Nguemtchouin Mbouga Marie Goletti1, Kaptso Kuaté Giscard2, Noumi Guy Bertrand3, Noudem Jean Antoine1, Allou Guy Yanick3
1Department of Applied Chemistry, National School of Agro-Industrial Sciences, University of Ngaoundere, Ngaoundere, Cameroon
2Department of Social Economy and Family Management, Higher Technical Teachers' Training College, University of Buea, Kumba, Cameroon
3Department of Chemistry, Faculty of Sciences, University of Ngaoundere, Ngaoundere, Cameroon
Correspondence to: Nguemtchouin Mbouga Marie Goletti, Department of Applied Chemistry, National School of Agro-Industrial Sciences, University of Ngaoundere, Ngaoundere, Cameroon.
Email: |  |
Copyright © 2018 Scientific & Academic Publishing. All Rights Reserved.
This work is licensed under the Creative Commons Attribution International License (CC BY).
http://creativecommons.org/licenses/by/4.0/

Abstract
In this work, batch adsorption experiments were carried out for the removal of copper (II) ions as pollutants, from aqueous solutions using montmorillonite clay as the low-cost adsorbent. Montmorillonite clay was used in its natural form as well as after treatment with saponins extracted from kernels of Balanites aegyptiaca. The modification of the montmorillonite by the saponins was done in four proportions, whose adsorption studies on the copper ions by various parameters showed that the most balanced treatment ratio is 5:1 (clay mass/saponins mass). The contact time, adsorbent dosage, pH and temperature were investigated. Results revealed that adsorption rate initially increased rapidly, and the optimal removal efficiency was reached within 40 min for the natural-clay and 12 min for the saponins-clay material. The adsorption capacity of the two clay samples decreases with increasing temperature, pH of the reaction medium, the initial dose of clay, and the initial concentration. Thus, the combination of adsorption parameters producing the most efficient result can be characterized by ambient temperature values (25°C); of neutral pH (for MMT-HS and MMT-SP respectively). The adsorption process obeys both Langmuir and Freundlich adsorption isotherms for the two samples. All these results have shown that the modification of clay with saponins increases the adsorption capacity of the clay, with Qmax(MMT-HS)=91.77 mg.g-1 against Qmax(MMT-SP)=129.02 mg.g-1. The results indicate that the use of saponins-clay materials could provide a cheap and technically viable option for heavy metals removal from polluted water.
Keywords:
Copper adsorption, Water, Montmorillonite, Balanites aegyptiaca, Saponins
Cite this paper: Nguemtchouin Mbouga Marie Goletti, Kaptso Kuaté Giscard, Noumi Guy Bertrand, Noudem Jean Antoine, Allou Guy Yanick, Adsorption of Copper (II) Ions from Aqueous Solutions by Using Natural Saponins-Clay Modified Materials: Isotherm, Kinetic and Thermodynamics, American Journal of Chemistry, Vol. 8 No. 2, 2018, pp. 29-35. doi: 10.5923/j.chemistry.20180802.01.
