenyl- phosphonium, allyl triphenylphosphonium chloride, and allyl triphenylphosphonium bromide and allyl triphenylphosphonium iodide.The optimized structures and geometries of the compounds under investigation are shown in Figure 2 and 3. Frontier Molecular orbitals (HOMO and LUMO), energy gap (∆E), hardness
, softness
, fraction of electron transferred
, and electrophililcity index
, were calculated for these compounds according to molecular orbital theory [8].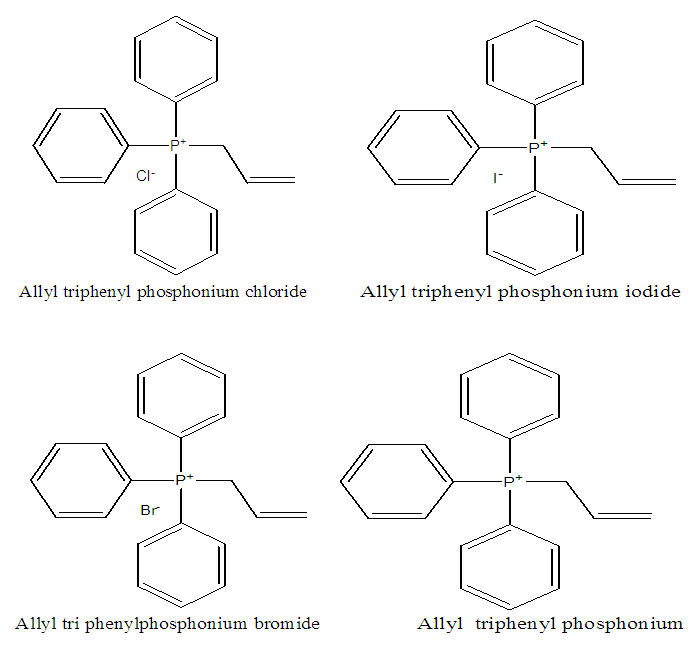 | Figure 2. Structure of the compounds to be calculated |
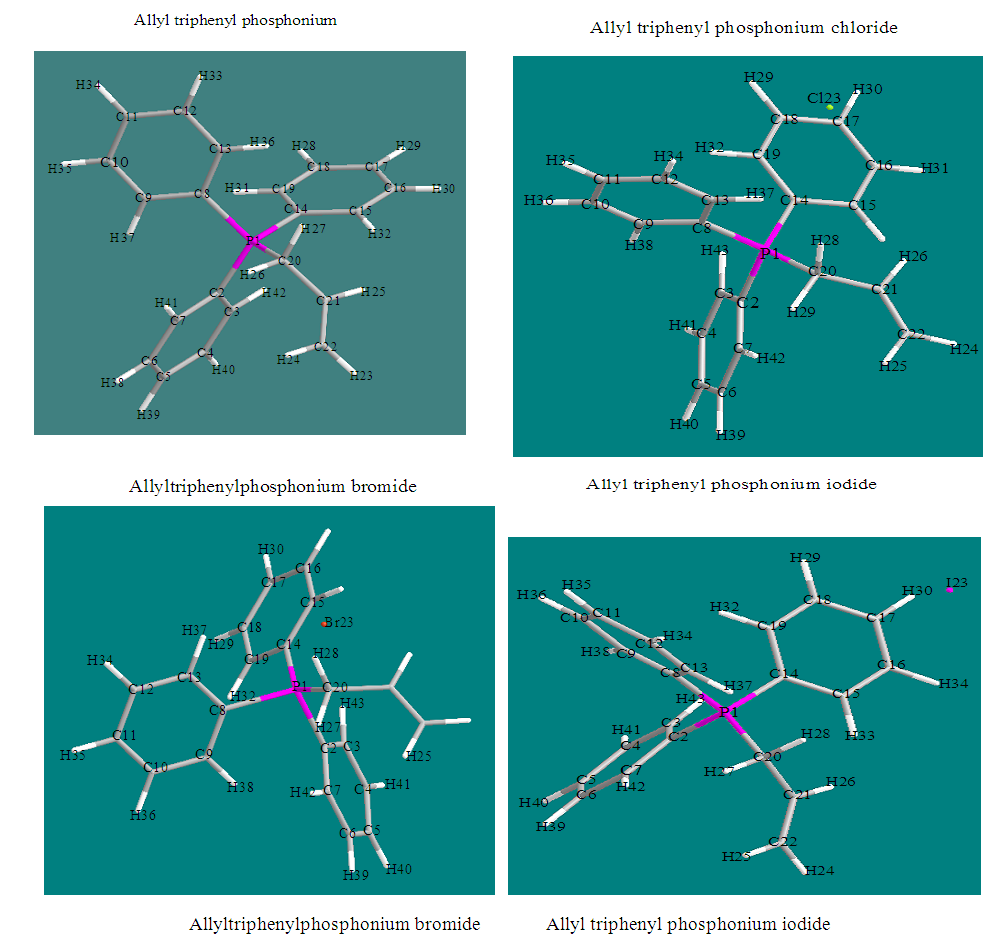 | Figure 3. Optimized geometry of the phosphonium compounds |
The EHOMO and ELUMO of the inhibitors molecule are related to the ionization potential (I) and the electron affinity (A) respectively, by the following relation:The electron affinity (A) is the energy released when an electron added to the molecule, and related to EHOMO as the following equation:
The ionization potential (I) is the energy required to remove an electron from the molecule, and related to EHOMO by the following equation:
Electronegativity
is the measure of the power of an electron or group of atoms to attract electrons towards it [9]; it can be estimated by using the equation:
The absolute electronegativity
equal:
And the absolute chemical hardness
of the inhibitor:
Chemical softness
is the measure of the capacity of an atom or group of atoms to receive electrons [10]. It is estimated by using the equation:
Global electrophilicity index
is the measure of the electrophilic tendency of a molecule; it is estimated by using the electronegativity and chemical hardness parameters through this equation. [9a, 9b]:
Where
is the chemical potential and equals the negative value of electronegativity
[9b].
is the chemical hardness and assumed to be equal to the negative of electronegativity
was proposed by Parr [9] as a measure of the electrophilic power of a molecule. When two systems, metal and inhibitor, are brought together, electrons will flow from lower
(inhibitor) to higher
(metal) until the chemical potentials become equal. The obtained values of
and
are used to calculate the fraction of the electron transferred,
from the inhibitor to metallic surface as the following [10]:
Where
and
denote the absolute electronegativity of metal and the inhibitor, respectively,
and
denote the absolute hardness of metal and the inhibitor, respectively. The values of
and
