Enos Masheija Rwantale Kiremire
Department of Chemistry and Biochemistry, University of Namibia, Windhoek, Namibia
Correspondence to: Enos Masheija Rwantale Kiremire, Department of Chemistry and Biochemistry, University of Namibia, Windhoek, Namibia.
Email: |  |
Copyright © 2016 Scientific & Academic Publishing. All Rights Reserved.
This work is licensed under the Creative Commons Attribution International License (CC BY).
http://creativecommons.org/licenses/by/4.0/

Abstract
Gold forms clusters with some of the p-block elements. The clusters have spectacularly interesting shapes. This paper applies the series method to examine selected golden clusters in which golden fragments are bound to carbon, nitrogen and oxygen and some of their corresponding analogues. Nearly all the clusters analyzed have been found to be capped with nuclear centers comprising of two skeletal elements. The cluster identity numbers k, were calculated using the newly introduced skeletal numbers. The cluster k values so obtained were transformed into series and correlated to those of the corresponding osmium series. The background to the use of skeletal numbers to generate cluster series has been highlighted to enable the readers be able to easily follow the analysis of gold-main group element clusters.
Keywords:
Skeletal-numbers, Series, Golden-clusters, Isolobal, Capping, Linkages, Valence, Stripping
Cite this paper: Enos Masheija Rwantale Kiremire, Clusters of Gold Containing p-Block Elements, American Journal of Chemistry, Vol. 6 No. 5, 2016, pp. 126-144. doi: 10.5923/j.chemistry.20160605.03.
1. Introduction
Gold forms a wide variety of clusters with unique skeletal architectural designs which have attracted a lot of attention [1-4]. Some have been classified as torroidal and others as spherical in shape [3]. It has recently been found that the golden clusters could be analyzed and categorized with the help of the series method [5]. This paper attempts to examine the golden clusters which have the small atoms, C, N, P, O, and S atoms of the p-block main group elements [1, 6].
2. Results and Discussion
2.1. Background
2.1.1. Skeletal Numbers and Skeletal Shapes
The series method has introduced the use of skeletal linkage numbers of the transition metal atoms and the main group elements [7] to categorize clusters. These have been reproduced for ease of reference and popularization of their usage. They are provided in Tables 1 and 2.Table 1. Skeletal Numbers (k) of the Transition Elements 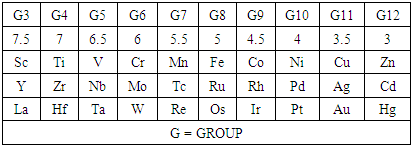 |
| |
|
Table 2. Skeletal Numbers (k) of the Main Group Elements 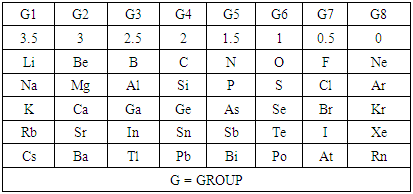 |
| |
|