1. Introduction
Environmental protection is a common concern for all and is therefore considered to be a global problem that disregards all international borders. Man is at the center of pollution carrying out various activities which highlight several forms of pollution, some of which are linked to heavy metals ions such as Cu(II), Pb(II), Hg(II), Zn(II), As(V) and Cr(VI) ions [(Fan et al., 2012; Selimi et al., 2016; Yang et al., 2014)]. These pollutants are endowed with particular chemical properties which give them toxicity with respect to human beings and also to the other organisms of the animal kingdom and sometimes even vegetables [(Li et al., 2014)]. The heavy metals copper is an essential trace element, but it shows toxicity if in excess amounts in drinking water. It may, like other metals, have an inhibitory effect on sperm mobility and at the same time cause significant health problems such as stomach cramps, skin irritations, vomiting, nausea and anemia [(Garcia, 1997)]. As like the majority of heavy metals, it has the ability to accumulate throughout the food chain. Toxicity can result from any of the heavy metals if they are present less from its original limits in drinking water [(Chaitali and Jayashree, 2013)]. The World Health Organization (WHO) recommends that the maximum quantity of Cu2+ ions in drinking water should be 0.2 mg.L-1, while the European Communities Directive sets it at 0.1 mg.L-1. Moreover, the concentration of copper for which a bad flavor is detected is between 1 and 2 mg.L-1, and concentrations above 5 mg.L-1 render the water undrinkable. Several methods and techniques for the removal of Cu2+ ions from aqueous solutions have been proposed including adsorption [(Glatstein and Francisca, 2015)], precipitation [(Charerntanyarak, 1999)], bioremediation [(Amini et al., 2009)], membrane filtration [(Qdais and Moussa, 2004)], ion-exchange [(Alyüz and Veli, 2009)] and solvent extraction [(Ahluwalia and Goyal, 2007)]. Among these techniques, adsorption was considered superior due to its high efficient removal, easy operation, cost effectiveness and availability of efficient adsorbents [(Kurko and Matovi´c, 2015)]. Likewise, adsorption makes it possible to eliminate the copper ions at low concentrations (<10 mg.L-1), in contrast to other methods mentioned above. In order to satisfy these different standards, numerous methods and techniques for depollution via adsorption have been developed over the last few years. Various researchers have shown that a variety of materials of natural or biological origin have the ability to fix large quantities of heavy metals from solutions: clay substrates such as calcium kaolinite, limestone powder [(Ghazy and Ragab, 2007)], zeolite, modified clays [(Ouakouak and Youcef, 2016)], and even synthetic polymers. Although clay materials show a dual advantage of availability and reduced cost, several studies have shown that their use in the natural form, limits the total utilization of their adsorption capacity. Thus, the bridging processes, activation and precipitation etc., have enabled the valorization of these solid supports in several fields. However, some of the methods that involve the use of chemicals have in turn shown some detrimental effects on the environment. This inconvenience has prompted many researchers to investigate "bio" modification processes in order to improve the adsorptive power of the clay in an environmentally appropriate manner. Accordingly, the literature has shown that it is possible to use natural surfactants such as saponins and phospholipids, as additives that can act as organic surfactants toincrease the adsorption capacity of clays for use in insecticide formulations [(Noudem et al., 2017)]. The main objective of the present work is to investigate the performance of natural saponins treated clay for the removal of copper metal ions from aqueous solutions.
2. Materials and Methods
2.1. Extraction of Saponins
The fruits of B. aegyptiaca were bought in a market of the city of Ngaoundere Cameroon in June 2016. After the forwarding of these fruits to the laboratory, B. aegyptiaca nuts were obtained after soaking the whole fruit in distilled water for period of 24 h. The pulps of the fruits were removed by simple hand washing with water. After this the nuts were dried in an oven at 50 °C for 72 h. Then the nuts were decorticated manually to obtain the kernels. The kernels were dried at 40 °C for 24 hours after which they were ground. The paste obtained constituted the raw material in the extraction of saponins. The saponins extraction protocol used is the modified method of Noudem et al., (2017).
2.2. Qualitative Analysis of Saponins
After extraction of the saponins, qualitative analyzes were carried out, in order to verify the composition of the extract obtained. This phytochemical screening consisted of using specific reagents to determine the different families of the compounds present in the extract. The screening was done according to the work of Abdallah et al. (2012) which revealed the presence of saponins, tannins, terpenoids, alkaloids and phenolic compounds in the fruit extracts of B. aegyptiaca.
2.3. Preparation of Natural Clay Powder
The clay used in this work was obtained from the locality of Maroua, Far-North region of Cameroon. This clay was disintegrated, then conditioned to undergo extraction of the clay fraction (less than 50 μm). Extraction of the clay fraction was done using the Stokes law. The resulting clay fraction was eliminated of organic materials using hydrogen peroxide 50% (V/V).The organic-free montmorillonite was dried at 70 °C and then transformed into homosodium montmorillonite. Montmorillonite clays were homoionized using NaCl solution (1 mol.L-1), with a solid: liquid ratio of 1:200 (5 g clay/1000 mL NaCl). The ion exchange was realized at 25 °C. After each process, the clay was washed with distilled water until no chloride ions were found by AgNO3 test. To avoid the rapid evaporation of the water, the ion exchanged samples were slowly dried at 70 °C and ground to pass through a 50 μm mesh sieve. The treated Na-clay was designated Mont-HS.
