Khalil Abid, Afnan Abd-Almonuim
Chemistry Department, College of Science, University of Al – Mustansiriyah, Baghdad, Iraq
Correspondence to: Afnan Abd-Almonuim, Chemistry Department, College of Science, University of Al – Mustansiriyah, Baghdad, Iraq.
Email: |  |
Copyright © 2018 Scientific & Academic Publishing. All Rights Reserved.
This work is licensed under the Creative Commons Attribution International License (CC BY).
http://creativecommons.org/licenses/by/4.0/

Abstract
New mesogen Schiff base ligand 1,3-bis-[(4-butyloxy-benzylidene)-amino]-propan-2-ol [L] was synthesized by the reaction of substituted 4-butyloxy benzaldehydeand 1,3-diamino-2-propanol in 2:1 Molar ratio. Four mononuclear lanthanide complexes with (Ln = La(III), Ce(IV), Nd(III) and Gd(III)) were synthesized and characterized by 1HNMR, Fourier transform infrared (FT-IR) spectroscopy, UV – vis, mass spectroscopy, elemental analysis (C.H.N), magnetic susceptibility and molar conductivity measurements. Thermal properties of the title compounds were studied using the thermogravimetric analysis/differential scanning calorimetry (TGA/DSC) and polarizing optical microscopy (POM), the ligand and coordination compounds exhibit liquid crystalline properties.
Keywords:
Schiff base, Liquid crystals, Lanthanide complexes, Thermotropic phase
Cite this paper: Khalil Abid, Afnan Abd-Almonuim, Synthesis, Structural Characterization, and Liquid Crystal Properties of Lanthanide Complexes with Schiff Base Ligand 1,3-bis-[(4-butyloxy-benzylidene)-amino]-propan-2-ol, American Journal of Chemistry, Vol. 8 No. 1, 2018, pp. 19-28. doi: 10.5923/j.chemistry.20180801.04.
1. Introduction
The metal containing liquid crystals, named "metallomesogens", combines the physical characteristics exhibited by metal coordination complexes with those of mesogenic molecules forming the significant materials [1]. It is well-known that the liquid crystals with transition-metal core group, called metallomesogens, have attracted increasing attention because of the stability the physico-chemical properties of the metal, i.e., the color, magnetic moments, reduction-oxidation systems and the polarizability which are all the framework with organic part [2].The lanthanide-containing metallomesogens (lanthanidomesogens) are the well-known class of luminescent metallosogens [3]. The emission resulted from these materials is caused by transferring energy levels with the 4f shell of La(III) ion. The significance of fluorescence of lanthanide complexes that involves liquid crystalline properties can be utilized in high coloric materials due to the range of wavelengths of emission spectra [4]. Detailed studies by liquid crystal researchers have led to empirical rules, one of which includes the effect of the chemical constitution in the formation of nematic and smectic mesophases [5]. Most of these studies focused largely on Schiff’s bases since the discovery of 4-methoxybenzylidene-4’- butylaniline, which exhibited a nematic phase at room temperature. The subject of mesogenic properties of lanthanide(III) complexes possessing long chains of alkoxy –OR encouraged many researches to study their structures and liquid crystals properties, and one of them was Pawan, R. et. al [6] who prepared poly dentate Schiff bases, by condensation of 2,6-diaminopyridine with 4-alkoxy-2-hydroxybenzaldehyde in acidic medium and all the lanthanide (III) complexes were isolated in tetrahydrofuran (THF) as inert solvent. On basic and spectroscopic investigations of lanthanide metal complexes of a chain of mesogenic alkoxy Schiff-base subordinates, this paper reports the results of examination on the synthesis, spectroscopic investigations and thermal conduct of the mesogenic Schiff-base, 1,3-bis-[(4-butyloxybenzylidene)- amino]-propan-2-ol [L] and the relating lanthanide complexes.
2. Materials and Methods
All chemicals were analytical grade and used without any modification. 1HNMR spectra were obtained using a Bruker 500 MHz and d6 –DMSO as a solvent at University of Al Albeit, Amman, Jordan. FTIR spectra were recorded as KBr disc using a Shimadzu 8400 FTIR spectrophotometer in the range 4000–400 cm−1. Elemental analysis (C.H.N.S) for the ligand and metal complexes were carried out on EuroEA-Element Analyzer model 3000. Mass spectra were recorded by electron impact mass spectrometry (EIMS) using a Direct Injection Probe on a Shimadzu GCMS – QP5050A Spectrometer, Electronic spectra were recorded by using (Varin Cary 100 Conc. UV–Vis) spectrophotometer in the range (200–800nm), Molar conductivity of the complexes were measured in DMF as a solvent in 0.001M solutions using a CON 510 bench conductivity meter with 2-ring stainless steel conductivity electrode (cell constant, K = 1.0) were carried out at the Chemistry Department, College of Science, Mustansiriyah University, Baghdad, Iraq. Magnetic susceptibility measurements were carried out using the Faraday method with balance magnetic susceptibility model MSB-MKI. Thermal stability (weight changes) of the samples was recorded by Mettler Toledo TGA851 in the temperature up to 600°C at College of Education of Pure Science Ibn-Alhaithum, University of Baghdad, Iraq. The phase transition temperatures determined by using a differential scanning calorimetry (DSC) thermograms in different ranges were carried out at (R.T.) (heating and cooling rate = 5°C/min) using (Linseis STA.PT-1000). POM was carried out on a microscope model Leica DM2500 equipped with a hot-stage system of Linkam THMSE-600.