are taken as 4.0267 and = 3.8757 respectively. The difference in electronegativity drives the electron transfer, and the sum of the hardness parameters acts as resistance [9]. The calculated results of the energies of frontier molecular orbitals for the inhibitors are given in Table 1.Table 1. Calculated HOMO-LUMO energies of phosphonium compounds inhibitor and electronic charge density on phosphorus atoms 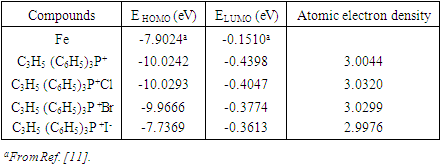 |
| |
|
The electronic charge density on the phosphorus atom changes slightly when it is compared with the charge density on the inhibitors. These results go along with a previous study on the effect of organo-phosphorus compounds on the corrosion behavior of iron [12]. According to the frontier molecular orbital (FMO) theory, the chemical reactivity is a function of interaction between HOMO and LUMO levels of reacting species [13]. EHOMO is quantum chemical parameter which is associated with the electron donating ability of the molecule. High energy value of EHOMO is likely indicates a tendency of the molecule to donate electrons to the appropriate acceptor molecule, with an empty low energy molecular orbital, ELUMO (for inhibitor) [14]. Therefore, low energy of the lowest unoccupied molecular orbital, ELUMO, indicates that, the high ability of molecule to accept electrons [15]. So the lower the value of ELUMO, is the better the molecule acceptor. The smaller the value of energy gap the better the corrosion inhibitor. Thus the binding ability of the inhibitor to metal surface increases with increasing energy of HOMOFe and decreasing the energy of the LUMOinh. The energies of HOMO and LUMO of iron were compared to the values, calculated for phosphonium derivative compounds, are given in Table 2. Table 2 shows the LUMOinh – HOMOFe (energy gap) for interaction between iron - inhibitor. Iron will act as a Lewis base while the inhibitors phosphonium compounds act as a Lewis acids. So iron will utilize the HOMO orbital to initiate the interaction with LUMO orbital of the phosphonium compounds. The interaction will have certain amount of ionic character because the values of LUMOinh – HOMOFe gap fall about 7.0 eV. Strong covalent bond can be expected only if LUMOinh – HOMOFe gap is approximately ~ zero eV [16,17].Table 2. HOMO-LUMO gap for interaction of iron-inhibitor phosphonium compounds 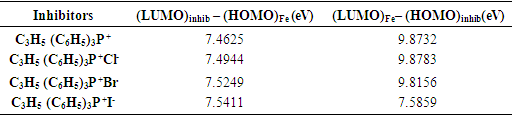 |
| |
|
