Cosme Sagbo Kouwanou1, Cokou P. Agbangnan Dossa1, Euloge S. Adjou2, Fidèle P. Tchobo2, Clément Bonou3, Mohamed M. Soumanou2, Dominique C. K. Sohounhloué1
1Unit of Research in Molecular Interactions, Polytechnic School of Abomey-Calavi, University of Abomey-Calavi, Cotonou, Benin
2Unit of Research in Enzyme Engineering and Food, Polytechnic School of Abomey-Calavi, University of Abomey-Calavi, Cotonou, Benin
3Laboratory of Research in Applied Biology, Polytechnic School of Abomey-Calavi, University of Abomey-Calavi, Cotonou, Benin
Correspondence to: Dominique C. K. Sohounhloué, Unit of Research in Molecular Interactions, Polytechnic School of Abomey-Calavi, University of Abomey-Calavi, Cotonou, Benin.
Email: |  |
This work is licensed under the Creative Commons Attribution International License (CC BY).
http://creativecommons.org/licenses/by/4.0/

Abstract
Eichhornia crassipes is one of the aquatic plants that creates enormous annoyances because of its propensity to completely cover the surface of the water it colonizes, hence its negative impact on navigation, irrigation, fishing and the conservation of biodiversity. The aim of this work is its valorization by producing bioethanol of second generation; it is an optimal valorization for the production of simple fermentable sugars with high added value. In the first process which is chemical hydrolysis, the biomass was depolymerized by steam treatment. Then a second process which is enzymatic hydrolys is with Aspergillus Niger cellulase. The monitoring of kinetic parameters such as Brix, pH and glucose level showed a considerable evolution of the glucose level from 0.5 to 11.21 mg/ml according to the pretreatment time and from 11.21 to 21.46 mg/mL after the enzymatic hydrolysis according to the type of hydrolysed sample. In conclusion, this work allowed to show the impact of the thermomechanical and biotechnological processes used on the lignocellulosic biomass and to set up a time of pretreatment by steam explosion and an optimal concentration of cellulase applied to Eichhornia crassipes for its bioconversion in ethanol fuel.
Keywords:
Eichhornia crassipes, Enzymatic hydrolysis, Second generation bioethanol
Cite this paper: Cosme Sagbo Kouwanou, Cokou P. Agbangnan Dossa, Euloge S. Adjou, Fidèle P. Tchobo, Clément Bonou, Mohamed M. Soumanou, Dominique C. K. Sohounhloué, Physicochemical and Enzymatic Hydrolysis of Eichhornia crassipes for the Production of Second-Generation Bioethanol, American Journal of Chemistry, Vol. 8 No. 2, 2018, pp. 41-44. doi: 10.5923/j.chemistry.20180802.03.
1. Introduction
Greenhouse gas (GHG) emissions, especially those from the combustion of fossil fuels such as oil [1] and its price increase justify the search for raw materials and alternative technologies capable of reduce dependency on these fossil fuels and protect the environment [2, 3]. Indeed, it is accepted that the use of pure bioethanol instead of petrol allows a reduction of CO2 emissions of around 90% [4]. Lignocellulosic biomass is one of the most abundant renewable resources on earth, and certainly one of the least expensive [5]. It is composed of three main fractions of which the first, of the order of 35 to 50%, is cellulose which is a polymer of glucose. The second, called the hemicellulosic fraction, of the order of 20 to 30%, is also a polysaccharide, essentially consisting of pentoses (including xylose and arabinose) and glucose. The third is lignin (15 to 25%), a complex structure polymer based on phenyl groups [6]. The conversion of lignocellulosic biomass to ethanol fuel occurs after several stages of pretreatment and physical, chemical and biological hydrolysis to obtain fermentable sugars [2].From its scientific name Eichhornia crassipes, the water hyacinth is a perennial freshwater aquatic plant that floats on the surface of the water thanks to these petioles which have at their base a bulge of rounded shape and which acts as floats [7]. One of the inconveniences generally mentioned, when we talk about the proliferation of this plant, is its propensity to completely cover the surface of the water it colonizes, hence its negative impact on navigation, irrigation, fishing and the conservation of biodiversity, as it causes the disappearance of many species of flora and fauna [8; 9]. Article 8 (h) of the Convention on Biological Diversity recommends that contracting parties prevent the introduction of invasive species and combat their spread [10]. As a result, the recovery of this invasive bio-resource into ethanol fuel involves the evaluation of the available glucose level and it is within this framework that this study was initiated and is mainly oriented on the experimentation of water hyacinth treatment processes more precisely the optimization of the treatment temperature and the enzyme concentration used for the release of fermentable sugars from the plant for bioconversion to ethanol.