3. Experimental
3.1. Preparation of 4-n-butyloxy Benzaldehyde
Equimolar amounts of 4-hydroxy benzaldehyde (7.5 mmol, 9.15 g), 1-bromobutane (7.5mmol, 10 gm) and potassium hydroxide (7.5 mmol, 4.2 gm) were added to a solution of absolute ethanol (100 mL). The mixture was refluxed for 48h and filtered while hot to remove the insoluble solids. Dilute sodium hydroxide (10%) was added to the filtrate until the point of neutralization and the product was then extracted twice with CHCl3 (100 mL portions). The chloroform extract was concentrated by evaporation and cooled to obtain a white solid, in 73 – 85% yield. It was further purified by recrystallisation using alcohol. See Scheme (1).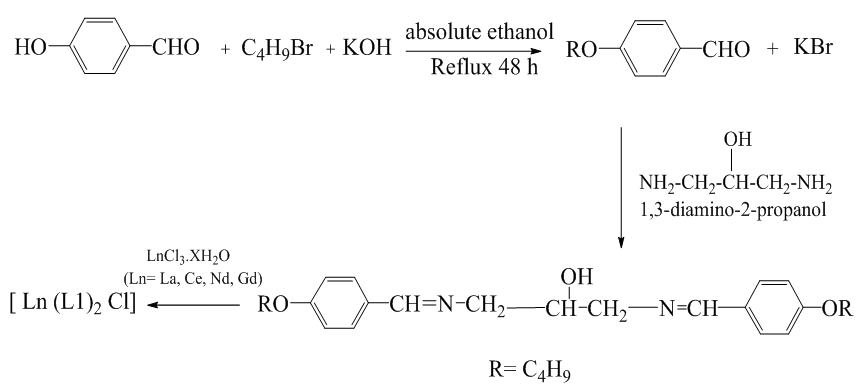 | Scheme 1. Synthesis of the ligand L and its lanthanide complexes |
3.2. Synthesis of 1,3-bis-[(4-butyloxy-benzylidene)-amino] -propan-2-ol [L]
An ethanolic solutions of (2 mmol, 1.78gm in 30 mL) 4-butyloxybenzaldehyde and (1 mmol, 0.90gm, in 20 mL) of 1,3-diamino-2-propanol were refluxed for 6 h in presence of a few drops of acetic acid as catalyst. The precipitate was filtered off and recrystallized from (ethanol), yield: 85%; m.p: 74–75°C. Element analysis for C25H34N2O3, M. wt. 410; calculated (%)C 73.14, H 8.35, N 6.82; found (%) C (74.51); H (8.05); N (6.94).
3.3. Synthesis of the Lanthanide Complexes
To a stirred solution of (2 mmol) of the ligand [L] in ethanol (40ml) and (1mmol) of the LnCl3.XH2O (Ln = LaCl3.7H2O, CeCl3.7H2O, NdCl3.6H2O, and GdCl3.6H2O) in ethanol (30ml) were mixed under stirring the resultant solution turned cloudy after
