Jeddah Marie G. Vasquez, Terence P. Tumolva
University of the Philippines, Diliman, Quezon City, Philippines
Correspondence to: Jeddah Marie G. Vasquez, University of the Philippines, Diliman, Quezon City, Philippines.
Email: |  |
Copyright © 2015 Scientific & Academic Publishing. All Rights Reserved.
Abstract
Hydrogels are hydrophilic polymers that can swell and absorb water without dissolving, provided that chemical or physical crosslinks exist among the macromolecular chains. The hydrogel can be derived from synthetic and natural polymers, the latter having desirable properties such as biocompatibility and biodegradation that do not produce adverse and reproductive and developmental effects. The aim of this study was to synthesize a physical hydrogel from a water-soluble cellulose derivative and a solution of 6 wt% sodium hydroxide/5 wt% thiourea. Physical hydrogels (also called self-assembling hydrogels) are formed when macromolecules self-assemble through non-covalent, secondary molecular interactions such as hydrophobic, electrostatic, and H-bonding. Hydroxyethyl cellulose, hydroxypropyl cellulose, and microcrystalline cellulose were used in this study. A hydrogel made from hydroxyethyl cellulose containing weight percentages of 5, 7.5, 10, and 12.5 wt% in the sodium hydroxide/thiourea solution. Hydroxypropyl cellulose only formed a cloudy yellow solution while microcrystalline cellulose did not dissolve at all in the solution. The hydroxyethyl cellulose/ sodium hydroxide/thiourea hydrogels were then characterized according to its functional groups present, physical structure, and mechanical properties, and biocompatibility.
Keywords:
Hydrogels, Natural polymers, Hydroxyethyl cellulose, Physicochemical properties
Cite this paper: Jeddah Marie G. Vasquez, Terence P. Tumolva, Synthesis and Characterization of a Self-Assembling Hydrogel from Water-Soluble Cellulose Derivatives and Sodium Hydroxide/Thiourea Solution, American Journal of Chemistry, Vol. 5 No. 2, 2015, pp. 60-65. doi: 10.5923/j.chemistry.20150502.03.
1. Introduction
Hydrogels are hydrophilic polymers that can swell and absorb water without dissolving, provided that chemical or physical crosslinks exist among the macromolecular chains [1]. The hydrogel can be derived from synthetic and natural polymers, the latter having desirable properties such as biocompatibility and biodegradation that do not produce adverse and reproductive and developmental effects [2].The properties of the hydrogel allow it to have applications in several fields of study. Biomedical engineering, in particular, can use it as the aqueous environment of the hydrogel can protect cells and drugs, and the modifiability of hydrogels to form at different temperatures or pHs [3, 4]. Specific applications of hydrogels made from natural polymers, such as modified cellulose, include soft contact lens, phantoms for ultrasound imaging, wound healing dressings, cell immobilization islets, and bioactive scaffolds for regenerative medicine [1, 5].Physical hydrogels (also called self-assembling hydrogels) are formed when macromolecules self-assemble through non-covalent, secondary molecular interactions such as hydrophobic, electrostatic, and H-bonding [6].Water-soluble macromolecules bearing attractive groups that can develop physical bonds through intermolecular associations when they are dissolved in aqueous media belong to a broad class of polymeric systems, namely “associative” polymers (APs), which are among the best polymeric building elements for the creation of temporary networks [6]. The self-organization of these APs when dissolved in water, results in a dramatic increase in the viscosity of the medium [6].In recent decades, the need of physical crosslinked gels has been potentially increased to avoid the use of chemical crosslinking agents and reagents [7]. These agents are not only often toxic compounds which can be removed or extracted from prepared gels before applications, but also can affect the integrity of substances when entrapped (e.g. proteins, drugs, and cells) [8]. Therefore, the physical crosslinking method has been chosen and preferred comparably to the chemical crosslinking method for most crosslinked polymer’s preparation [8].Polysaccharides are one class of polymers that are able to form hydrogels that are highly hydrated and porous, being similar to living tissue and have low toxicity [3]. Chitosan, starch-based, gellan gum, hyaluronic acid, aginate, and cellulose and its derivatives have been used to prepare natural hydrogels.Cellulose is the most abundant naturally occurring polymer of glucose, made up of glucose units held together by 1,4-β-glucosidic linkages [9]. Chemical modification of cellulose, usually involving esterification or etherification of the hydroxyl groups, is performed to produce cellulose derivatives, named cellulosics, which are more easily processable and are largely applied in industries [1]. The degree of substitution, defined as the average number of etherified hydroxyl groups in a glucose unit, can be controlled to a certain extent, in order to obtain cellulose derivatives with given solubility and viscosity in water solutions [1].Cellulose and cellulose-based hydrogels in particular offer various advantages over synthetic hydrogels. Microbial cellulose hydrogels have similar properties to synthetic hydrogels in that they display high water content (98-99%) and good sorption of liquids, it is non-allergenic, and can be safely sterilized [3]. Other advantages include its biodegradability, smart, stimuli-responsive behavior, availability in nature, and the low cost of cellulose and its derivatives [1, 3].The aim of the study is to synthesize a physically crosslinked hydrogel from a cellulosic polysaccharide: microcrystalline cellulose (MCC), hydroxyethyl cellulose (HEC), or hydroxypropyl cellulose (HPC) upon the addition of a 6 wt% sodium hydroxide/ 5 wt% thiourea solution.