2.4. Preparation of Saponins Clay Powder
For the preparation of the montmorillonite-saponins, 20 g (5 g.L-1) of the montmorillonite-Na obtained, was previously are soaked in four beakers, each containing 1 L of ultra-pure water and then stirred for 24 h by a magnetic stirrer. The various solutions of saponins are obtained by dissolving: 0.5 g; 1 g; 1.5 g and 2 g of saponins in 1 L of the ethanol and ultra-pure water mixture and adjusting the pH of the solution to 2.3 by the addition of a 0.1 M HCl solution. The various solutions of saponins are then gradually added to the suspensions of clay while stirring at a flow rate of 8 mL.min-1 using a peristaltic pump. After complete introduction of the saponins into the Erlenmeyer flask, the mixture is stirred for 1 hour. The pH is again adjusted to 2.3 by the addition of 0.1 M HCl solution into the medium. The distilled water/ethanol ratio is 1:2 (V/V). The mixture obtained is allow to stand for 24 h at room temperature. After this, the resulting saponins-montmorillonite complex is separated by centrifugation. The pellet obtained is washed several times with distilled water until the disappearance of the excess of saponins (absence of foam). After drying at 40 °C. in an oven, the clays treated with the different proportions of saponins are ground in a porcelain mortar and passed through a 50 μm sieve meshin in order to remove the large particles. These operations were repeated twice for each of the proportions montmorillonite-HS / saponins. At the end of the treatments, the clays treated with saponins were obtained in the following ratios (clay mass / saponin mass): 5:0.5; 5:1; 5:1.5 and 5:2.
2.5. Absorbate
Salt of copper (CuSO4, 5(H2O)) was used as a source of metals ions. Dilute hydrochloric acid and sodium hydroxide solutions were used for pH adjustment. Distilled water was used for all experimental investigations.
2.6. Adsorption Experiments
Adsorption tests were performed by batch experiments, under stirring at room temperature (25°C). Each adsorption study was performed by adding 0.5 g of the adsorbent to 20 mL of a given concentration of the adsorbate. At the end of the given contact time, the solid and liquid phases were separated by decantation and filtration with Whatman filter paper no. 40. Subsequently, a few drops of ammonia were added, which act as complexing agent, giving the solution a blue color. The measurement was carried out using as reference a solution consisting of distilled water and drops of ammonia. The initial (C0) and equilibrium (Ce) concentrations of Cu2+ ions in the solutions were determined by the Beer-Lambert method in UV-Visible Spectrophotometry. Each experiment was repeated and the mean value was calculated in order to minimize errors. The amount of Cu2+ ions adsorbed was calculated from the formulae: 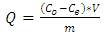 | (1) |
Percent removal of Cu2+ ions is calculated according to the formulae: | (2) |
Where Q is the uptake capacity (mg.g-1), C0 is the initial ion concentration (g.dm-3), Ce is the concentration of ions remaining in solution at equilibrium (g.dm-3), V is the volume of ion solution used (dm3) and m is the mass of the adsorbent.Both Langmuir and Freundlich isotherms have been studied. The Langmuir isotherm could be represented by the following equation: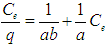 | (3) |
Where Ce is the equilibrium aqueous copper (II) ion concentration (g.L-1), q the amount of metal ions adsorbed per gram of adsorbent at equilibrium (mg.g-1), a and b are the Langmuir constants related to the maximum adsorption capacity and energy of adsorption, respectively. Freundlich adsorption isotherm is mathematically expressed as follows: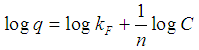 | (4) |
Where, KF is an indicator of the adsorption capacity and 1/n is the adsorption intensity. By plotting log q versus log C, values of KF and n can be determined from the intercept and slope of the plot, respectively.