15 min; stirring and reflux were continued for 8h. The precipitate was filtered off, washed with cold ethanol and dried in vacuum oven for 3h at 70°C.
4. Results and Discussions
4.1. Infrared Spectra
The spectra were recorded in the region 4000-400cm-1 using KBr disc. It be seen that the vibrational mode of both υ(C=N), υ(O−H) of the ligand had been affected in somewhat by the long chain of alkoxy -OR in intensity and small vibrational frequency shift were observed. Thus, the vibrational mode υ(O-H) and υ(C=N) groups at 3674cm-1 and 1656cm-1 respectively [7]. The absorption bands for ν(Ar-C–H,), ν(C–H, aliphatic) and ν(C=C, aromatic) have observed were recorded around 3070, 2955-2872 and 1508 cm-1 respectively. The FT-IR spectra of lanthanum(III) complexes formed with such ligands showed some shifts in υ(C=N) to a range (1689-1681cm-1) supporting the binding of lanthanum(III) ions with nitrogen atoms of imine moiety [8]. The broad band around 3674 cm-1 belonged to –O-H group have suffered to lowering in the wavenumbers in all IR spectra of lanthanum(III) complexes due to the coordination of hydroxyl group with the metal ion and this remarkable change was shown in the range (3570-3248 cm-1), Furthermore, the weak to medium bands around (528-415 cm-1) could be assigned to the coordination bonds of ν Ln–N,ν Ln–O [9]. The results obtained from FT-IR spectra of complexes confirms the tridentate behavior of NON-donor system.