The skeletal linkage values of elements are extremely useful as they assist in rapid categorization and prediction of the shapes of a wide range of clusters [7]. Let us take the osmium (Os) skeletal element as an illustration. It has been assigned a skeletal linkage value of five (k = 5) as shown in Table 1. When the osmium atom combines with a two electron donor such: CO, to form a complex [Os(CO)1] one skeletal linkage value is utilized by the CO ligand according to series method [7]. If we regard the skeletal linkage values of Os atom as being deposited in its ‘basket’, then the removal of 1 linkage value from the basket leaves 4 in the basket. If we take the sum of what the ligand has removed from the basket and what remained in the basket we get 1+4 = 5 which is the original skeletal linkage value of the ‘naked’ Os element. If another CO ligand is added to the osmium atom, we get [Os(CO)2] fragment. This means that 2 skeletal linkage values of osmium will be taken away from the basket, and 3 will be left over. Again, the sum of the skeletal linkage values consumed and those left over in the basket = 2+3 = 5, the original skeletal linkage content value of osmium. When five CO ligands are added to produce, [Os(CO)5] complex, all the skeletal linkage values have been consumed and its basket is, in principle, now empty. But still the sum 5+0 = 5 holds true. When k = 0 for Os(CO)5 mono-skeletal cluster, the 18 electron rule is obeyed. If the naked metallic cluster consists of 2 osmium atoms, then the Os2, will initially have 5+5 = 10 skeletal linkage values in its basket. When the carbonyl ligands are successively added until there 9 CO ligands altogether are added to Os2 cluster to form Os2(CO)9, then the cluster will have a value of k = 1. This means that the two osmium skeletal atoms in the cluster will, in principle, be linked by 1 skeletal bond. That is, Os―Os bond. In the case of Os3(CO)12 cluster, k = 15-12 = 3. Therefore, the three Os skeletal elements will be linked by 3 bonds which usually gives rise to a triangular skeletal shape S-1. For Os4 (CO)16, k = 4(5)-16 = 4. A characteristic skeletal shape is expected to be a square, S-2. In the same way, Os4 (CO)14, k = 20-14 = 6 (tetrahedral), Os5(CO)16, k =9 (trigonal bipyramid) and Os6(CO)182―, k =11(octahedral). The corresponding ideal skeletal shapes are shown in S-3, S-4, and S-5.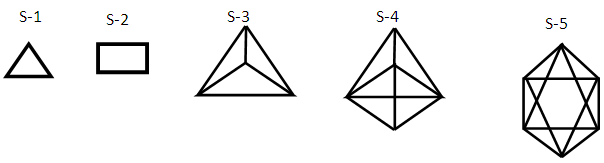
2.1.2. Possible Mechanism
The possible mechanism for the formation of cluster fragments for Os1 and Os2 with a carbonyl ligand is summarized in Schemes 1 and 2 and also Table 3Table 3. Illustration of Conservation of Skeletal Linkages Principle 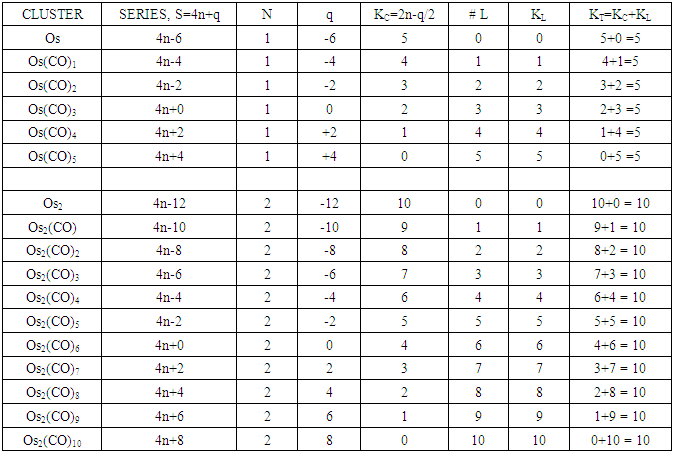 |
| |
|
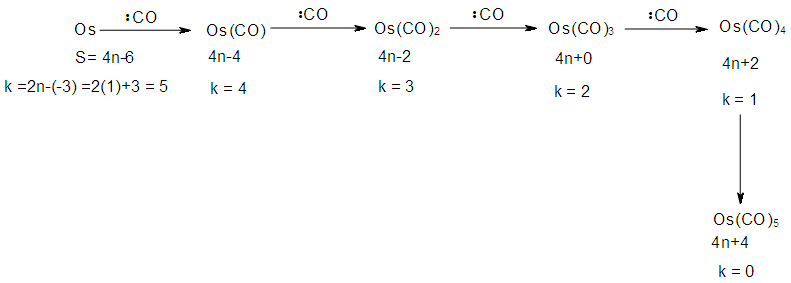 | Scheme 1. Formation of carbonyl fragments of mono-skeletal osmium |
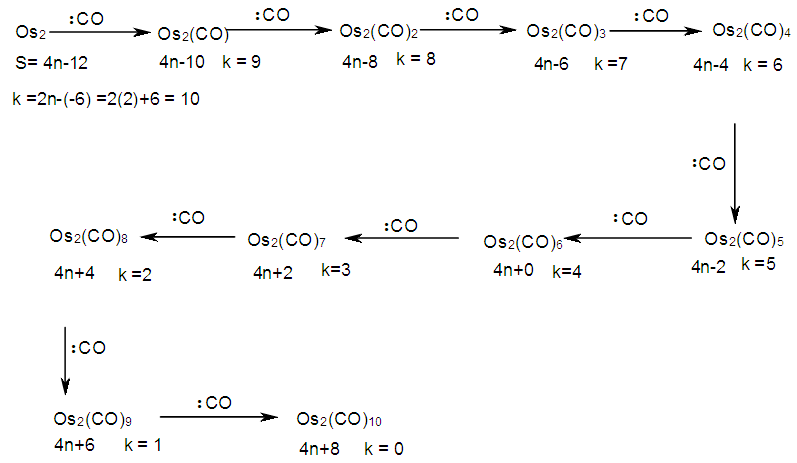 | Scheme 2. Formation of carbonyl fragments of bi-skeletal osmium |