All quantum chemical parameters are given in Table 3. The separation energy of the inhibitor, ΔEgap = (ELUMO – EHOMO), is an important parameter and it is a function of reactivity of the inhibitor [18]. The effectiveness of phosphonium compounds under investigation as inhibitors has been further addressed by evaluating the global reactivity parameters: The electronegativity, X, global chemical hardness, η, global softness, σ, the fraction of electrons transferred, ΔN, and electrophilicity ω, are shown in Table 3.Table 3. The calculated quantum chemical parameters for phosphonium compounds with Fe 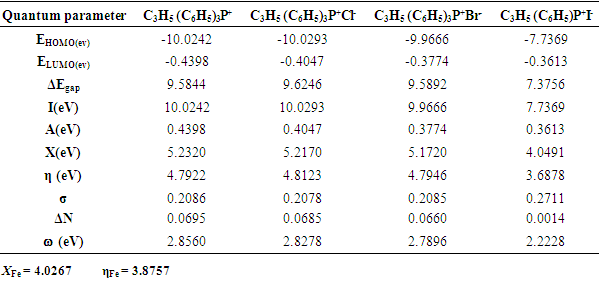 |
| |
|
Values of ΔN show the inhibition efficiency resulting from the electron-donating ability of the inhibitor. It is found that the ability of donation is in the order: allyl- triphenylphosphonium chloride > allyl- triphenylphosphonium bromide > allyl- triphenylphosphonium iodide. These results are in correspondence with the decrease in the ELUMO of these compounds (Table 3). The electrophilicity index (ω) shows the ability of the inhibitor molecules to accept electrons. Thus allyl triphenylphosphonium and allyl-triphenylphosphonium chloride exhibit the highest value of electrophilicity as compared to that of other phosphonium compounds (Table 3), which confirms its high capacity to accept electrons. So, unoccupied d orbitals of Fe atoms can accept electrons from inhibitor molecules to form a coordinate bond. Also the inhibitor molecule can accept back electrons from Fe atoms through its antibonding π- orbitals to form back-donating bonds. These results agree with the recent theoretical calculations of carbohydrates as inhibitors of iron [19]. The donation and back-donation processes strengthen the adsorption of allyl- triphenylphosphonium and allyl-triphenyl- phosphonium chloride onto the iron surface as compared to the allyl-triphenyl- phosphonium iodide and allyl-triphenylphosphonium bromide [20]. It has been reported that adsorption of chloride ions on iron increases its softness [21], which agrees with the present results in this work.
3. Structural Details
All geometrical parameters (bond lengths and bond angles) for the compounds under investigation are reported. As can be seen from the calculated geometrical parameters, that Chloride, Cl atom in (allyltriphenyl-phosphonium chloride ) causes changes in the adjacent bond lengths, while Br (allyl triphenylphosphonium bromide) and Iodine atom, I in (allyl triphenylphosphonium iodide) show almost no change in bond lengths. For the effect on bond angle: Cl, Br and I show a slight change in the bond angles at which they are attached to phosphorus atoms. This agrees with theoretical calculations of Khalil [22] and Al-Halasah and Khalil [23].