4.2. 1HNMR Spectra
1HNMR spectra were recorded using d6-DMSO as solvent and TMS was used as an internal standard. Chemical shifts were reported in parts per million (ppm) and coupling constants were given in Hertz (Hz). The 1HNMR spectra of ligand exhibits singlet peak at 8.23 ppm which is attributed to the resonance of –CH=N- of symmetric structure labeled at 4,4-, figure (5). The multiple peaks in the range (6.97-7.68) ppm could be assigned to the aromatic Ar-H labeled at 5,5-/8,8- confirming the 1,4-disubstituted phenyl group [7]. As well as the resonance peaks of –OH1, -CH2- aliphatic –CH2-CH2- protons were observed around (3.53, 3.72) and (0.96-1.74) ppm confirming the condensation of the diamino alcohol starting material via –NH2 functional group with the carbonyl HC=O of 4-alkoxy benzaldehyde derivative then agree well with the other analysis of C.H.N and FT-IR spectra to confirm the structure of the Schiff base ligand. The remarkable shift of –CH=N- and –OH resonance protons to the regions (8.62) ppm and 4.028 ppm suggesting the coordinating of oxygen and nitrogen atoms of hydroxyl and imine with the empty orbitals of the La(III) ion [10, 11], Figure (7).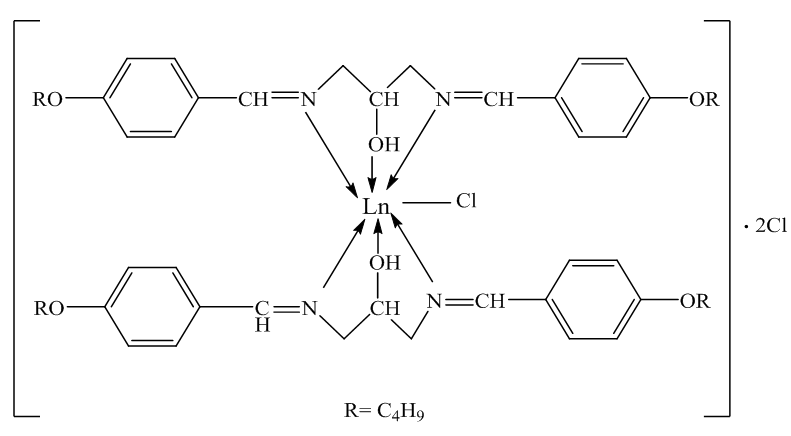 | Figure (1). Proposed coordination in lanthanide complexes |
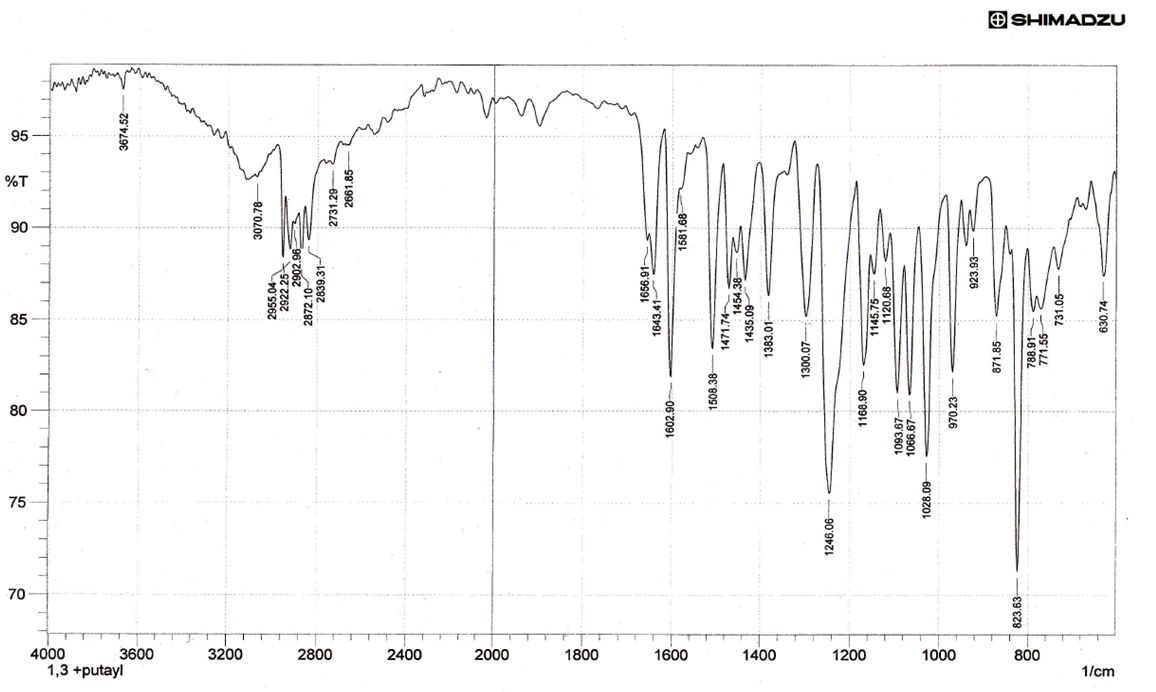 | Figure (2). FT-IR for L |
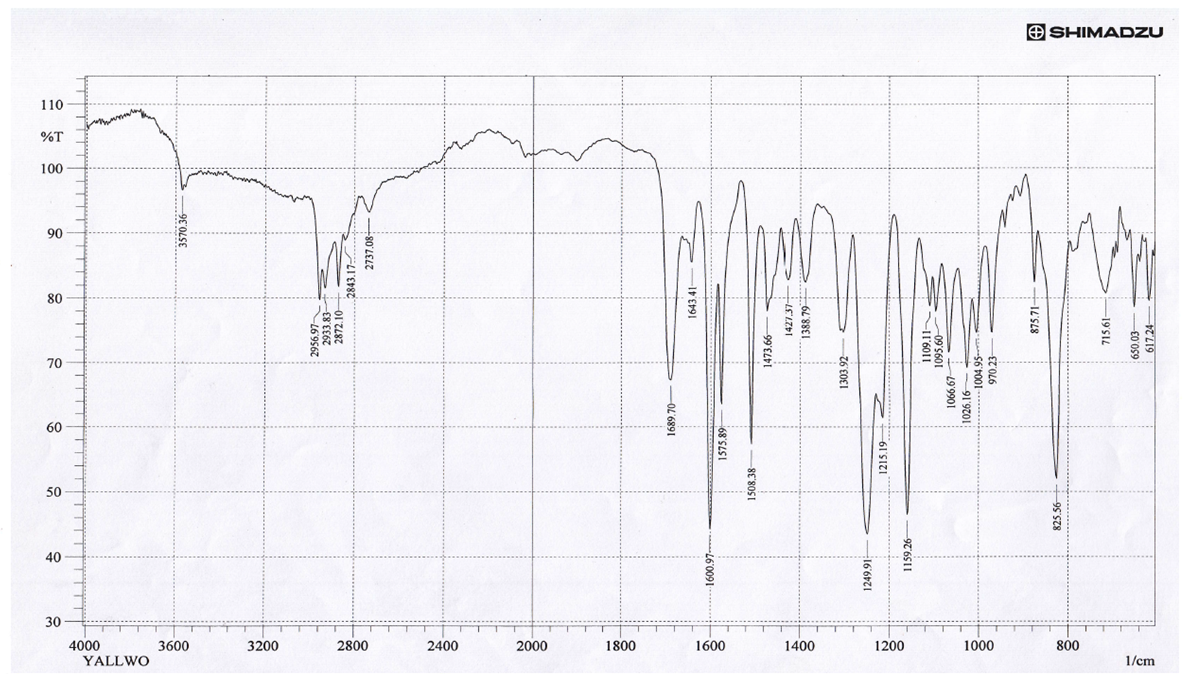 | Figure (3). FT-IR for LLa(III) |