2. Methodology, Results, and Discussion
2.1. Preparation of Sodium Hydroxide/Thiourea Solution
A 1000 g solution of 6 wt% sodium hydroxide/5 wt% thiourea was prepared by dissolving 60 grams sodium hydroxide and 50 grams thiourea in 890 grams water. The solution was stored at room temperature.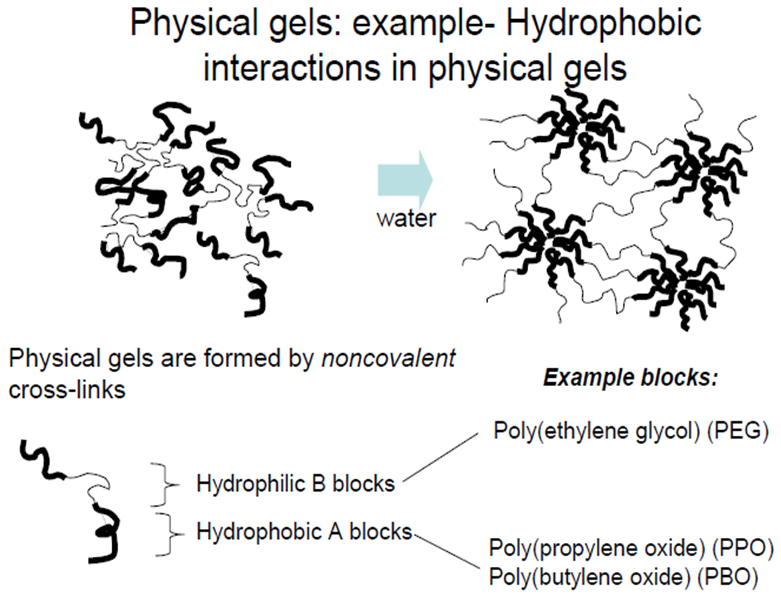 | Figure 1. Example of a physical hydrogel formation [13] |
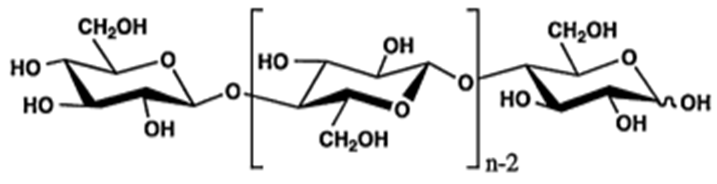 | Figure 2. Chemical structure of cellulose [9] |
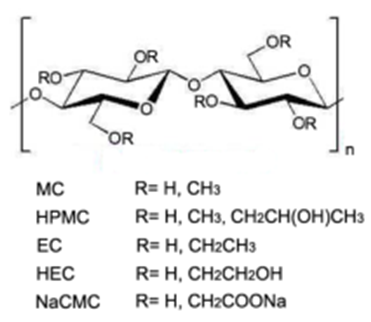 | Figure 3. Cellulose derivatives; MC=methylcellulose, HMPC=hydroxypropyl methylcellulose, EC=ethylcellulose, HEC=hydroxyethylcellulose, NaCMC=sodium carboxymethylcellulose [1] |
2.2. Synthesis of the Hydrogel and Hydrogel Film
Microcrystalline cellulose (MCC), hydroxypropyl cellulose (HPC), and hydroxyethyl cellulose (HEC) were used to determine if a hydrogel would be formed from the prepared sodium hydroxide/thiourea (NaOH/thiourea) solution at room temperature. Varying weight percentages of 5, 7.5, 10, and 12.5 wt% cellulose or cellulose derivative added with the sodium hydroxide to create a total of 20 grams. The cellulose derivatives that had formed a well-mixed gel were then used for further analysis and characterization of its properties. The synthesized hydrogels were dried at 50°C for 24 hours in order to create a hydrogel film with a reduced water content that will be used for FTIR characterization and measurement of gel fraction and swelling ratio.At room temperature of 25°C, only hydroxyethyl cellulose was able to form a hydrogel spontaneously at the given weight percentages of 5, 7.5, 10, and 12.5 wt%. Microcrystalline cellulose remained insoluble to the mixture and hydroxypropyl cellulose dissolved in the solution but did not form a gel, as seen on Figure 5 with the solution tilted slightly to show the lack of gelling.