3. Results and Discussion
3.1. Extraction of Saponins
The yield of total saponins of B. aegyptiaca kernels extracted using 100% methanol was about 6.7%. This result is close to that of Noudem et al., (2017), which extracted the saponins from the kernels of B. aegyptiaca from the Fare-North of Cameroon and obtained a yield of 8.84%. Phytochemical screening performed on the crude saponins is presented in Table 1. It appears from this table that the extract obtained contains only saponins.Table 1. Phytochemical screening for n-butanol extract of B. aegyptiaca kernels 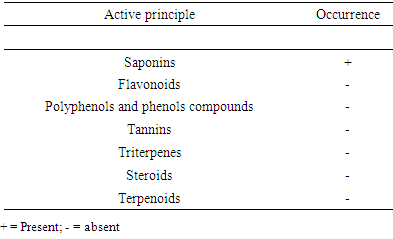 |
| |
|
3.2. Effect of Agitation Time and Search for the Effective Mass of Saponins for the Treatment of Clay
To evaluate the amount of saponins necessary for the treatment of 5 g of clay, the differently treated clays were used under the same conditions. The adsorbed amount as a function of time of the untreated clay is shown in Figure 1 and those of the clays treated in Figure 2. 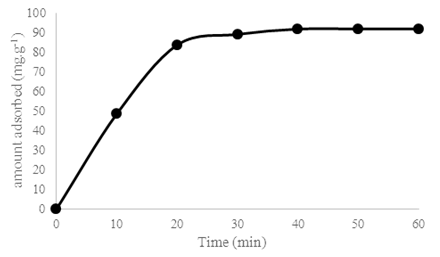 | Figure 1. Effect of contact time on the removal of Cu2+ by the montmorillonite-HS clay at T=25 °C; V= 20 mL; m(MMT-SP) = (0.1±0.01)g; [Cu2+]0 = 1 g.L-1, ant the pH of the polluting solution) |
Figure 1 can be subdivided into three parts: the first part between 0 and 20 minutes where a rapid increase in the adsorbed amount is observed; the second part from 20 to 40 minutes where a very small increase in the adsorbed amount is observed and the last part from 40 to 60 minutes where the adsorbed amount is constant. The equilibrium time is therefore 40 minutes. This time characterizes the maximum adsorption which is 91.8 mg.g-1. This time is higher than that of Ouakouak and Youcef, (2016) who worked on the adsorption of Cu2+ ions by a montmorillonite-rich bentonite from north western Algeria and found an equilibrium time of 20 min. However, the maximum quantity of adsorbed Cu2+ ions (91.8 mg.g-1) is much greater than that found by this montmorillonite-rich bentonite.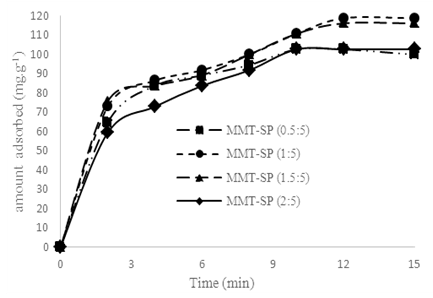 | Figure 2. Effect of contact time on the removal of Cu2+ by the different clays treated with saponins at T = 25 °C; V = 20mL; m(MMT-SP) = (0.1±0.01)g; [Cu2+]0 = 1 g.L-1, and the pH of the polluting solution |
The curves of figure 2 show a common variation. It is observed that they are initially characterized by rapid growth in the first 3 min, and then they continue to increase but this time slowly between 4 and 10 min. After these 10 min, all curves tend to become linear. Considering the time scale by which all these absorbance were measured (less than 15 min), it can be deduced that the adsorption phenomenon was carried out rapidly with respect to the untreated clay. This speed of adsorption reflects the surfactant effect of the saponins applied. These graphs make it possible to determine an adsorption stage, although individually varying but well delimited between 10 and 12 min. Thus the values of 10 min for the treated clays were obtained in the proportions 5:0.5 and 5:2, and 12 min for the proportions of 5:1 and 5:1.5.These results are justified by the fact that for the MMT-SP (5:0.5), the saturation is complete but the quantity of saponins is not completely repaired on the surface of the MMT-HS and seems insufficient. However, for MMT-SP (5:2), the amount of saponins is largely sufficient at the point where it tends to form a double layer on the surface of the clay. This second layer which is not strongly fixed will be released quickly during the process of adsorption of Cu2+ ions. The molecules of saponins dissociated from the adsorbent and present in the reaction medium will again attempt to bind for a second time on the montmorillonite. This second attempt of fixation is presented as a competitive adsorption phenomenon to that of the Cu2+ ions, hence a low adsorption capacity for the clay treated in the 5:2 ratio. With regards to MMT-SP (5:1 and 5:1.5), and the treatment seems to be balanced especially in the proportion 5/1 where an adsorbed amount is greater than all other proportions. These observations make it possible to conclude that for a treatment of 5 g of clay with saponins 1 g of saponins is necessary. The equilibrium time for the MMT-SP treated at this proportion is 12 min which is lower than that of the MMT-HS. The adsorbed amount of 118.76 mg.g-1 of MMT-SP (5:1) is also higher than that of MMT-HS which is 91.8 mg.g-1, a difference of 26.96 mg.g-1.