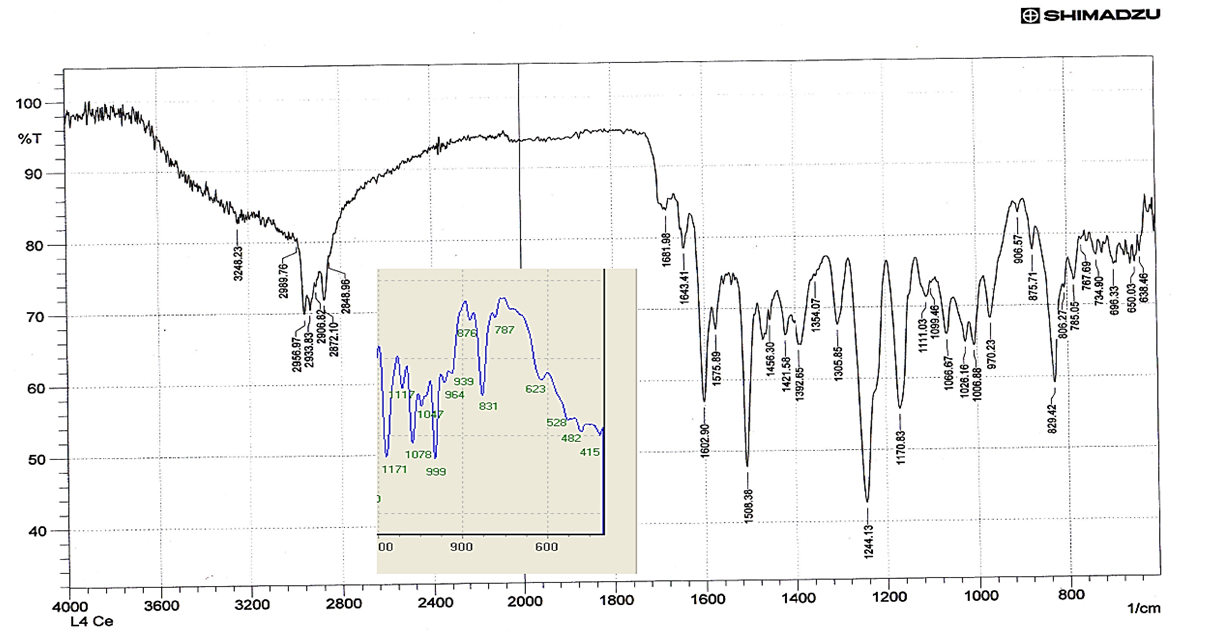 | Figure (4). FT-IR for LCe(IV) |
 | Figure (5). 1HNMR label atoms of the ligand L |
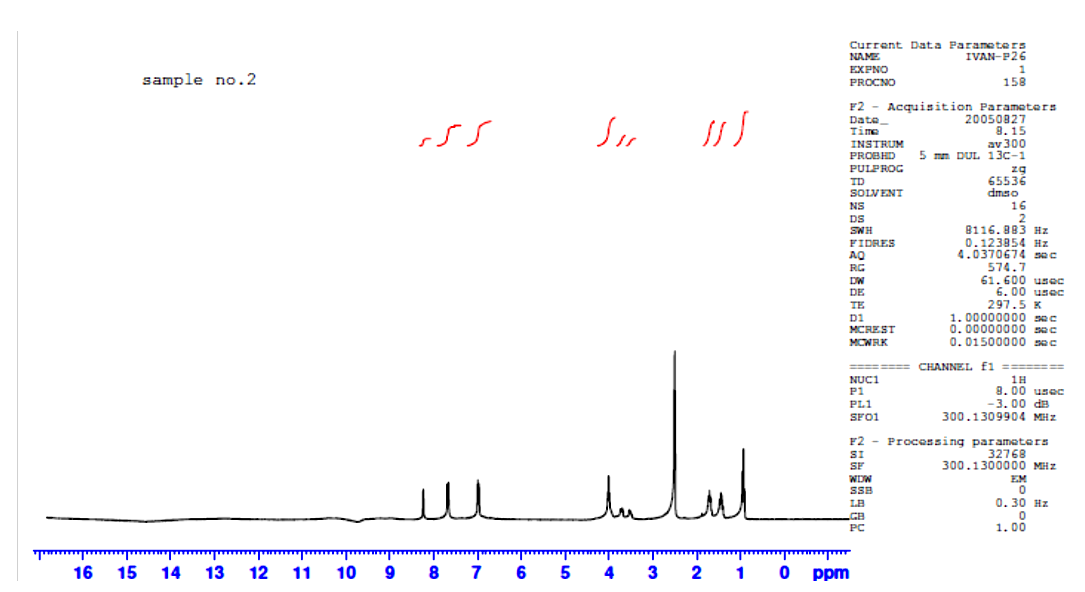 | Figure (6). 1HNMR spectrum of L |
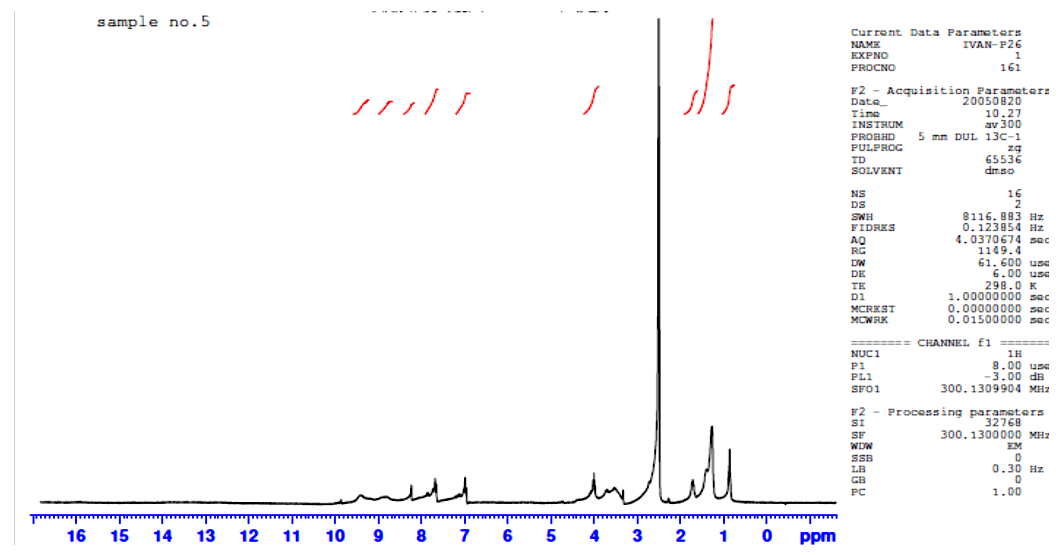 | Figure (7). 1HMNR for La(III) complex |
4.3. Mass Spectra
The mass spectral of the ligand as illustrated in figure (8). Was described by molecular ion peak at m/e = 410 which confirm the molecular weight m/e value of 410 that agree with the empirical formula of the ligand, (C25H34N2O3). The intensity was 100% for the base peak 220 was estimated for the cleavage of [C4H9OC6H4CH=NCH2]+. Several peaks were observed in the mass spectrum at m/e [fragment, intensity %] 260 [m/e= C4H9OC6H4, 10], 235 [C4H9OC6H4CH = N, 40], 191[m/e= C4H9OC6H4CH = NCH2CH-O, 80].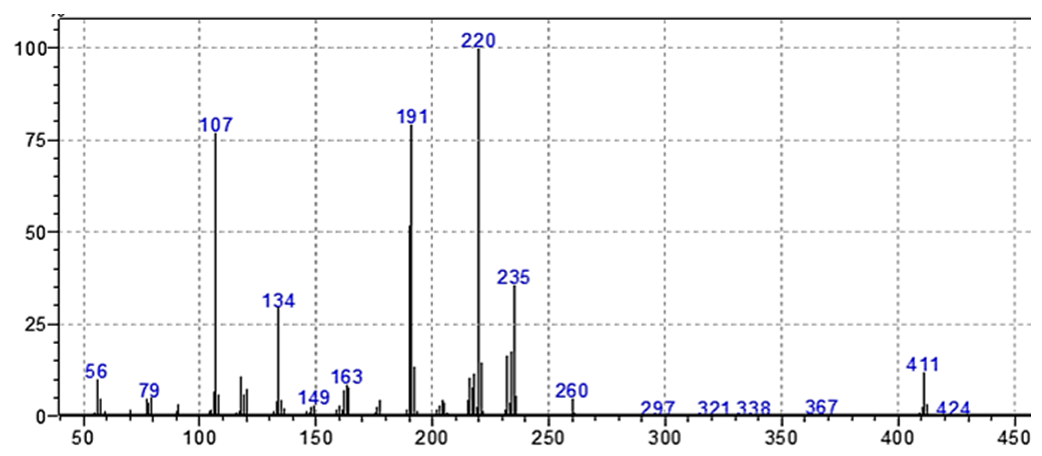 | Figure (8). Mass spectrum of L |
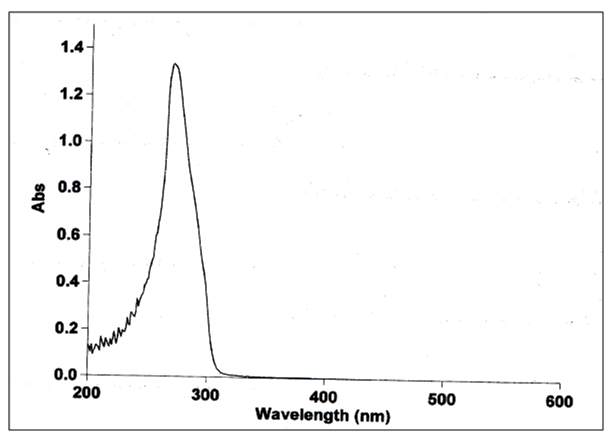 | Figure (9). uv-visble for L |
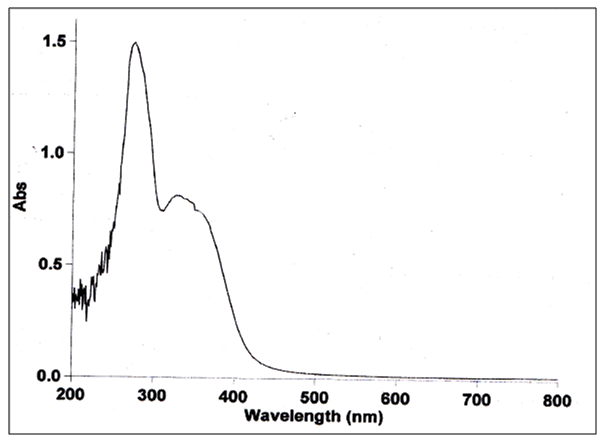 | Figure (10). uv-visble for LNd(III) complex |
4.4. UV-Visible Spectra
The UV-Visible spectra of L showed absorption bands at (241- 269) nm (41493 – 37174) cm-1 these bands could be attributed to the (π–π*) and (n– π*) transitions of C=C aromatic and C=N respectively. The Nd(III) complex showed absorption band at 330nm 30,303cm-1 assigning to LMCT transitions respectively then confirms the complexation of the ligand with the empty orbitals of the Nd(III) ion [12].