2. Material and Methods
2.1. Collection of Plant Material
Samples of Eichhornia crassipes were collected in three lacustrine areas of the political capital of Benin (Porto-Novo) which are Dangbo, Adjohoun and Bonou. Once returned to the laboratory, the samples were washed, dried and then mechanically ground.
2.2. Pretreatment with Steam Explosion
Pretreatment with steam explosion is a very promising technique for lignocellulosic biomass before bioconversion. It is a thermo-mechano-chemical process that destroys the lignocellulosic material and partially hydrolyses it. This steam explosion process was developed by W. H. Mason in 1925 for the production of hardboard [11]. It is composed of two distinct phases; steam-cracking and explosive decompression [2]. Under these conditions, 50g of the leaves and stems of Eichhornia crassipes cleaned, washed with pure water to remove impurities, cut and crushed and placed in an Erlenmeyer flask containing 0.5liter of distilled water. The substrate is pretreated with steam in an autoclave (1h at 270°C). Samples were taken every 10min to evaluate the evolution of sugar level and glucose concentration.
2.3. Ensymatic Hydrolysis
After the pretreatment stage of the raw material, a hydrolysis phase is necessary to obtain the fermentable sugars. In the present study, it is enzymatic hydrolysis which is catalyzed by enzymes called generically cellulases (compounds of endo and exo-glucanases and β-glucosidases). At first, endoglucanase would attack microcrystalline cellulose in amorphous regions, creating faults in linear chains. This action would be followed by the action at these breaking points of the exoglucanase or cellobiohydrolase, which releases cellobiose. The continuous and combined action of these two enzymes (endo- and exoglucanases) results in the complete conversion of cellulose into cellobiose and small oligosides. B-glucosidase or cellobiase finally acts on cellobiose and oligosides to give glucose [12]. Thus, the enzymatic hydrolysis of Eichhornia crassipes previously pretreated and soaked with water was carried out by adding Aspergillus Niger cellulase at four different concentrations (2, 4, 6 and 8 g of enzyme per 50 g of substrate). The incubation temperature is 50°C for 144h.
2.4. Determination of Glucose Content
The glucose content of samples are investigated by using the phenol-sulfuric colorimetric method [13, 14]. To 1 ml of untreated, pretreated or hydrolysed Eichhornia crassipes mash is first added 1ml of phenol solution (5%) then very slowly 5ml of concentrated sulfuric acid (H2SO4). The mixture is stirred, and immediately placed in a water bath at 25-30°C for 20min. A stable yellow color develops. After cooling in water at room temperature, the absorbance is measured at 490nm with the UV-Visible spectrophotometer. Two readings of concentrations are carried out per sample and the results are expressed in grams of total sugars per 100g of mutton. The glucose concentration of the samples is determined from a calibration curve previously plotted with glucose.
2.5. Brix Degree
The total soluble content (Brix, expressed in °Bx) of the different samples was determined by direct measurement using a PAL3-ATAGO digital portable refractometer according to the AOAC method [15]. It allows us to follow the evolution of all the sugars present in the material.
2.6. pH Measurement
The pH of the various samples was determined using a portable pH meter (HANNA HI 98129) by direct measurement on 20mL of Eichhornia crassipes mussels.