2.1.3. Conservation of Skeletal Linkage Principle
The conservation of skeletal linkages principle follows directly from the mechanism. The sum of the linkages used up by the ligands and the linkages remaining with the skeletal atom(s) are equal to the original number of skeletal linkages supplied by the naked skeletal elements. THIS IS THE BASIS OF THE CONSERVATION OF SKELETAL LINKAGE PRINCIPLE [7]. The conservation of skeletal linkage principal is illustrated for Os1 and Os2 skeletal fragment series in Table 3. The changes in k value for Os1, Os2, Os3 to Os10 when CO ligands are added are shown in Table 4. The formulas of the known transition metal carbonyls conform to the concepts of skeletal linkage values mentioned above. Selected known carbonyl clusters or their equivalents can be identified from the Table 4 are given in Table 5. This underpins the strength of skeletal linkage value concept.
2.1.4. Generating Series with Skeletal Numbers of Naked Skeletal Elements
Table 4. Generating formulas of cluster series 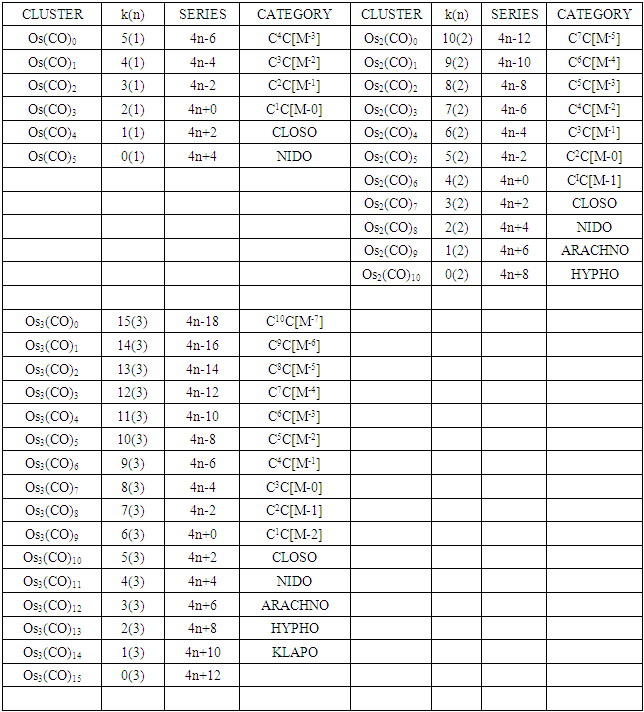 |
| |
|
Table 4. Continued 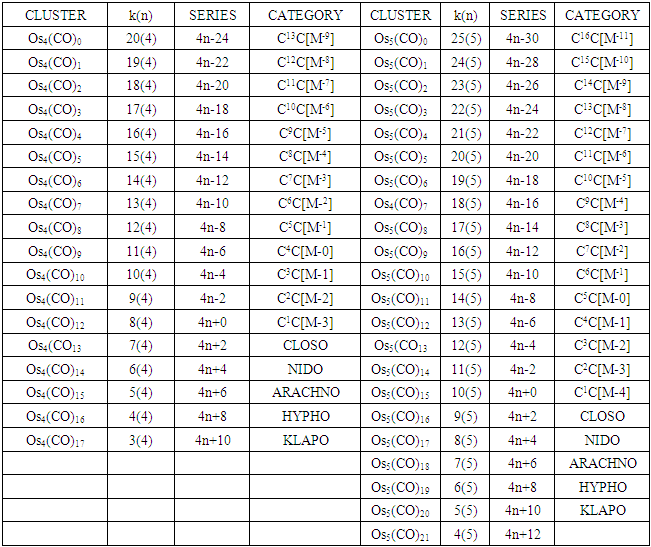 |
| |
|
Table 4. Continued 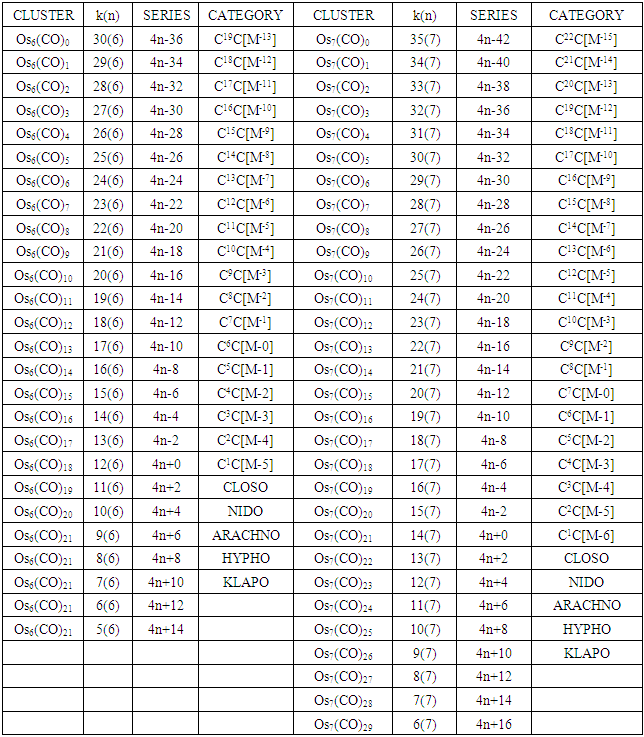 |
| |
|
Table 4. Continued 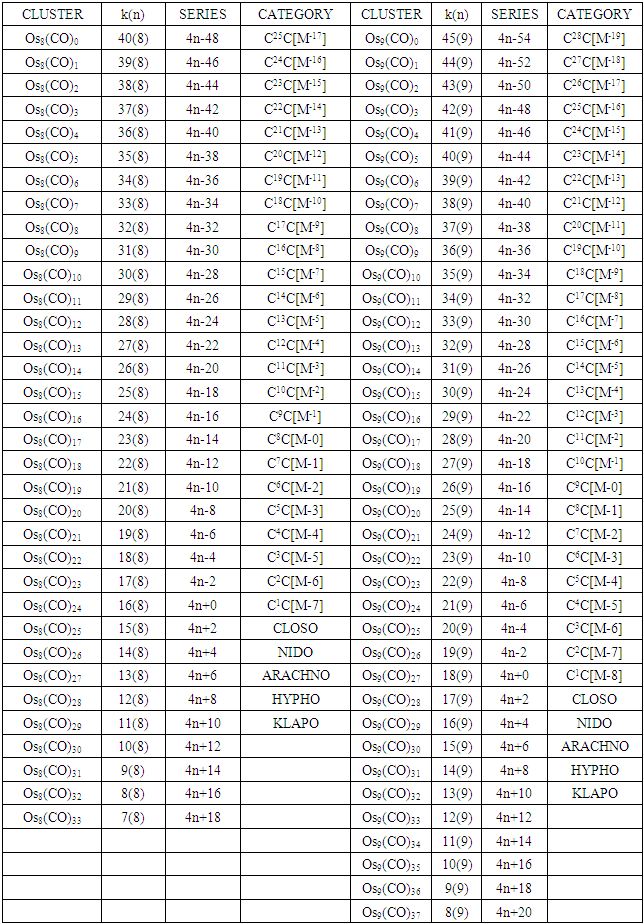 |
| |
|
Table 4. Continued 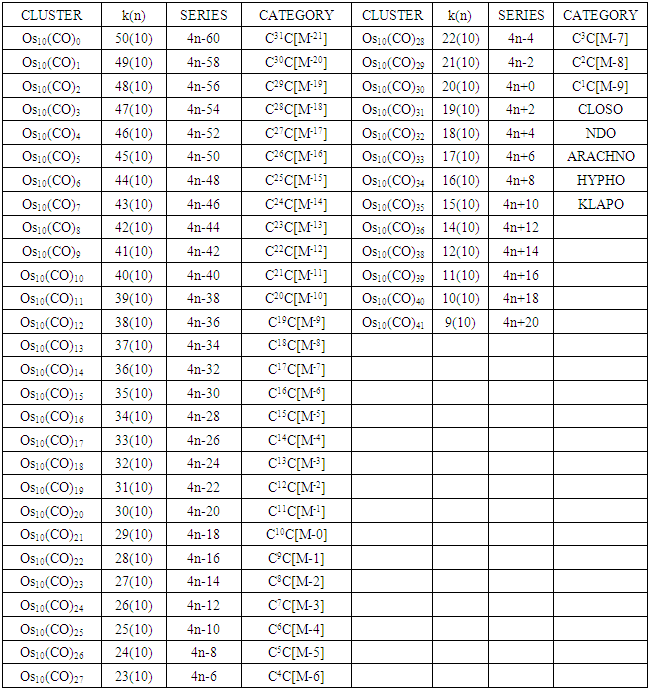 |
| |
|
2.1.5. Table 5
Table 5. Selected known osmium carbonyls 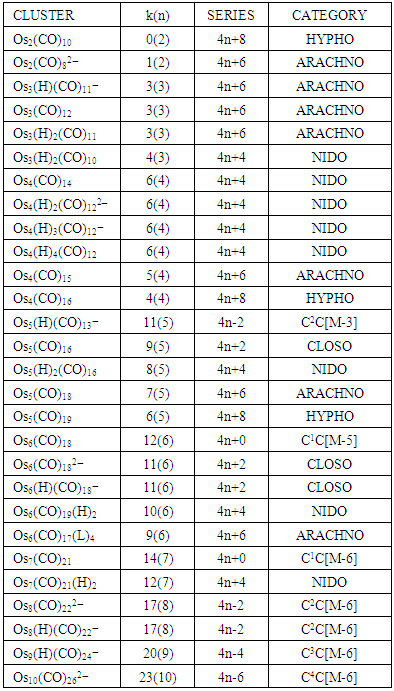 |
| |
|
2.1.6. Important Conclusions from Table 4
The construction of Table 3 summarizes 4 FUNDAMENTAL concepts about clusters. These are: The assigning of skeletal linkage values to skeletal elements in Table 1-3. The conservation of the total linkage values when ligands are added to naked skeletal elements. The influence of the skeletal cluster linkages (k) on the final symmetry of the cluster. The use of capping symbol CnC that has been developed and adequately explained in earlier work [8].
2.1.6.1. The Cluster Symmetry as a Function of k(n) Parameter
The k(n) parameter has a major influence on the type of shape a cluster will adopt. This is demonstrated by the tetrahedral symmetry of different clusters with the same k(n) value in Table 6.Table 6. Selected Tetrahedral Clusters that conform to k(n) = 6(4) 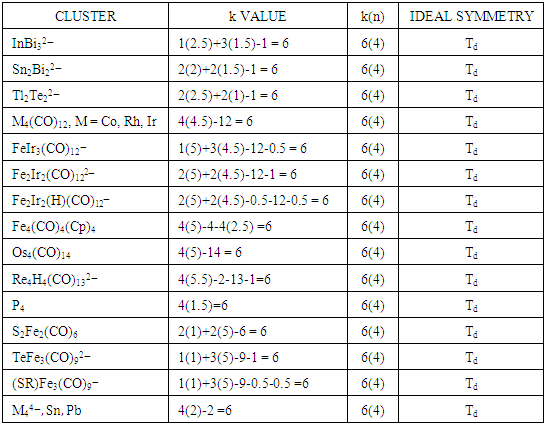 |
| |
|
Table 6 summarizes a very important principle. That is, if different clusters have the same skeletal k and n values, [k(n) the same], then clusters are very likely to adopt the same shape. Table 6 has been used to demonstrate the tetrahedral symmetry, but the same can be done for square pyramid, k(n) = 8(5), trigonal bipyramid, k(n) = 9(5), octahedral, k(n) = 11(6), mono-capped octahedral, k(n) = 14(7), bi-capped octahedral, k(n) = 17(8), and so on.