3.3. The Effect of pH on the Removal of Copper (II) Ions
The pH of the solution was varied from 1 to 5 by the addition of 0.1 M HCl. It was found that MMT-HS and MMT-SP have almost the same sorption in the pH range 1 to 5. The optimal pH for MMT-HS and MMT-SP was 5.0. At pH higher than 5.0, copper (II) ions were precipitated due to formation of hydroxides. The effect of pH on the adsorption of Cu2+ by MMT-HS and MMT-SP is shown in Figure 3.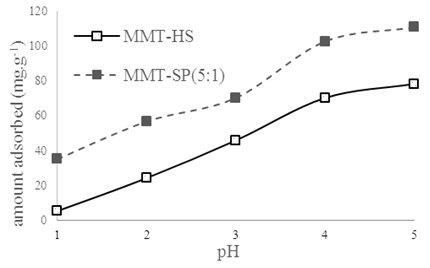 | Figure 3. Effect of pH on amount adsorbed at T = 25 °C; V = 20 mL; m(MMT-HS) = m(MMT-SP) = (0.1± 0.01)g; [Cu2+]0 = 1 g/L; t1=40 min ;t2=12 min |
3.4. The Effect of Amount of Sorbent on the Removal of Cu2+ Ions
The effect of varying MMT-HS and MMT-SP doses on the uptake Cu2+ ions from aqueous solutions was plotted in Figure 4. According to his figure, the removal efficiency and the mass of adsorbent varies in the same way; the more the mass of the clay substrate increases, the greater the adsorption.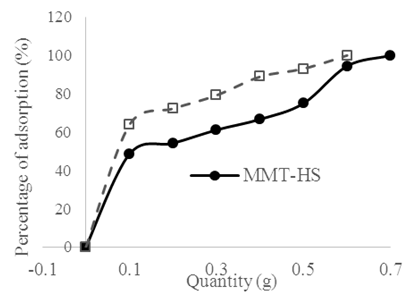 | Figure 4. Variation in the percent of adsorption Cu2+ ions versus the dose of MMT-HS and MMT-SP at T = 25 °C; V = 20 mL; m(MMT-HS) = m(MMT-SP) = (0.1± 0.01)g; [Cu2+]0 = 1 g/L; t1=40 min ; t2=12 min |
These observations are justified by the fact that the increase of the clay doses increases the availability of the fixation sites, which promotes a large adsorption and the yield accordingly (Amer et al., 2010). It is also observed that for the removal of 1 g of Cu2+ ions (ie 100% removal) from the pollutant solution, 0.7 g of homosodium clay is required as compared to 0.6 g for MMT-SP, which proves moreover, a real modification of the adsorption capacity of the crude clay.This measure also allowed us to determine, on average, the adsorption capacity gain due to the application of the surfactant. Thus, when treating a sample of homosodium montmorillonite in the proportions of 5 g of clay with 1 g of saponins, a gain of 31.50 mg of adsorbed Cu2+ ions per 1 g of adsorbent would be expected.