3. Result and Discussion
3.1. Effect of Pretreatment by Explosion on Matter
3.1.1. Evolution of the Brix Degree
Figure 1 shows the evolution of the Brix degree as a function of time. The analysis of this figure shows that the concentration of sugars in the medium remains constant during the first minute while considerable variation is noted from 30 minutes to the end of the pretreatment (1 hour) where the highest value of the brix has been registered. We can then say that the thermomechanical pretreatment step has indeed begun the fragmentation of cellulosic and hemicellulose chains. Sugar release was notable for the high degree of brix observed at the sample level after 1hour of pretreatment compared with the untreated (E0). The pretreatment with steam positively impacted the lignocellulosic substrate. In the literature, the pretreatment of biomass is absolutely essential for the biological stage because the enzymes are very little active on the raw biomass [16, 17].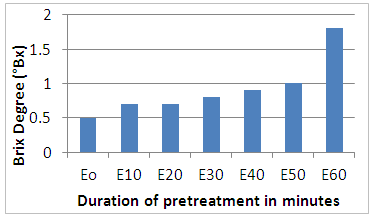 | Figure 1. Evolution of Brix Degree during pretreatment |
3.1.2. Evolution of Glucose Level
Figure 2 shows the destructuration of the lignocellulosic material and the release of some sugars. From the analysis of this figure, an increase of the glucose level after 10 minutes of pretreatment and a constancy of this rate is noted up to 20 minutes followed by a considerable variation of the glucose release as a function of the pretreatment time. From this analysis, it appears that steam pretreatment has broken the hydrogen bonds that bind the cellulose and hemicellulose molecules and allows efficient fractionation of the constituent components [2] making them more available for enzymatic hydrolysis.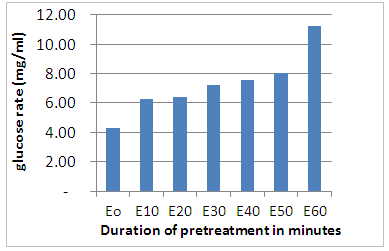 | Figure 2. Glucose release during pretreatment |
3.2. Effect of Enzymatic Hydrolysis on Matter
3.2.1. pH Evolution
Figure 3 shows the pH evolution of Eichhornia crassipes during enzymatic hydrolysis. From the analysis of this figure, there is a gradual increase in pH up to the fifth day of hydrolysis. This increase in pH could be related to the exponential growth phase of enzymes, often characterized by the production of secondary metabolites of basic types. After five days of hydrolysis, we notice that the pH of the must stabilizes, which could correspond to the phase of stabilization of the growth of the enzymes. This evolution of the pH was clearly visible in the musts of the E2 sample.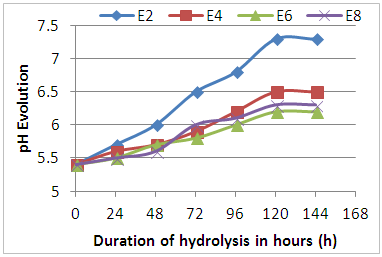 | Figure 3. Evolution of pH during enzymatic hydrolysis |
3.2.2. Evolution of the Brix Degree
Figure 4 shows the results of the evolution of all the sugars at the level of the different samples during the hydrolysis. The analysis of this figure shows that there has been a rapid decrease in the rate of sugars after 24 hours of hydrolysis followed by a gradual decrease to the fifth where we observe a stabilization of the sugar content at values depending on each the concentration of the enzymes used.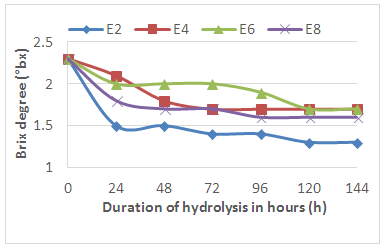 | Figure 4. Evolution of Brix Degree during enzymatic hydrolysis |
3.2.3. Evolution of Glucose
By observing the results of Figure 5 it is noted that during the first 48 h, hydrolysis was very slow in the four hydrolysed samples which can be justified by the acclimation of enzymes in the middle. But after 48h, finds that some samples are hydrolysed faster than others (E2 and E4), especially the E2 sample, which gives an interesting concentration after 96hours, 21.46mg/ml. sample E8, it is found that the release of the glucose level is not considerable during hydrolysis. This principle is fundamental because complex sugars are broken down into simple sugars by the synergistic action of enzymes that can be defined by good coordination in hydrolysis. The three types of enzymes involved in the process are endo-1,4-glucanase and exo-1,4-glucanase cellulases, which hydrolyze celluloses in cellobiose, and β-glucosidases which hydrolyze cellobioses to glucose [2, 18].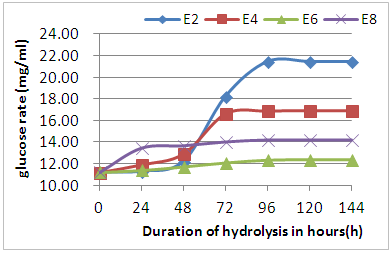 | Figure 5. Glucose release during the hour enzymatic hydrolysis |
4. Conclusions
Eichhornia crassipes is an aquatic plant that has an ethanolic potential by alcoholic fermentation because of the glucose present in its cellulosic and hemicellulosic chains. This study showed an increase in glucose content of 4.32mg/mL before pretreatment at 21.46 mg/mL after enzymatic hydrolysis. Pretreat-ment resulted in a glucose level 5 times higher than that of the untreated material. This can be explained by the ease of access of the enzyme to the glucoside bonds to be hydrolysed and the minimal loss of glucose by degradation or modification following its exposure to steam explosion conditions. Pretreatment then relaxes the structure of the cellulose and makes it more accessible to enzymatic digestion which releases monomeric reducing sugars such as glucose. The optimal time of the steam explosion and the optimum concentration for good hydrolysis are respectively one hour at a temperature of 270°C and 4% of cellulase.It will be appropriate to consider the bioconversion of glucose obtained in bioethanol and the evaluation of its fuel characteristics.