2.1.7. The Application of Skeletal Numbers to Test the 8 and 18- Electron Rules
The application of the 8 and 18-electron rules test is simple. If the k value of the centered skeletal element is equal to zero, then that skeletal element obeys the corresponding rule. For example in CH4, k = 1(2)-4(0.5) = 0. This means that each hydrogen ligand removes 0.5 skeletal linkage value from the carbon central atom. The number of skeletal linkages removed are 2. Since the carbon atom has k = 2, then all the linkage values have been utilized by H ligands and k = 0 for the carbon atom. Hence, it obeys the 8 electron rule. The same approach is applied to Fe(CO)5, k = 1(5)-5(1) = 0. Therefore Fe obeys the 18-electron rule. The Mn2(CO)10 complex, each Mn skeletal element is tested separately. Thus, k = 1(5.5)-5(1)-1(0.5) = 0. In this case, the 5 CO ligands and 1 Mn-Mn bond are taken into consideration. Hence, each Mn skeletal element obeys the 18-electron rule. The same treatment was applied to the Re4(CO)162― and Sn[Fe(CO)4]4 complexes. This information is summarized in Scheme 3.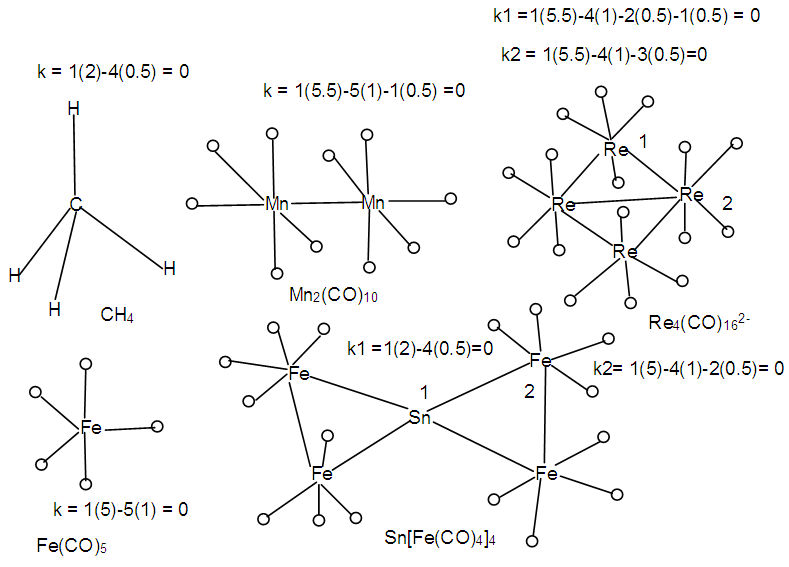 | Scheme 3. Examples to illustrate the use of skeletal numbers to test 8 and 18 electron rules |
2.1.8. Isolobal Relationship
The combination of one or more osmium skeletal elements with the CO ligands produce chemical fragments as shown in Schemes 1 and 2 and Tables 3 and 6. Their formation follows chemical series. Using the series, it is possible to predict the shapes of a limited number of those fragments. For instance, Os(CO)5 is expected to have a trigonal bipyramid shape whereas Os2(CO)9 is expected to have a single Os-Os bond with one or more bridging CO ligands. We know that both Os(CO)5 and Os2(CO)9 do obey the 18-electron rules. However, the series method for now can not be used to determine how stable the fragments are. On the other hand, the series method is extremely useful as a guide identify those fragments which are isolobal [14, 15] based upon the equivalence relationship in k values. For example CH3(k =2-1.5 =0.5) and Mn(CO)5[k = 5.5-5 = 0.5]. Therefore, CH3
Mn(CO)5, and CH2(k = 2-1 = 2) and Fe(CO)4[k =5-4 = 1] and hence, CH2
Fe(CO)4. Likewise, CH(k =2-0.5 = 1.5) and Rh(CO)3[k = 4.5-3 =1.5] and the two fragments are isolobal, CH
Rh(CO)3. Table 7 has shows selected number of isolobal fragments. These are classified as follows: Os
(Fe, Ru); Os(CO)
(Ni, Pd, Pt); Os(CO)2
(Zn, Cd, Hg); Os(CO)3
(C, Si, Ge, Sn, Pb); Os(CO)4
(CH2, CR2); Os(CO)5
(CH4, CR4); Co(CO)3
(CH,CR); Mn(CO)5
CR3
Fe(Cp)(CO)2; Fe2(CO)4(Cp)2
Mn2(CO)10; Fe2(CO)8(CR2)
Rh3(Cp)3(CO)3
Rh2(Cp)2(CO)2(CR2)
Fe3(CO)12; Si2R4
Rh2(Cp)2(CO)2
Fe2(CO)8; C4R4
C3R3Co(CO)3
C2R2Co2(CO)6
(CR)Co3(CO)9
Co4(CO)12; Re3(CO)12(SnR2)―
Re2(CO)8C2R4
Re4(CO)162―; AuL
(B, Al, Ga, In, Tl); AuX
(C, Si, Ge, Sn, Pb).Table 7. Illustration of Isolabal Fragments 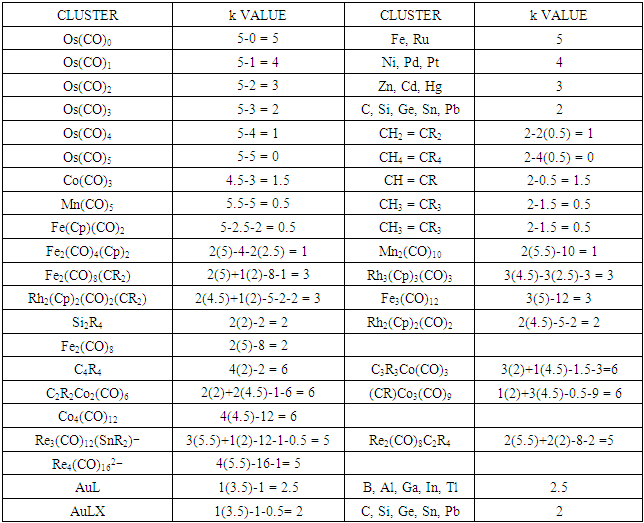 |
| |
|
2.2. Series and Golden Clusters
2.2.1. Categorization of Golden Clusters into Series: Worked out Examples and Interpretations
Ex-1 Au8L72+, L = PR3k = 8(3.5)-7+1 = 22, k(n) = 22(8)
Os8(CO)18; q = 2[ 2n-k] = 16-22] = 2[-6], S = 4n-12, Cp = C1C+C6C = C7C[M-1]. According to the series, this means the golden cluster will have one (1) nuclear skeletal gold atom surrounded by 7 other capping skeletal golden atoms. However, the series do not allow us to know how the 7 capped skeletal atoms will be arranged around the nuclear atom. A sketch of the graphical representation of the cluster is shown in S-6.
Indeed in this cluster is found to have one skeletal atom in the nucleus surrounded by 7 others [9, 10].Ex-2 Au9L83+k =9(3.5)-8+1.5 = 25, k(n) = 25(9)
Os9(CO)20 (from Table 3); S = 4n-14, Cp = C8C[M-1]. This means the cluster has one skeletal atom at the nucleus which is surrounded by 8 capping skeletal atoms. This is what is observed. As stated in Ex-1, the series cannot tell us how the 8 capping atoms are arranged. A graphical representation sketch is shown in S-7.
Ex-3 Au10Cl3L6+K = 10(3.5)-3(0.5)-6+0.5 = 28, K(n) = 28(10)
Os10(CO)22 (from Table 3), S =4n-16, Cp = C9C[M-1]. Again here we have a golden cluster with one atom at the nucleus surrounded by 9 capping atoms. This is what is found [9]. This graphically represented as in S-8.