3.5. The Effect of Initial Metal Ion Concentration
The effect of copper (II) ions concentration on the sorption by the MMT-HS and MMT-SP was investigated by varying the metal concentration from 1 to 2 g.L-1 (Figure 5).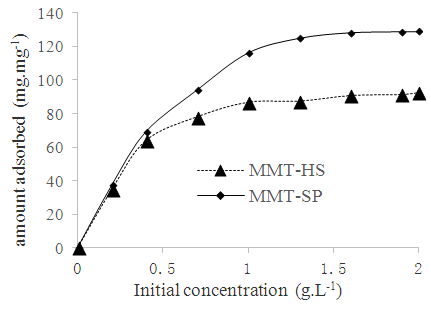 | Figure 5. Sorption isotherm of copper (II) ion on MMT-HS and MMT-SP(5:1) as a function of initial metal concentration at T = 250 °C; V = 20 mL; m(MMT-SP) = (0.1±0.01)g; and the pH of the polluting solution |
It was observed that the percent Cu2+ ions removal of the modified and crude clays increased with increasing Cu2+ concentration. Between 0.5 and 1 g.L-1, the adsorbed amount increases as a function of time, this characterizes an increasing saturation of the adsorption sites. It is obvious that for higher initial concentrations, more efficient utilization of sorption sites is expected due to a greater driving force by a higher concentration gradient. The saturation step is reached at a concentration substantially equal to 1 g.L-1 value after which the variation of the adsorbed quantity as a function of time tends to be linearized. The maximum amount of adsorption of copper ions is 91.77 mg.g-1 for MMT-HS and 129.02 mg.g-1 for MMT-SP. These results are superior to those of Ouakouak and Youcef (2016) who worked on the adsorption of Cu2+ ions on powdered activated carbon and sodium bentonite and found a value of 2.83 mg.g-1 for activated carbon and 4.58 mg.g-1 for sodium bentonite.
3.6. Isotherm Study
The isotherms obtained in figure 5 were modeled according to the Langmuir and Freundlich model and the constants of these models are summarized in Table 2. According to this table the two correlation coefficients of MMT-HS are greater than 0.90; which implies that the two models of Langmuir and Freundlich are applicable to explain the adsorption of copper on homosodium montmorillonite. Indeed, the Langmuir model provides information on the homogeneous distribution of adsorption sites, while that of Freundlich shows that the adsorbent exhibits surface heterogeneity. The Freundlich model has a correlation coefficient R2=0.99 and that of Langmuir 0.96.Table 2. Constants of Langmuir and Freundlich adsorption models 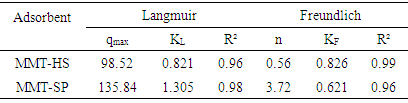 |
| |
|
The Freundlich model is therefore more appropriate to explain the adsorption of c Cu2+ ions by MMT-HS. The value of the Feundlich constant "n" of less than 1 indicates a low affinity between the MMT-HS and the Cu2+. The value of qmax shows that MMT-HS remains effective even at low concentrations.Concerning the saponins-clay, the values of the correlation coefficients reported in Table 2 show that both models are applicable to explain the adsorption of Cu2+ ions by MMT-SP. Contrary to the observations made on the isotherms of MMT-HS, Langmuir linearization not only has a coefficient of correlations closer to "1" but also gives a maximum adsorbed quantity (qmax) closer to the value experimental. An increase in Langmuir equilibrium constant is also observed when the treated substrate is passed from the untreated support. This can be explained by the more marked presence of multilayers on the surface of the adsorbent (MMT-SP) due to the saponin binding. For the modeling according to Freundlich, it is found that the correlation coefficient R2, is lower than that obtained with the MMT-HS. The Langmuir model seems to be the most adequate to explain the adsorption of copper ions by MMT-SP. The value of "n> 1" reflects a high affinity between the adsorbent and the adsorbate, and also an increase in absorption.Table 3. Thermodynamic parameters deduced from the effect of temperature on the adsorption of the two types of clay samples  |
| |
|
3.7. Effect of Temperature