References
[1] | Novidzro K. M: 2013. Production of bioethanol by alcoholic fermentation of fruit juices of: Balanites aegyptiaca, Curcubitapepo, Dialium guineense and Opilia amentacea; p 1- 202. |
[2] | Eloutassi N, LouasteB, Boudine L, Remmal A: 2014. Physico-chemical and biological hydrolysis of lignocellulosic biomass for the production of second-generation bio-ethanol. "Nature & Technology". C- Environmental Sciences. 10. P. 10 – 14. |
[3] | Gbohaida V., Mossi I., Adjou E. S., Agbangnan Dossa C. P, Wotto D. V., Avlessi F. and Sohounhloue K. C. D: 2016. Assessment of the fermentative power of Saccharomyces cerevisiae and S. carlsbergensis in the production of bioethanol from the juice of the cashew apple; Journal of Applied Biosciences, 101:p9643 – 9652. |
[4] | Ward O. P. and Singh A.: 2002. Bioethanol technology: Development and perspectives. Adv. Applied Microbiol: 51, p.53-80. |
[5] | Saidi A.: 2011. La biomasse lignocellulosique et la bioénergie, Extrait du Portail Algéri 1077; n des En 1077; rgi 1077; s R 1077; nouv 1077; 120261; ab 120261; 1077; s. |
[6] | Ogier J.-C., Ballerini D., Leygue J.-P., Rigal L. and Pourquié J.: 1999. Production of ethanol from lignocellulosic biomass. Oil & Gas Science and Technology- IFP Review, Vol. 54, No. 1, pp. 67-94. |
[7] | www.auxbulles.com. |
[8] | Holm L.G., Plucknett D.L., Pancho J.V., Herberger J.P.: 1977. The world's Worst Weeds. Distrbution and biology. Press of Hawaii. Honolulu, 609p. |
[9] | Jiaqing, D., Wang, R., Zhongnam, F.: 1995. Distribution and Infestation of Water Hyacinth and the Control Strategy in China. Journal of Weed Science 9 (2): 12-17. |
[10] | Almoustapha O., Kenfack J. M.-R. S.: 2008. Production of biogas and compost from water hyacinth for sustainable development in Sahelian Africa. E-journal in environmental science, Vol.8, No. 1, pp. |
[11] | Wertz J-L., Richel A. and Gerin P: 2016. Prétraitements de la biomasse lignocel-lulosique. p1-58, www.valbiom.be. |
[12] | Debboub A: 2012. Enzymatic hydrolysis of lignocellulosic biomass: a new pretreatment and catalysis technology. Memory p 1- 78. |
[13] | AOAC. Association of Official Analytical Chemists. Methods of Analysis. Washington D.C. 18th Ed., 2003. |
[14] | Agbo N.G. and Ronald E. S.: 1999. Characteristics of juice from Palmyrah Palm (Borassus), fruit. Plant Foods Hum. Nutr., 42 (1), p55-70. |
[15] | Ezoua P, Kouamé D, Agbo N. G, 1999. Characteristic of the juice of the fresh pulp of the rônier fruit (Borassus aethiopum Mart).Cahier d'agriculture, 8, p 126-128. |
[16] | Wyman C. E.: 2007. What is (and is not) vital to advancing cellulosic ethanol. Trends Biotechnol 25(4): 153–157. |
[17] | Laurent P., Roiz J., Wertz J-L., Richel A. et Paquot M.: 2010. Biorefining, a promising alternative to petrochemicals Biotechnol. Agron. Soc. Environ, 14 (2) p561-566. |
[18] | Kumar R., Singh S., SingV. h, 2008 Bioconversion of lignocellulosic biomass: biochemical and molecular perspectives: J Ind Microbiol Biotechnol 35 p 377-391. |