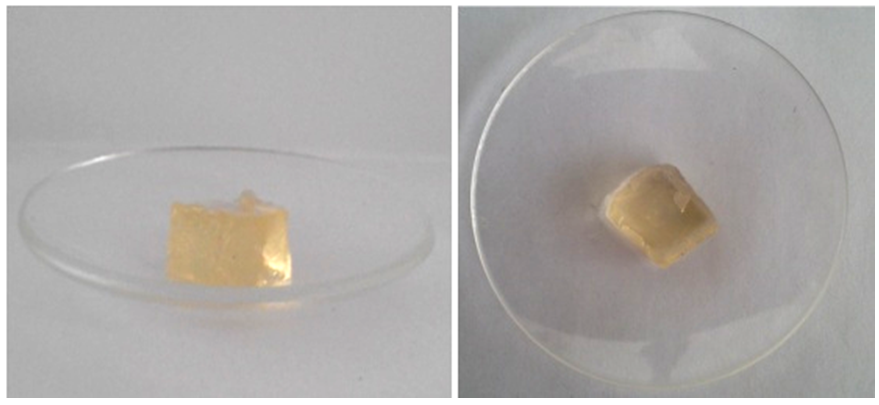 | Figure 4. Front and top views of synthesized 12.5 wt% hydroxyethyl cellulose hydrogel |
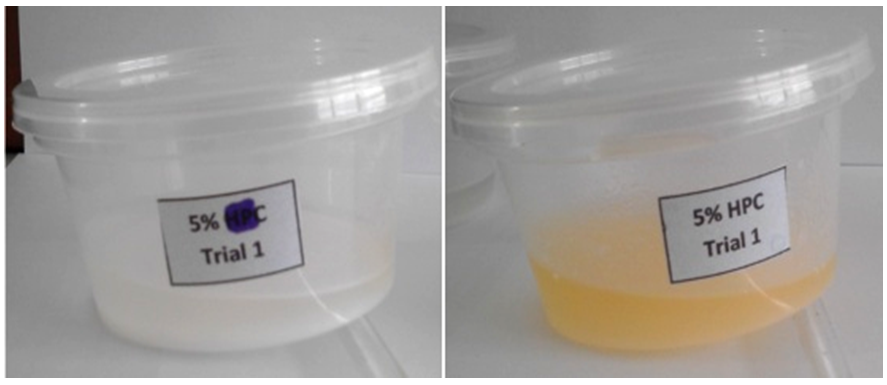 | Figure 5. Mixture of cellulose (left) and hydroxypropyl cellulose (right) with 6 wt% NaOH/5 wt% thiourea at room temperature |
2.3. FTIR Characterization of the Hydrogel
Oven dried samples of thiourea, thiourea/NaOH solution, and resulting HEC/NaOH/thiourea hydrogels were analyzed by FTIR on a Thermo Nicolet 6700 instrument. The FTIR spectra were obtained by recording 64 scans between 4000 and 400 cm-1 with a resolution of 16 cm-1. All samples were measured in absorbance mode.In Figure 5, FTIR spectra of pure thiourea, pure hydroxyethyl cellulose, and dried NaOH/thiourea solution as the hydrogel components are shown. Upon the addition of sodium hydroxide to the thiourea in the NaOH/thiourea solution, no new peaks are formed indicating that no new species are formed.From Figure 6 there was no obvious difference in the chemical shifts or peak shapes between the spectra of the NaOH/thiourea solution, dried HEC and those of the gels indicating that the chemical environments of carbon atoms on the macromolecular chain practically remain the same during the transition. In other words, the networks at the gel state were caused by a physical interaction junction rather than by a covalent bond.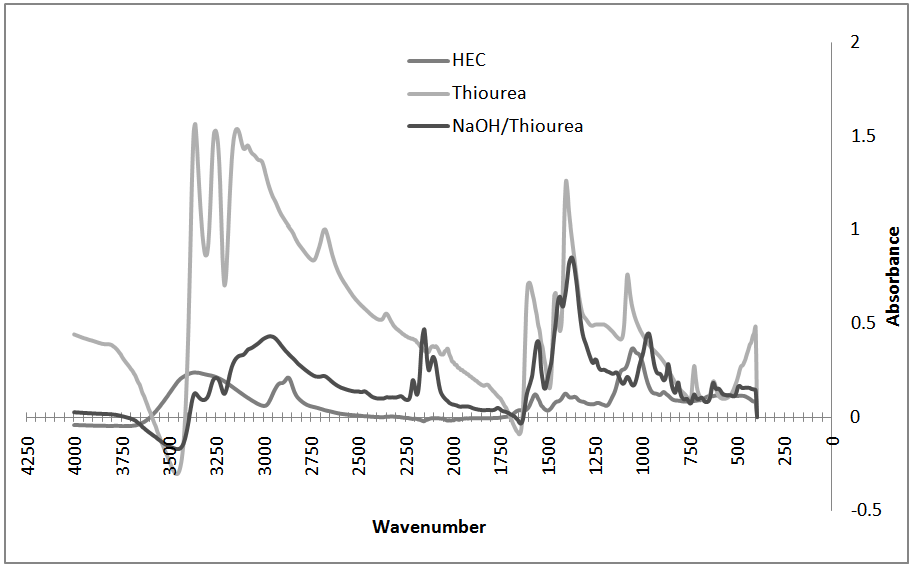 | Figure 6. FTIR spectra of hydrogel components and pure thiourea |