4.5. Magnetic Measurements
The f-block elements of lanthanides have special magnetic properties due to the presence of many odd electrons in their valence orbitals. The magnetic susceptibility for the complexes was recorded in the solid state at 298 K using Faraday’s method. As an important source of magnetism, the magnetic properties of rare earth ions are well known and are dominated by the internal nature of the f orbital different from the 3d orbitals of transition metal ions in a ligand field [13]. The 4f electrons are responsible for the strong magnetism exhibited by the metals and compounds of the lanthanides. In the incomplete 4f subshell the magnetic effects of the different electrons do not cancel out each other as they do in a completed sub shell, and this factor gives rise to the interesting magnetic behavior of these elements. At higher temperatures, all the lanthanides except lutetium are paramagnetic (weakly magnetic), and this paramagnetic frequently shows a strong anisotropy.They also show a strong anisotropy in their magnetic behavior depending on the crystal direction. Study of the magnetism of rare-earth elements has had great influence on present-day theories of magnetism. The maximum number of unpaired electrons is 7, in Gd+3, with a magnetic moment of 7.94 B.M., but the largest magnetic moments, at 10.4–10.7 B.M., are exhibited by Dy+3 and Ho+3. However, in Gd+3 all the electrons have parallel spin and this property is important for the use of gadolinium complexes as contrast reagent in MRI scans [14]. The table (3) shows the magnetic moments in Bohr Magneton units for all the prepared complexes where the La(III) and Ce(IV) complexes with ligand exhibit diamagnetic properties since no odd electrons found in the valence shell of orbital [15]. The complexes of Nd(III) showed paramagnetic properties due to the presence of three unpaired electrons in 4f orbitals with a magnetic moment range (3.1-4.1) BM. As well as the Gd(III) complexes showed parallel spin characters with magnetic moments in the region (7.6-8.6) BM respectively indicating the 4f7 configuration then supports the high coordination number of C.N=7 with equilibrium environments of mono capped trigonal prism geometry around the ion of Gd(III) complexes [16].
4.6. Molar Conductivity Measurements
The molar conductance of 10-3M concentrations of all complexes in DMF solvent were done in order to assist us in the elucidation the formula and structures of the prepared lanthanide complexes. The data observed for molar conductance of lanthanide complexes formed with L showed a non-electrolyte for La(III) complexes except the Ce(IV), Nd(III), Gd(III) with ligand which have molar conductivity values of 77.6 Ω-1cm2mol-1 for LCe(IV) assigning to the presence of chloride ion as a counter ion in the structures. While the LNd(III), LGd(III), complexes showed molar conductivity values in the range (143.2-137.6) Ω-1cm2mol-1 which agrees well with the electrolytic properties of 2:1 ratio due to the presence of two Cl-1 in the outer-sphere of complexes structures [17], Table (3).Table (1). Physical properties of L and its metal complexes 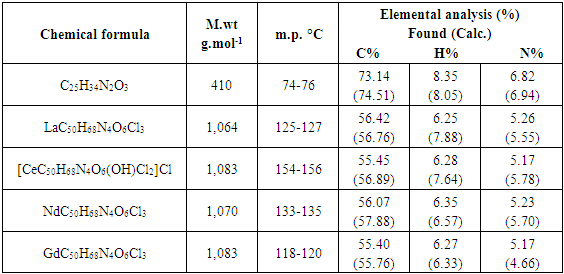 |
| |
|
Table (2). Major infrared absorption bands of L and its lanthanide complexes (cm−1) 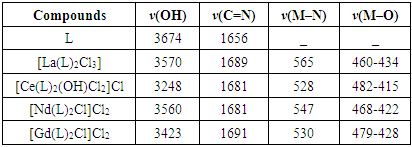 |
| |
|
Table (3). Magnetic moment and molar conductivity data of L complexes 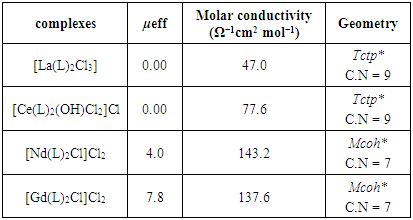 |
| |
|
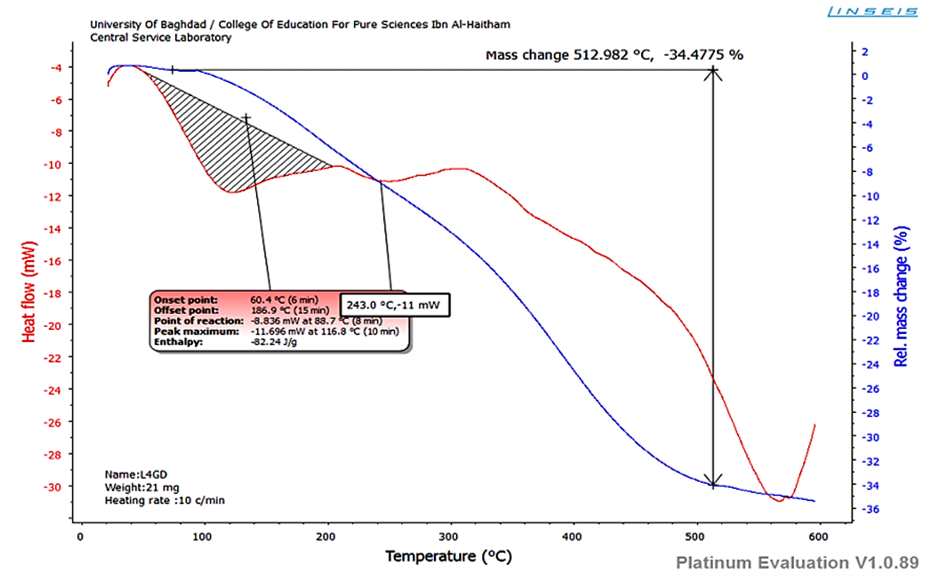 | Figure (11). TGA of LGd (III) complex |
4.7. Thermogravimetric Analyses
Thermogravimetric analyses (weight changes) were performed for complexes in the temperature range from room temperature up to 600°C using oxygen gas at the heating rate of 20°C/min. The thermal behavior of Gd(III) complex figure revealed that the complex is stable up to 500°C and do not show any weight loss below this temperature. It is strong evidence, which represent that the complex is devoid of lattice water as well coordinated water in the coordination sphere. The main mass loss recorded up to 513°C indicated the loose of organic species and the formation of a thermally stable metal oxide [18, 19].