4. Electron Densities
The electron density on every atom in the compounds under investigation are calculated, It can be seen from calculated electron distribution that Cl substituent in allyl triphenyl-phosphonium chloride increases the electron density on phosphorus atom, i.e. acts as electron withdrawing group, while Br (allyl- triphenylphosphonium bromide) acts as a weak electron withdrawing. For I substituent (allyl-triphenylphosphonium iodide), the electron density decreases slightly on phosphorus atom, and acts as a weak electron releasing group [24,25]. This may be due to the low electronegativity of iodide compared to other halogens. Also the positive charge on the phosphorus atom has a tendency to attract electrons, i.e. depends on electron demand [26].
5. Conclusions
1. It can be concluded that allyl-triphenylphosphonium and allyl-triphenylphosphonium chloride are good corrosion inhibitors for iron, while allyl-triphenylphosphonium bromide and allyl triphenylphosphonium iodide are corrosion inhibitors for iron, to a less extent.2. The adsorption of the inhibitor on the metal surface is spontaneous. This study displays the reliability of the PM3 method to study corrosion inhibition of metal surfaces.
References
[1] | E. Khamis, E. S. H. El-Ashry , A. K. Ibrahim: Brit. Corros.J., 35, 150 (2000).Hill, New York (1967) |
[2] | E. E. Foad El Sherbini, Mater. Chem. Phys., 60, 286 (1999). |
[3] | K. Fukui, Acc. Chem. Res., 4, 57 (1971). |
[4] | R. B. Woodward and R. Hoffman, J.Am. Chem. Soc., 87, 395 (1965). |
[5] | M. J. S. Dewar, Frontier Orbital Theory", J. Mol. Struct. (Theo. chem.) 200(1989). |
[6] | C. A. Coul and H.C. Longuet-Higgins, Proc. R. Soc. Ser. A, 193, 447 (1948). |
[7] | M. J. S. Dewar, J. Am. Chem. Soc., 74, 3341 (1952). |
[8] | J., Kruger, Passivity of Metals – a Materials Science Perspective, "International Materials Reviews", 33, 113 (1988). |
[9] | a. R. G. Parr, L. Szentpaly, S. Liu, J. Am. Chem. Soc. 121, 1922(1999). b. E.E. Foad El Sherbini, Mater. Chem. Phys., 60, 286 (1999). |
[10] | F. Bentiss, M, Traisnel, M. Lagrenee: Brit., J., 35, 315 (2000). |
[11] | J. F. Richardson, J. M. Ball, P. M. Boorman “Structure of Tetra phenyl Chloride” Acta. Crystallographica, C42, 1271 (1986). |
[12] | P. Mutombo, Hackerman, Anti-corr. Methods and Mater, 45,413 (1998). |
[13] | A. Y. Musa, A. H. Kadhum, A. B. Mohamad, A. B. Rohoma, H. Mesmari, J. Mol. Struct. 969, 233 (2010). |
[14] | G. Gece, S. Bilgic, Corros. Sci., 51, 1876 (2009). |
[15] | I. Ahamd, R. Prasad, M. A. Quraishi, Corros. Sci., 52, 1472 (2010). |
[16] | G. Klopman, J. Am. Chem. Soc., 90, 233 (1968). |
[17] | W.B. Jensen, Chem. Rev., 78, 1(1978). |
[18] | M. K. Awad, M. R. Mustafa, M. M. Abo Elnga, J. Mol. Struct. (Theorem), 959, 66 (2010). |
[19] | S. M. Khalil, E. E. Ali and N. M. Ali, Z. Naturforsch 68a, 2013 (in press). |
[20] | T. Arslan, F. Kandemirli, E. E. Ebenso, I. Love, H. Alemu, Corros. Sci., 51, 35 (2009). |
[21] | K. Aramaki, T. Mochizuki, and H. Nichihara, J. Elec. Soc., 135, 2427(1988). |
[22] | S. M. Khalil, Z. Naturforsch., 63a, 42 (2008). |
[23] | W. F. Al-Halasah, Z. Naturforsch., 59a, 980 (2004). |
[24] | S. M. Khalil, Z. Naturforsch, 43a, 801(1980). |
[25] | S. M. Khalil, H.M. Jarjis, Z. Naturforsch, 40a, 898(1991). |
[26] | B. M. Salim and S. M. Khalil, Z. Naturforsch, 60a, 47(2005). |