The study of Weng, et al. (2013), explains that in the HEC/NaOH/thiourea aqueous solution system, the presence of NaOH can break the intermolecular hydrogen bonds of hydroxyethyl cellulose to create a significant ion-pair interaction, which gives a chance for the water or thiourea molecules to form hydrogen bonds with the released -OH groups on the cellulose chains. The thiourea with highly polar C=S and NH2 groups serves as a hydrogen bonding donor and receptor between thiourea and cellulose to form new intermolecular interactions. The stable network units were, thus, formed by the random association of cellulose chains and further aggregated to form gels.
2.4. Measurement of Gel Fraction and Water Uptake of the Hydrogel
The obtained hydrogels were dried at 50°C in an oven for 24 h and weighed
The dried xerogel samples were soaked in distilled water for 24 h up to an equilibrium swelling weight
for removing the leachable or soluble HES parts from membrane. The gel was then dried directly at 50°C in an oven and weighed again
The gel fraction (GF %) was calculated by the following equation [10].
In order to measure the swelling degree of the hydrogel, samples were cut into 2 x 2 cm pieces and dried at 50°C in an oven for 24 h, and the weight of the dried sample was determined
The dried samples were then soaked in distilled water, maintained and then weighed
at specific interval times. The water uptake of the hydrogel was determined using the following formula [10].
Figure 7 presents the water uptake percent of the hydrogels membranes versus HEC content. Increasing the HEC content increases the gel fraction and swelling ratio of the hydrogel. However, beyond the limit of its swelling the hydrogel has a tendency to be dissolved into the solution. This is due to the components being physically crosslinked only and HEC has a soluble in water, which is the swelling medium.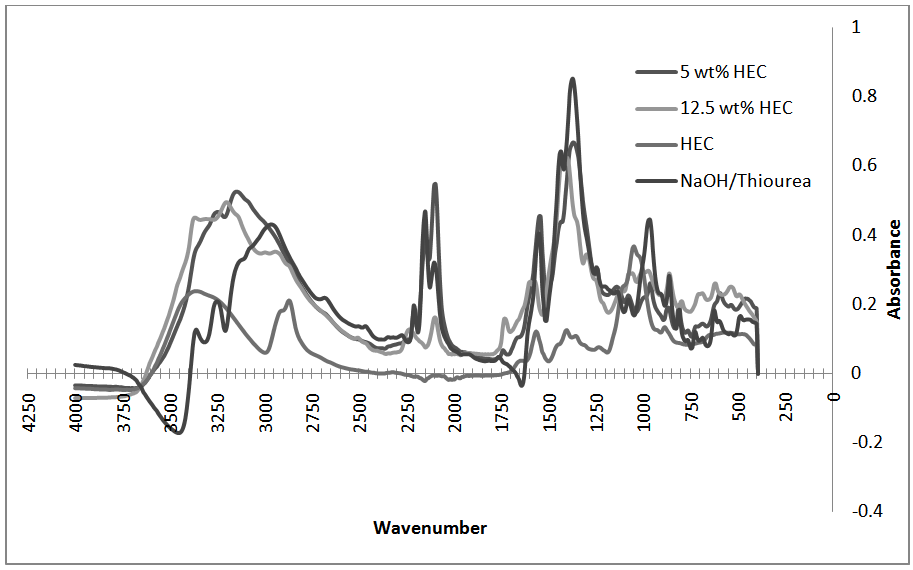 | Figure 7. FTIR Spectra of hydrogels and hydrogel components |