4.8. Liquid Crystal Properties of Lanthanide Complexes
The mesomorphic behavior of the compounds was probed by polarized optical microscopy (POM) and differential scanning calorimetry (DSC). The ligand exhibits mesophase while the complexes showed monotropic phase only during heating. This ligand exhibits SmA phases during heating to isotropic liquid (I) and the reverse cooling process to crystalline solid (Cr). Phase transitions was observed at 70°C (Cr-SmA) and 86°C (SmA-I) (Figure 12). The enthalpy changes for this transition was -52.73 kJ/mol, on the reverse process, phase transitions happened at 65.3°C (I-SmA) and 57.6°C (SmA-Cr). The enthalpies for this transition was 41.54 kJ/mol.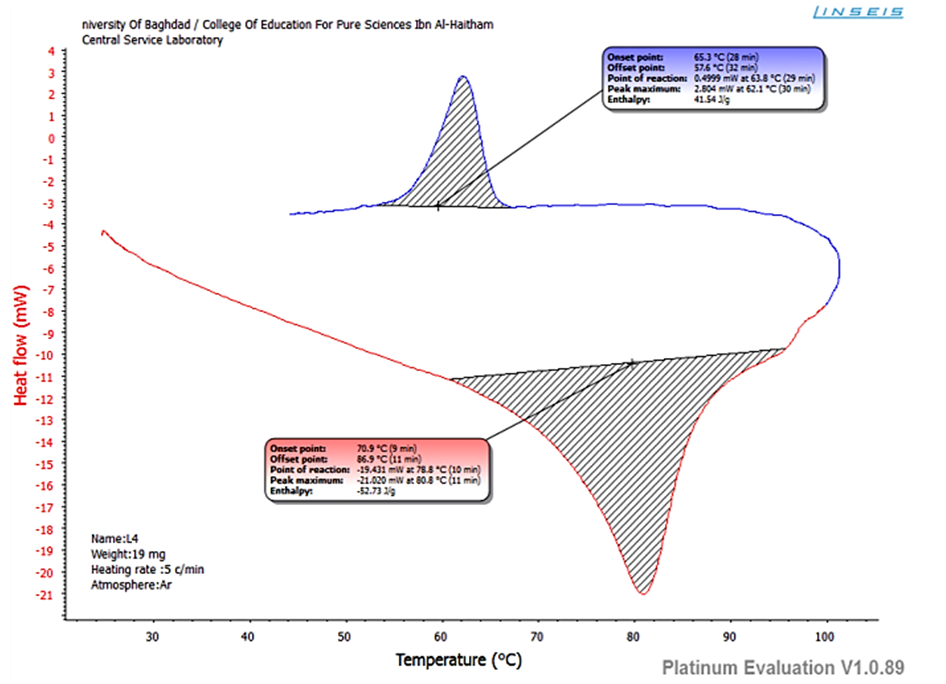 | Figure (12). DSC for L |
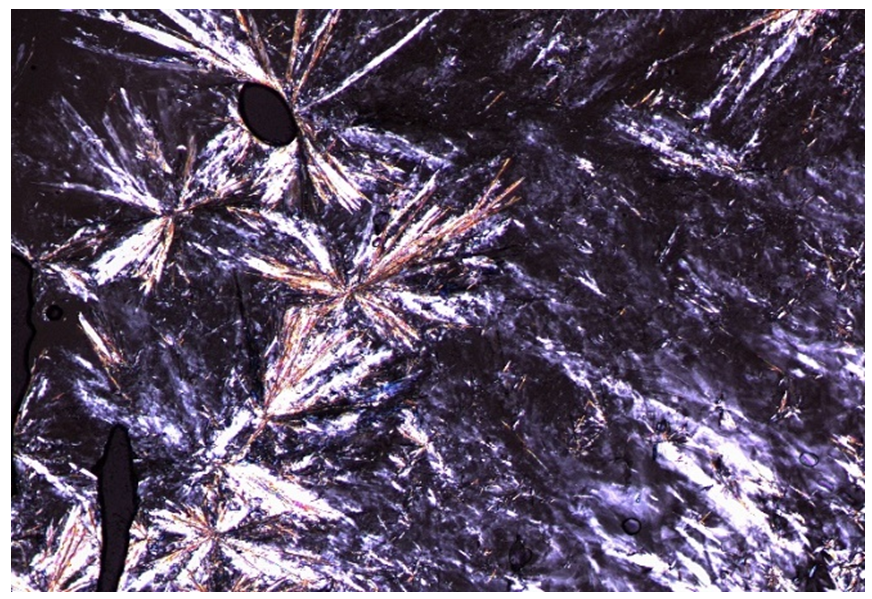 | Figure (13). Optical photomicrograph of L of SmA phase on heating to 80°C |
Lanthanide(III) complexes exhibit monotropic phase. Therefore, DSC analysis was done for the complexes to determine their phase transition temperature and transition enthalpies value (ΔH). Gd (III) complex exhibit SmA phases during heating only. Phase transitions were observed at 75°C (Cr-SmA) and 158°C (SmA-I) (Figure 14). The enthalpy changes for those transitions were -4.95 kJ/mol.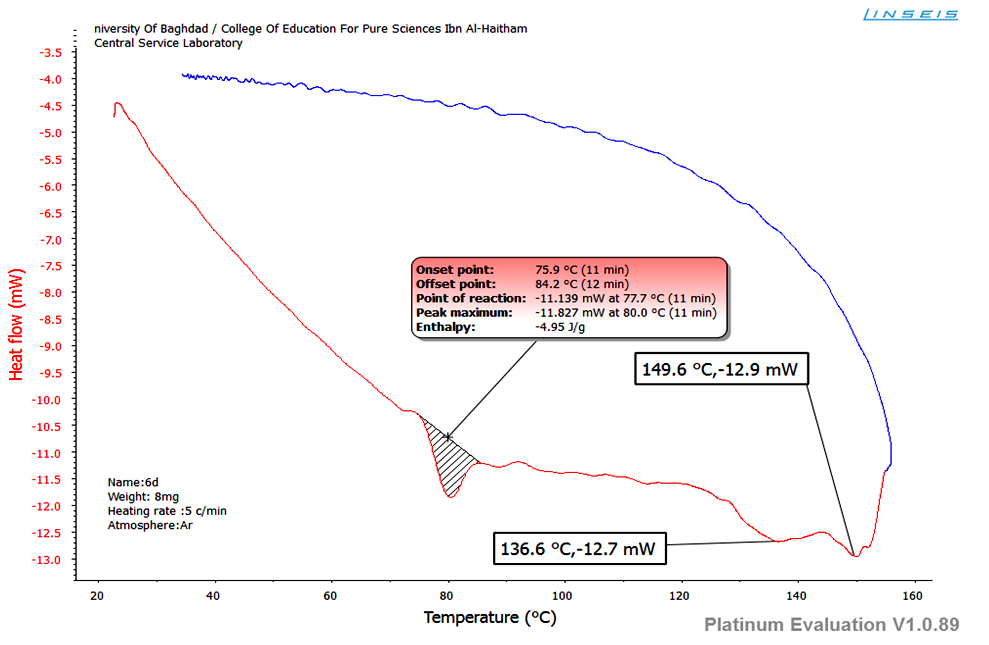 | Figure (14). DSC for LGd(III) |
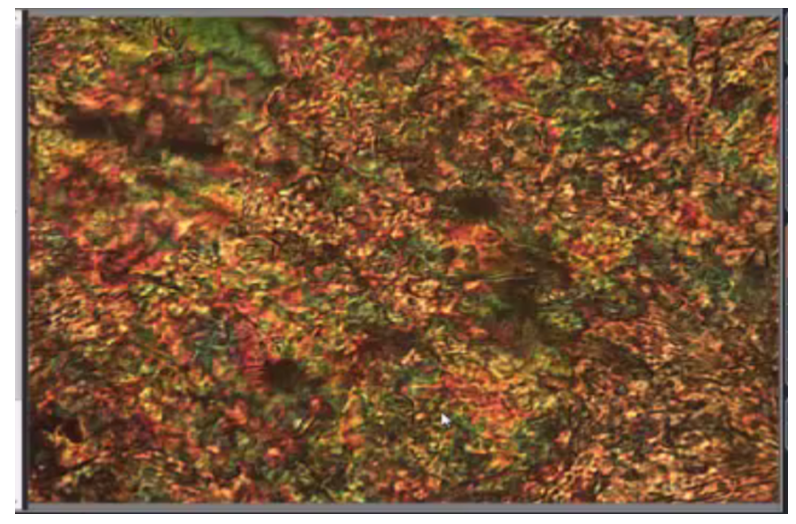 | Figure (15). Optical photomicrograph of LGd(III) of SmA phase on heating to 85°C |
5. Conclusions
The Schiff-base 1,3-bis-[(4-butyloxy-benzylidene)-amino] -propan-2-ol [L] and its lanthanide complexes were synthesized and characterized. Investigations of the collected results are consistent with the stoichiometry as two forms of ligands exist in the complexes: tridentate through one hydroxyl group and two azomethine nitrogen to complete seven coordination geometry. All the complexes show thermotropic properties except Ce(IV) complex. No water molecules present inside coordination sphere or outside as the complexes where stable up to 500°C.
ACKNOWLEDGMENTS