The adsorption of copper on to MMT-HS and MMT-SP at different temperatures is shown in Figure 6. The adsorption of copper on to MMT-HS and MMT-SP at different temperatures showed a decrease in the adsorption capacity with increase in temperature. This indicates that the adsorption reaction was exothermic in nature. The decrease in the adsorption capacity reflects the decline of the chemical interactions between adsorbent and adsorbate. The calculations were carried out with the same temperature values which were previously converted to Kelvin. The relative adsorbed quantities obtained at different temperatures were used to calculate the important thermodynamic properties such as Gibbs ΔG° free standard energy (KJ.mol-1), standard enthalpy (ΔH°) (KJ.mol-1), and the standard entropy (ΔS°) in KJ.mol-1.K-1. | (5) |
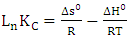 | (6) |
Where, Kc is the adsorption equilibrium constant calculated according to equation (5), R is the perfect gas constant and T is the temperature in kelvin. | (7) |
With Cd and Ce the concentrations of the Cu2+ ions on the surface of the adsorbent and in the residual solution in g.L-1. The standard enthalpy (ΔH°) and entropy (ΔS°) values have been deduced from the graph ln (Kc) as a function of 1/T which is a straight line of slope
and of the ordinate at the origin
.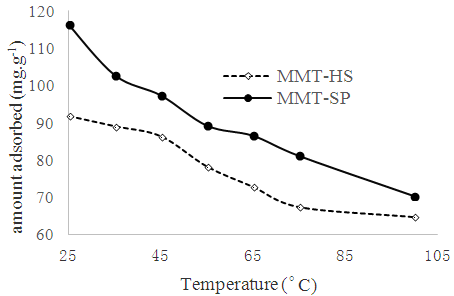 | Figure 6. Effect of temperature on the quantity of Cu2+ ions adsorbed by MMT-HS and MMT-SP |
The values of (ΔG°), (ΔH°) and (ΔS°) are shown in Table 3. The data presented above show the thermodynamic behavior of the adsorption process bonding to both types of clay. When the clay substrate is untreated, the Gibbs standard energy values remain positive, with increasing temperature. This observation indicates a disadvantaged aspect of the adsorption of the Cu2+ ions by the thermodynamic MMT-HS (Bhattacharyya and Gupta, 2011; El-Batouti et al., 2003). On the other hand, adsorption performed on MMT-SP shows negative energy adsorptions for temperatures between 25 and 100 °C involving not only low-temperature sorption spontaneity, but also a lowering of energy barriers between the adsorption on the surface of the adsorbent (Amer et al., 2010). This may be justified by the nature of the molecules used for the modification of the clay.Negative enthalpy values demonstrate that the adsorption process is exothermic. The variation of standard entropies is the measure of the disorder. This makes it possible to think that Cu2+ ions at the solid-liquid interface are more organized than that of the liquid phase. Using the value of the entropy variation, it is possible to classify the type of sorption (physical or chemical) characteristic of the phenomenon involved in this work. Thus, depending on the magnitude of the enthalpy variation, it is assumed that binding strengths <84 KJ.mol-1 are generally those of the physical type while the binding forces for chemisorption can vary from 84 to 420 KJ.mol-1. On this basis, the adsorption of Cu2+ ions on the two adsorbents is referred to as a physical process.
4. Conclusions
The general objective of this study was to evaluate the adsorptive power of a saponin-treated montmorillonite-clay, on Cu2+ ions. In order to do this, extraction of the saponins from the kernels of the plant B. aegyptiaca was carried out. The fixation of these saponins on the surface of the montmorillonite was done at different proportions. The proportion of 1 g of saponins per 5 g of clay proved to be more effective in the phenomenon of adsorption of Cu2+ ions. The adsorption of Cu2+ ions has been subjected to evaluation using several physicochemical parameters and the adsorption of Cu2+ ions by MMT-SP is faster (12 min) than on MMT-HS (40 min). The adsorption of Cu2+ ions decreases when the acidity of the medium increases in both adsorbents. The modeling of the adsorption isotherms according to Langmuir and Freundlich models has shown that these two models can be used to explain the phenomenon of adsorption of Cu2+ ions by MMT-HS and MMT-SP. The adsorption of Cu2+ ions by the saponin-treated clay is of the physical type. The maximum amount adsorbed per unit mass of the MMT-HS is 91.77 mg.g-1 and that of the MMT-SP is 129.02 mg.g-1. All this information allows to say that the MMT-SP to greater pollution power than MMT-HS.