Ex-4. Au8L82+, L = PPh3k = 8(3.5)-8+1 = 21, k(n) = 21(8), q = 2[2n-k] = 2[16-21] = 2[-5], S =4n-10, Cp = C1C+C5C = C6C[M-2]. The symbol means that 6 skeletal atoms will be capped around 2 nuclear atoms. A simple graph of the skeletal sketch of what is expected is shown in S-9 and what is observed [4, 8] in S-10. The skeletal atoms labeled 1 and 2 in S-10 may be associated with [M-2]. The two nuclear atoms according to series obey the closo series S = 4n+2, k =2n-1 = 2(2)-1 = 3. Ideally, this implies that the two skeletal atoms could be linked by a triple bond.
N-1. Pt(AuL)82+
n = 1+8 = 9, k(n) = 25(9)
Os9(CO)20;q = 2[2n-k] = [2(9)-25] = 2[-7]S = 4n-14; Cp = C1+C7 = C8C[M-1]. The Pt skeletal element was found to be in the middle of the cluster [1].
N-2. Pt(CO)(AuL)82+
K(n) = 24(9)
Os9(CO)21;q = 2[2n-k]= 2[2(9)-24]= 2[18-24] = 2[-6]; S = 4n-12, Cp = C1+C6 = C7C[M-2]. In this case, 2 gold skeletal elements occupied the middle position [1].
N-3. (AuL)6Re(CO)3+
K(n) = 18(7)
Os7(CO)17; q = 2[2n-k] = 2[2(7)-18] = 2[14-18]=2[-4], S = 4n-8,C1+C4 = C5C[M-2]. This is in agreement with the literature [9]. The atoms labeled 1 and 2 represent [M-2]. The other 5 skeletal elements surround the [M-2] nucleus.
N-4. Os7(CO)21k = 7[5]-21 = 14; k(n) = 14(7); q =2[2n-k] = 2[2(7)-14] = 2[14-14] =0; S = 4n+0, C1C[M-6]; mono-capped octahedral complex, V = 14n+0 = 14(7)+0 = 98. This agrees with what is reported [11].
N-5. Re8(C)(CO)242―k = 8[5.5]-2-24-1 = 17; k(n) = 17(8)
Os8(CO)23;q = 2[2(8)-17]=2[-1]; S = 4n-2, Cp = C2C[M-6]→Bi-capped octahedral complex [10], V = 14n-2 = 14(8)-2 = 110.
N-6. Pd23(CO)20L10, L = PEt3k = 23(4)-20-10 = 62, k(n) = 62(23), q = 2[2n-k] = 2[46-62] = 2[-16], Cp = C1+C16 = C17C[M-6]. This means, the cluster is expected to have an octahedral nuclear inner center.This is simply diagrammatically represented as in F-6.
N-7. C(AuL)4k = 1(2)+4(2.5) = 12, n = 1+4 = 5, k(n) = 12(5)
Os5(CO13; q = 2[2n-12] = 2[2(5)-12] = 2[-2], S = 4n-4, Cp = C3C[M-2]. The cluster is expected to have 3 capping skeletal elements and this is what is observed with the lower Au skeletal atoms capping the two C-Au skeletal atoms [1, 6].
N-8. C(AuL)5+k =1(2)+5(2.5)+0.5 =15, n = 1+5 = 6, k(n) = 15(6)
Os6(CO)15; q = 2[2n-k] = 2[2(6)-15] = 2[-3], S = 4n-6 = C4C[M-2]. V = 4n-6 = 4(6)-6 = 18, adjustment for transition metal elements, V = 18+10(5) =68. This is the same as V = 4+(11+2)(5)-1 = 68, calculated from the formula of the carbon-golden cluster. The shape is in agreement with the literature [1, 6].
k1 = 1(2.5)-5(0.5) = 0, 8 electron rule obeyed. In this case, the central atom is regarded as C+(k = 2+0.5 =2.5).k2 = 1(3.5)-1(1)-5(0.5) = 3.5-1-2.5 = 3.5-3.5 = 0, 18 electron rule is obeyed.k2 = k3 = k4, all the corresponding skeletal atoms obey the 18 electron rule.k5 = 1(3.5)-1(1)-4(0.5) = 3.5-1-2 = 3.5-3 = 0.5. The skeletal atom is short of one electron in order to obey the 18 electron rule, 18-1 =17. It has 17 valence electrons.k6 = k5 and hence does not obey the 18 electron rule. The shape is in agreement with the literature [1, 6].N-9. C(AuL)62+
k = 1(2)+6(2.5)+1 = 18, n = 1+6 = 7,k(n) = 18(7)
Os7(CO)17; q = 2[2n-k] = 2[2(7)-18] = 2[-4], S = 4n-8, Cp = C5C[M-2]. V = 4n-8 = 4(7)-8 = 20. Adjustment for metal skeletal elements, V = 20+10(6) = 80. Calculation from the formula, V = 4+6(13)-2 = 80. The shape is in agreement with the literature [1, 6].N-10. N(AuL)4+k = 1(1.5)+4(2.5)+0.5 = 12, n = 1+4 = 5, k(n) = 12(5)
Os5(CO13; q = 2[2n-12] = 2[2(5)-12] = 2[-2], S = 4n-4, Cp = C3C[M-2]. The ideal skeletal shape will be similar to that in F-7 except C skeletal element will be replaced by N atom.N-11. N(AuL)52+k = 1(1.5)+5(2.5)+1 = 15, n = 1+5 = 6, k(n) = 15(6)
Os6(CO)15; q = 2[2n-k] = 2[2(6)-15] = 2[-3], S = 4n-6 = C4C[M-2]. V = 4n-6 = 4(6)-6 = 18, adjustment for transition metal elements, V = 18+10(5) =68. This is the same as V = 4+(11+2)(5)-1 = 68, calculated from the formula of the nitrogen-golden cluster. The ideal skeletal shape expected to be as in F-8 with N substituting C.N-12. P(AuL)4+K = 1(1.5)+4(2.5)+0.5 = 12, n = 1+4 = 5, K(n) = 12(5)
Os5(CO13; q = 2[2n-12] = 2[2(5)-12] = 2[-2], S = 4n-4, Cp = C3C[M-2]. The ideal skeletal shape will be similar to that in F-7 except C skeletal element will be replaced by P atom.N-13. P(AuL)52+k = 1(1.5)+5(2.5)+1 = 15, n = 1+5 = 6, k(n) = 15(6)
Os6(CO)15; q = 2[2n-k] = 2[2(6)-15] = 2[-3], S = 4n-6 = C4C[M-2]. V = 4n-6 = 4(6)-6 = 18, adjustment for transition metal elements, V = 18+10(5) =68. This is the same as V = 4+(11+2)(5)-1 = 68, calculated from the formula of the nitrogen-golden cluster. The ideal skeletal shape expected to be as in F-8 [1, 6] with P substituting C. However, what is observed is as sketched in F-10.
Clearly, the skeletal shape F-10 is different from that of F-8. This is probably due to what we may call “capping isomerism”.N-14. (PR)(AuL)42+k = 1(1.5)+4(2.5)+1-0.5 = 12, n =1+4 = 5, K(n) = 12(5)
Os5(CO13; q = 2[2n-12] = 2[2(5)-12] = 2[-2], S = 4n-4, Cp = C3C[M-2]. The ideal skeletal shape will be similar to that in F-7 except C skeletal element will be replaced by P atom. However, the observed skeletal symmetry is as sketched in F-11 [1, 6]. In this case, C3C[M-2], the capping skeletal elements and the nuclear elements [M-2] face the same direction. This also can be regarded as a form of “capping isomerism”.