This work was supported by the department of chemistry, College of Science, University of Al – Mustansiriyah, Baghdad, Iraq. The author, therefore acknowledge with thanks for technical.
References
[1] | Chakraborty, L., et. al., (2012) Liquid Crystals, 39(5), 655. |
[2] | Binnemans, K., (2010) Molecular Materials, 61. |
[3] | Cardinaels. T, Driesen. K, Parac- Vogt. T. N, Heinrich. B, Bourgogne. C, Guillon. D, Donnio. B & Binnemans. K,; (2006) Chem. Mater., 17(2), 6589. |
[4] | Knyazev. A.A, Galyametdinov. Y.G.; Goderis. B.; Driesen. K.; Goossens. K.; Gorller-Walrand. C, Binnemans. K and Cardinaels. T.; (2008) Eur. J. Inorg. Chem, 16(3), 756. |
[5] | Gray, G.W, "Molecular Structure and the Properties of Liquid Crystals"; (1964), Academic Press, London,: 76 (17), 68. |
[6] | Pawan. R. S, Angad. K. S, and Rao. T.R, (2012); Materials Science and Engineering; 32(5); 1906. |
[7] | Khouba. Z, Benabdallah. T, and Maschke. U, (2009); Mol. Cryst. Liq. Cryst., 502, 121. |
[8] | Abid K. K. and Al-barody S. M.; (2014) Liquid Crystals; 41(9), 1303. |
[9] | Nakamoto K., (1970) “Infrared Spectra of Inorganic and Coordination Compounds”, 2nd ed. Wiley Interscience: New York. |
[10] | Ziyad A. Tahaa, et. al.,. (2011), Spectrochimica Acta Part A: Molecular and Biomolecular, 81: 570. |
[11] | Vigato, P. A., & Tamburini, S. (2004). Coordination Chemistry Reviews, 248, 1717. |
[12] | Binnemans, K., & Gündogan, B. (2002). Journal of Rare Earths, 20, 249. |
[13] | Benelli, C., & Gatteschi, D. (2002). Chemical Reviews, 102, 2369. |
[14] | Bunzli, J. C. G. (2006), Accounts of Chemical Research, 39, 53. |
[15] | Miller, J. S., & Drillon, M. (Eds.). (2006). "Magnetism: Molecules to materials IV". Weinheim: Wiley. |
[16] | Tang, K., Zhang, J. J., Zhang, D. H., Ren, N., Yan, L. Z., and Li, Y. (2013). The Journal of Chemical Thermodynamics, 56, 38. |
[17] | Greenwood, N. N., & Earnshaw, A. (2012). "Chemistry of the elements." Oxford: Butterworth-Heinemann Elsevier. |
[18] | Cifelli, M., Domenici, V., and Veracini, C. A. (2013). Current Opinion in Colloid & Interface Science, 18, 190. |
[19] | Hu, J. M., Zhang, J. Q., & Cao, C. N. (2003), Thermochimica Acta, 403, 257. |