References
[1] | Fan L., Luo C., Sun M., Qiu H., (2012). Synthesis of grapheme oxide decorated with magnetic cyclodextrin for fast chromium removal. Journal of Materials Chemistry, 22: 24577–24583. |
[2] | Selimi T., Hasani N., Sadiku1 M., Behluli M., Berisha V., (2016). Adsorption of Cu2+ and Mn2+ From Aqueous Solutions by Kosovo Clay. International Journal of Pharmaceutical Sciences Review and Research, 41(2); 58: 317-321. |
[3] | Yang Y., Wu WQ, Zhou HH, (2014). Adsorption behavior of cross linked chitosan modified by graphene oxide for Cu(II) removal. Journal of Central South University, 21: 2826–2831. |
[4] | Garcia and Miragaya J., (1977). Page A: Influence of exchangeable cation on the sorption of trace amounts of cadmium by montmorillonite. Soil Science Society of America Journal, 41:718-721. |
[5] | Li L., Luo C., Li X., (2014). Preparation of magnetic ionic liquid/chitosan/graphene oxide composite and application for water treatment. International Journal of Biological Macromolecules, 66: 172–178. |
[6] | Chaitali V. M. and Jayashree D., (2013). Review of heavy metals in drinking water and their effect on human health. International Journal of Innovative Research in Science, Engineering and Technology, 2(7): 2992-2996. |
[7] | Glatstein D. A. and Francisca F. M., (2015). Influence of pH and ionic strength on Cd, Cu and Pb removal from water by adsorption in Na–Bentonite. Applied Clay Science, 118: 61–67. |
[8] | Amini M., Younesi H., Bahramifar N. (2009). Statistical modeling and optimization of the cadmium biosorption process in an aqueous solution using Aspergillus niger. Colloids and Surfaces A: Physicochemical and Engineering, 337:67–73. |
[9] | Charerntanyarak L. (1999). Heavy metals removal by chemical coagulation and precipitation. Water Science & Technology, 39: 135–138. |
[10] | Qdais H. A. and Moussa H., (2004). Removal of heavy metals from wastewater by membrane processes: A comparative study. Desalination, 164: 105–110. |
[11] | Alyüz B. and Veli S., (2009). Kinetics and equilibrium studies for the removal of nickel and zinc from aqueous solutions by ion exchange resins. Journal of Hazardous Materials, 167: 482–488. |
[12] | Ahluwalia S. S. and Goyal D., (2007). Microbial and plant derived biomass for removal of heavy metals from wastewater. Bioresource Technology, 98: 2243–2257. |
[13] | Kurko S.V. and Matovic, L.L. (2015). Simultaneous removal of Pb2+, Cu2+, Zn2+ and Cd2+ from highly acidic solutions using mechanochemically synthesized montmorillonite–kaolinite/TiO2 composite. Applied Clay Science, 103:20–27. |
[14] | Ghazy S., Ragab A. H., (2007). Removal of copper from water samples by sorption onto powdered limestone. Indian Journal of Chemical Technology, 14: 507-514. |
[15] | Ouakouak A., Youcef L., (2016). Adsorption of Cu2+ Ions on Powder Activated Carbon And A Sodique Bentonite. Larhyss Journal, 2: 39-61. |
[16] | Noudem J. A., Nguemtchouin M. G.M., Kaptso K. G., Noumi G. B. (2017). Saponins-Clay Modified Materials: A New Approach Against Callosobruchus subinnotatus In Stored Products. International Journal of Scientific and Technology Research, 6: 134-141. |
[17] | Abdallah, E. M.; Anis, B. H.; Al-Khalifa, S. K. 2012. Antimicrobial, antioxidant and phytochemical investigation of Balanites aegyptiaca (L.) Del. edible fruit from Sudan. African Journal of Biotechnology, 11(52): 11535-11542. |
[18] | Amer M. W., Khalili F. I., Awwad A. M.,(2010).Adsorption of lead, zinc and cadmium ions on polyphosphate-modified kaolinite clay. Journal of Environmental Chemistry and Ecotoxicology, 2(1): 001-008. |
[19] | Bhattacharyya K.G., and Gupta S.S., (2011). Removal of Cu(II) by natural and acid-activated clays: An insight of adsorption isotherm, kinetic and thermodynamics. Desalination, 272:66-75. |
[20] | El-Batouti M., Sadek O. M., Assaad F. F. (2003). Kinetics and thermodynamics studies of copper exchange on Na–montmorillonite clay mineral. Journal of Colloid and Interface Science, 259:223-227. |