N-15. O(AuL)42+k= 1(1)+4(2.5)+1 = 12,n =5, k(n) = 12(5)
Os5(CO13, q = 2[2n-12] = 2[2(5)-12] = 2[-2], S = 4n-4, Cp = C3C[M-2]. The skeletal shape is expected to be similar to that observed in F-7. Indeed, this is what is observed with O replacing C atom [1, 6].N-16. (SMe)(AuL)32+
k = 1(1)+3(2.5)+1-0.5 = 9, n = 1+3 = 4, k(n) = 9(4)
Os4(CO)11; q = 2[2n-k] = 2[8-9]=2[-1], C1C+C1C = C2C[M-2]. This means that 2 skeletal atoms are capping on a nucleus of two others. A graphical representation is given in the sketch F-12 and what is observed is given in the skeletal sketch F-13 [1, 6].The symbol [M-2] means that the 2 nuclear skeletal elements belong to the closo series S = 4n+2 with k = 2n-1. If n = 2, then k = 2n-1 = 2(2)-1 = 3. Ideally this implies that the 2 nuclear skeletal elements are linked by a triple bond. If the S atom is one of the skeletal nuclear atoms them we could visualize one of the possible skeletal structure to be as given in F-14.
Is it be possible then that such a triple bond could be delocalized within the skeletal structure resulting in the S-Au bonds being shorter than the normal single bonds?
2.2.2. Skeletal Linkage k(n) as a Concept
A skeletal atom can be considered to possess a certain number of intrinsic skeletal linkage values which are kept in its ‘pocket or basket’. When a 2 electron ligand donor comes and binds to the skeletal element, it removes 1 skeletal linkage values from its basket. Hence, the basket content will have 1 less than before. Let us illustrate this with osmium skeletal element. As can be seen from Table 1, osmium (Os) is assigned the K value of 5, that is k(n) = 5(1). If a :CO ligand comes and gets bound to the Os skeletal element to form [Os(CO)] cluster, one of the k values will be removed from the basket, leaving 4 behind, that is, k(n) = 4(1). If the process continues until 5(:CO) ligands have been bound to the Os skeletal element to form [Os(CO)5] cluster, then all the 5 k values will be removed from the basket, and Os skeletal element will have 0 linkage values, that is, K(n) = 0(1). The entire process is summarized at the beginning of Table 3. The same procedure can be done foe naked skeletal clusters Os2, Os3, Os4, Os5, Os6, Os7, Os8, Os9, and Os10. All these operations are summarized in Table 3. Since a:CO ligand removes one(1) k value from the basket, it has been assigned a k value of -1, that is :CO(K = -1) while Os(K =5). All the Os1 to Os10 carbonyl series in Table 4 have been constructed on the basis of this concept. The construction of all the carbonyl series was terminated when k = n-1 for meaningful clusters based upon the previous study [12]. In order to verify the validity of the skeletal linkage concept, we can readily identify a good number of well known osmium carbonyl clusters from Table 4. These include Os(CO)5, Os2(CO)9, [analogue of Fe2(CO)9], Os3(CO)12, Os4(CO016, Os5(CO)16, Os6(CO)21, Os6(CO)18, Os6(CO)19[existing as Os6(CO)182―], Os7(CO)21, Os8(CO)23, [existing as Os8(CO)222―], Os9(CO)25, [existing as Os9H(CO)24―], and Os10(CO)27 [existing as Os10(CO)262―]. A good number of osmium carbonyl clusters have been collected and categorized according to series using Table 4. These given in Table 5. They may include those already mentioned.
2.2.3. The k(n) Symbol as an Embodiment of Symmetry
Let us focus on the Os4 skeletal cluster in Table 3. The skeletal cluster has 20 skeletal linkage values in its ‘pocket’ since each skeletal element has 5. This is expressed by a symbol k(n) = 20(4). When fourteen carbonyl (CO) ligands get clustered around the Os4 skeletal fragment, according to series, they grab 14 skeletal linkage values from the pocket of the Os4 skeletal fragment leaving behind 6 in its pocket. This is symbolically expressed as k(n) = 6(4). If we refer to Table 3, this belongs to NIDO series, S = 4n+4 and has a TETRAHEDRAL SYMMETRY (Td). For convenience of description, let us refer to series, 4n+q, q = 2, 4, 6, 8, 10 and so on as LOWER SERIES and 4n+q, q = 0, -2, -4, -6, -8, -10 and so on as HIGHER SERIES. The higher series are capping series; 4n+0, mono-capped, C1C, 4n-2, bi-capped, C2C, 4n-4, tri-capped, C3C, and so on. The geometrical shapes of lower series appear to be easier to predict than those of higher series. Keeping our attention on the k(n) = 6(4), it is quite interesting to note that any cluster which fulfills the condition k(n) = 6(4) tends to adopt a TETRAHEDRAL SKELETAL GEOMETRY. This is well demonstrated by the 15 selected examples given in Table-5 which all have an ideal skeletal tetrahedral shape.
In this geometry, 6(4), there are 4 skeletal elements bound by 6 skeletal linkages.
What will be the shape of k(n) = 10(4)? This cluster corresponds to Os4(CO)10 complex. The complex is tri-capped, C3C[M-1]. This means, there 3 skeletal elements surrounding one at the center. The symbol, [M-1] means the one nuclear skeletal atom is a member of the CLOSO family, S = 4n+2, k = 2n-2 = 2(1)-1 = 1. That is, besides the one skeletal element being in the nucleus, it also has 1 skeletal linkage value in its pocket while the other 3 are just capping (linked to 3 other atoms including the one in the middle. A sketch of the possible skeletal sketch is shown in F-15.The change in symmetry from k(n) = 6(4) tetrahedral, S = 4n+4, NIDO cluster Os4(CO)14 to k(n) = 10(4), tri-capped C3C[M-1] cluster,Os4(CO)10 is dramatic.
2.2.4. The Capping Clusters
The concept of capping has already been briefly explained. The capping is soprevalent in golden clusters that it is important to touch it again. The series method, can readily be applied to categorize both the lower and upper series and where possible the corresponding structures predicted. It was overwhelming when applying series to the giant cluster formula, Ni38Pt6(CO)38(H)5―, that it was predicted that the cluster has a nucleus with an octahedral cluster and indeed an x-ray structure showed this to be the case [13]. Let us use the skeletal numbers in Table 1 and the fact that a 2 electron donor ligand such as CO removes 1 skeletal value from the ‘cluster pocket’ while a one electron donor such as H atom or a negative charge (represents 1e) removes 0.5 skeletal value from the cluster pocket to see how the cluster, Ni38Pt6(CO)38(H)5―, has an octahedral inner nucleus within it. The k value of the cluster is given by k = 38(4) + 6(4) – 38 - 0.5 - 5(0.5) = 125, n = 38 + 6 = 44. Also we know that the k value is related to the q value by q = 2[2n-k] = 2[88-125] = 2[-37] = -74. Hence the cluster series will be S = 4n-74. This has the capping symbol, Cp = CIC+C37C = C38C[M-6]. This symbol means the giant cluster of 44 skeletal elements, has within it an octahedral nucleus [M-6] of six skeletal atoms surrounded by 38 other skeletal atoms. In other words, we have a GIANT CLUSTER with a MINOR CLUSTER inside. What is the influence of the minor cluster on the overall shape of the giant cluster? Such a question is beyond the series at this moment. What was even more exciting was the fact that the 6 nuclear atoms are all found to be the platinum skeletal atoms [13]. Does this mean that in a capping cluster of mixed skeletal elements, the heavier elements have a tendency of being located within or near the nucleus? Using the series it was also easy to predict that Os6(CO)182―, will have an octahedral skeletal symmetry, [M-6], Os7(CO)21, will be a mono-capped cluster, C1C[M-6], Re8(C)(CO)242―, a bi-capped cluster, C2C[M-6], and Os10(CO)262―, is a tetra-capped octahedral, C4C[M-6] [10]. Whereas it is easy to visualize a cluster which mono-capped, bi-capped or tri-capped octahedral, it not easy to do so for large clusters such as C38C[M-6]. What interesting in the case of golden clusters, we can find relatively small clusters of the type C1C[M-1], C2C[M-1], C3C[M-1], C4C[M-1], C5C[M-1], C6C[M-1], C7C[M-1], C8C[M-1], C9C[M-1], C10C[M-1], and so on. All these clusters have 1 skeletal atom in the nucleus and the rest surround the nucleus. Again, the manner in which they surround the nuclear atom is beyond the scope of the series for now. However, when the x-ray structure is determined for these relatively small clusters and the one nuclear atom as predicted by the series is seen, there is some excitement.
2.3. Golden Clusters
2.3.1. Types of Golden Clusters
Applying the series method to golden clusters revealed that most of them are highly capped. It has been quite amazing to discover that all the following clusters have one skeletal element in the nucleus as predicted by series and confirmed by their x-ray structures [1, 4, 6]. The use of the series to categorize the golden clusters is indicated in the examples N-1, Ex-1 to Ex-4 above. The clusters are: Pt(AuL)82+, C8C[M-1]; Au8L72+, C7C[M-1]; Au9L83+, C8C[M-1] and Au10 Cl3L6+, C9C[M-1]. The other golden complexes that have been categorized by the series method include, (SR)(AuL)32+{C2C[M-2]}, the C3C[M-2] category include, C(AuL)4, N(AuL)4+, P(AuL)4+, and O(AuL)42+. The C4C[M-2] clusters include, C(AuL)5+, N(AuL)52+, and P(AuL)52+. Those which belong to C5C[M-2] are C(AuL)62+, (AuL)6Re(CO)3+ and S(AuL)64+. Most of these have been categorized by the series method and are illustrated in N-7 to N-16 with some explanations. What is interesting is that all these categories given in this last section are all capped based upon a two-centered CLOSO nucleus, [M-2]. The symbol, [M-2] means that the two skeletal elements at the nucleus belong to CLOSO family, S = 4n+2, k = 2n-1 = 2(2)-1 = 3. That is, the two closo atoms are triply bonded M≡M. The complexity in analyzing the shapes of gold clusters may further be illustrated by these two examples, Au6L62+{k(n) = 16(6), C5C[M-1]} is described as edge-sharing tetrahedron, while it is, according to series a penta-capped single skeletal atom centered cluster. On the other hand Au7L7+{k(n) = 18(7), C5C[M-2]} is described as a pentagonal bipyramid while according to series is regarded as penta-capped two-skeletal centered cluster. If it were a normal pentagonal bipyramid such as B7H72―, it would have k(n) =13(7) identity which is different from that of k(n) = 18(7). An osmium cluster, Os17(CO)362―, has a k value given by k =17(5)-36-1 = 48, k(n) = 48(17), q = 2[34-48] = 2[-14] = -28. Hence S = 4n-28, Cp = C1C+C14C = C15C[M-2]. According to series method, the cluster has a nucleus of 2 skeletal elements and is capped by 15 other skeletal elements. On looking at the literature structure [11] of Os17(CO)362―, the two nuclear atoms appear to be present.
2.3.2. The Application of 8-Electron and the 18-Electron Rules to Mixed Golden Clusters
The 8 or 18 electron rule is easily verified using the skeletal numbers. If the k value of the complex is zero (k=0), the cluster or complex obeys the rule. For instance, in CH4, k = 1(2)-4(0.5) = 0. Hence CH4 obeys the octet rule. The H atom is considered as a one electron donor which removes 0.5 k values from the carbon atom which is assigned the k value of 2 (see Table 2). Also in NH3, k = 1(1.5)-3(0.5) = 0, therefore NH3 obeys the octet rule. Consider the following complexes, FeH64―, k = 1(5)-6(0.5)-4(0.5)= 5-3-2 = 5-5 = 0. Hence the complex obeys the 18 electron rule. In Mo(CN)84―, k = 1(6)-8(0.5)-4(0.5) = 6-4-2 = 6-6 = 0, the complex obeys the 18 electron rule. The carbonyl complex, Fe(CO)5, k = 1(5)-5(1) = 5-5 = 0. The carbonyl complex obeys the 18 electron rule. These concepts are being applied in some of the worked out golden based examples given in the following sections.Let us consider the following examples as illustrations. The first, Se(AuL)42+. Using the skeletal numbers in Tables 1 and 2, we can calculate the k value of the cluster as follows, k = 1(1)+4(2.5)+1 = 12, n =1+4 = 5. Hence k(n) = 12(5), q = 2[2n-k ] =2[10-12] = 2[-2] = -4, S =4n-4, Cp = C3C[M-2], V = 4n-4 = 4(5)-4 =16. Metal adjustment, V = 16+10(4) = 56. Confirmation from the cluster formula, V = 1(6) + 4(11+2) - 2 = 56. The cluster is isolobal to Os5(CO)13, that is, Se(AuL)42+
Os5(CO)13. Selected possible isomeric skeletal shapes are given in F-16 and F-17.The ideal skeletal shape adopted by Se is as shown in F-17. The sulphur analogue adopts a similar shape whereas the oxygen analogue prefers F-16 [1]. This probably due to what we may refer to as “capping isomerism”.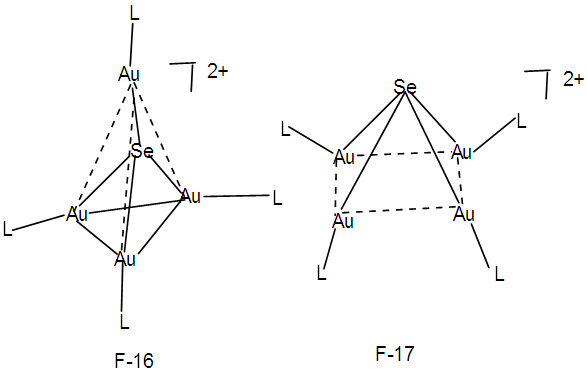
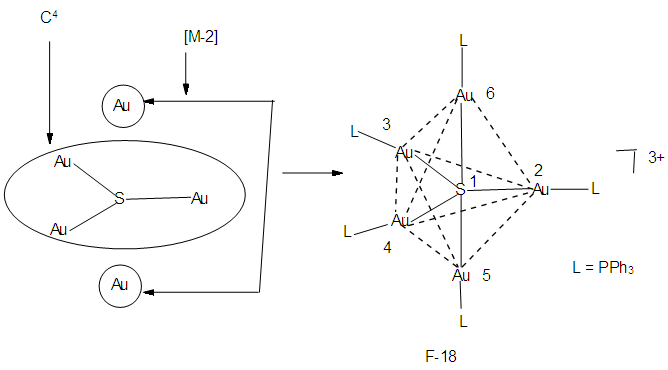
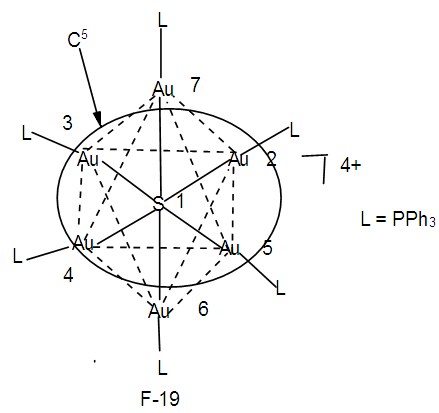
Let us take another example S(AuL)53+. This cluster can be split into fragments, S3+ (k =1+1.5 = 2.5) and 5AuL [5(3.5-1) = 12.5]. Hence net k = 2.5+12.5 = 15, n = 1+5 = 6. Therefore the cluster skeletal symbol k(n) = 15(6); q = 2[2n-k] = 2[12-15] = 2[-3] = -6, S = 4n-6, Cp = C4C[M-2]. The valence electrons, V =4n-6 = 4(6)-6 = 18. Metal adjustment, V = 18 + 10(5) = 68. Checking with the cluster formula, V = 1(6) + 5(11+2)-3 = 68. The possible shape is shown in F-18 in agreement with literature [6]. From the Table 4, S(AuL)53+
Os6(CO)15.We can use skeletal numbers to test whether the labeled skeletal elements obey the 8 or 18 electron rules. This is also easily done by applying skeletal numbers of the selected elements. For instance k1 = 2.5 -5(0.5) = 2.5-2.5 = 0. This means that the S3+ skeletal element obeys the octet rule. We can test this by considering the number of electrons on S3+ plus the electron donations from the 5(AuL) fragments through their bonds to S3+ skeletal element. Thus V = (6-3) + 5 = 8. Each of (AuL) is considered to donate one electron to the covalent bond. The k2 = 1(3.5)-1-5(0.5) = 3.5-3.5= 0. This means the Au skeletal atom at k2 obeys the 18 electron rule. But k2 = k3 =k4. Hence the equatorial Au skeletal atoms obey the 18 electron rule. Now k6 = 1(3.5)-1(1)-4(0.5) = 3.5-1-2 = 0.5. This means that the skeletal elements corresponding to k6 is short by 1e to reach the 18 electron rule, that is, V = 18-1 = 17e. This result will be the same as for k7.The last example to discuss is S(AuL)64+. We can split this into fragments S4+ and 6 (AuL). The corresponding k values are 1(1+2) = 3 and 6(2.5) = 15. The net k = 3+15 = 18. By going the same procedure as above, S = 4n-8, Cp = C5C[M-2]. This could guide us to produce a possible skeletal isomer as given in F-19. This sketch agrees with literature [6].k1 = 1(3)-6(0.5) = 3-3 = 0, the 8 electron rule is obeyed.k2= 1(3.5)-1(1)-5(0.5) = 3.5-1-2.5 = 3.5-3.5 = 0, the 18 electron rule is obeyed.k3 = k2 = k4 = k5. All the corresponding skeletal elements obey the 18 electron rule.k6 = 1(3.5)-1(1)-5(0.5) = 3.5-3.5 = 0, k7 = k6. Thus, skeletal elements 6 and 7, also obey the 18 electron rule. Finally, F-19 has an ideal octahedral shape with the central atom obeying the 8 electron rule and the rest of the skeletal atoms obeying the 18 electron rule. In terms of the series, the cluster may be regarded as being penta-capped system centered around 2 skeletal elements, C5C[M-2]. In order to reinforce the understanding of the calculation of the k values for the entire cluster and individual skeletal elements, we can work out the possible skeletal structure [10] and ligand distributions in Os5(CO)19. The k value of this cluster k =5(5)-19(1) = 6. One of the possible skeletal geometry is simply 2 joined triangles as in F-20.The skeletal sketch of Os5(CO)19 is shown in F-21.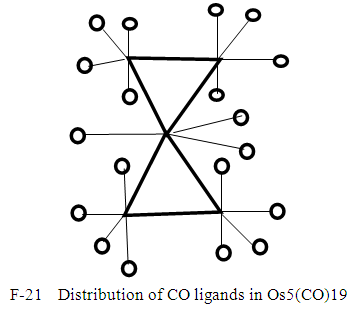
3. Conclusions
The skeletal numbers have been utilized to categorize clusters of gold containing p-block elements. All the golden clusters of central focus of this paper, namely N-7 to N-15 including Se(AuL)42+ and S(AuL)53+ have been found to be highly capped based on a nucleus comprising of two skeletal elements [M-2]. Hypothetical osmium cluster carbonyl series have been formulated to assist in categorizing the golden clusters. Some adequate background knowledge about series method has been provided to assist the readers to follow up the work on the analysis of the golden clusters. The series method has proven to offer a reasonable guide in the categorization and structural prediction of golden clusters containing p-block skeletal elements.
ACKNOWLEDGEMENTS
The university of Namibia is acknowledged for financial support and providing an enabling environment to do this work and NAMSOV, Namibia for financial assistance and Merab Kambamu Kiremire for proof-reading the draft.
References
[1] | Gimeno, M. C., The Chemistry of Gold in Modern Supra-molecular Gold Chemistry: Gold-Metal Interactions and Applications, Edited, Laguna, A., Wiley-VCH, 2008. |
[2] | Pauling, L.; Proc., Natl. Acad. Sci. USA, 74(12), 5335(1977). |
[3] | Mingos, D. M. P.; Pure and Appl. Chem., 59(2), 145(1987). |
[4] | Konishi, K.; Struc. Bond., 161, 49(2014). |
[5] | Kiremire, E. M. R., Inter. J. Chem., Canada (in press). |
[6] | Kwok-ming, L.; PhD Thesis, University of Hong Kong, 2011. |
[7] | Kiremire, E. M. R.; Int. J. Chem., 8(4), 78 (2016). |
[8] | Kiremire, E. M. R.; Orient. J. Chem. 31(2), 605(2015). |
[9] | Mingos, D. M. P.; Gold Bull., 17(1), 5(1984). |
[10] | Pivoriũnas, G., PhD Thesis, 2005, Ebert-Karls University Tübingen. |
[11] | Hughes, A. K., Wade, K.; Coord. Chem. Rev., 197, 19(2000). |
[12] | Kiremire, E. M. R.; Int. J. Chem., 8(3), 35(2016). |
[13] | Rossi, F., Zanello, P.; Electrochim. Acta, 29,309(2011). |
[14] | Hoffmann, R.; Angew. Chem., Int. Ed., 21(10), (1982). |
[15] | Kiremire, E. M.; Orient. J. Chem., 31(Spl. Edn), 59(2015). |