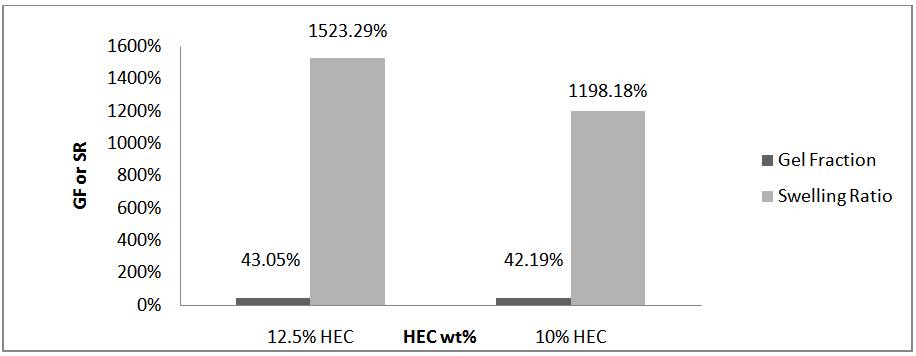 | Figure 8. Gel fraction and swelling ratio of hydrogels |
2.5. Strength of Adhesion of the Hydrogel
Using the method used by Nie, et al. (2013), 10 wt% gelatin solution was uniformly spread on the surface of a glass slide containing dimensions of 1 mm x 25 mm x 76 mm. The tissue adhesion strength was investigated according to the method modified from ASTM F2255-03. Various hydrogel solutions were applied to a 25 mm x 25 mm area on one slide and another slide was placed over it. The bonded slides were left to cure overnight before testing. More than five specimens of each group were subjected to uniaxial strain at a constant crosshead speed of 10 mm/min. The maximum strength was recorded and the adhesion strength was calculated as:
The adhesion strength of the HEC/NaOH/thiourea hydrogels ranged between approximately 1.006 to 13.950 kPa. The average bonding strength increased with increasing hydroxyethylcellulose content.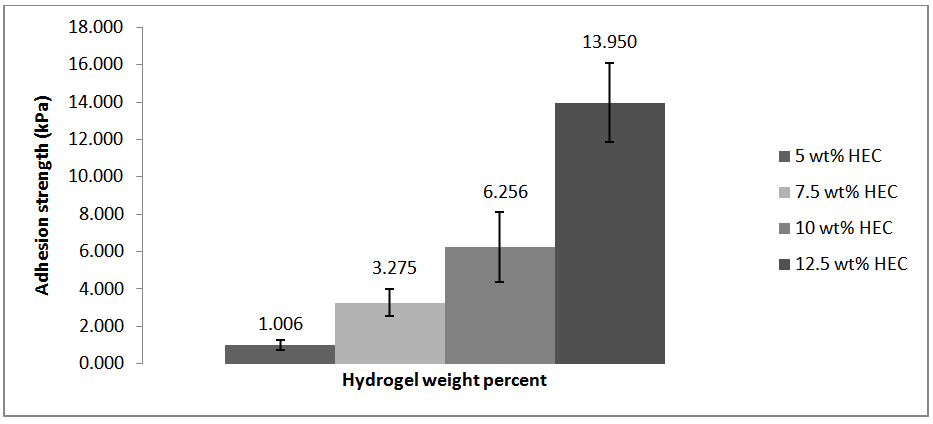 | Figure 9. Adhesion strength results |
3. Conclusions
In conclusion a hydrogel was formed from mixing hydroxyethyl cellulose with a sodium hydroxide/thiourea solution at ambient conditions. The hydrogel at 12.5 wt% HEC has a gel fraction of 43.05%, swelling ratio/water uptake of 1523.29% and adhesion strength of 13.950 kPa.
ACKNOWLEDGEMENTS
Authors gratefully acknowledge the funding provided by the Engineering Research and Development for Techology, College of Engineering, University of the Philippines - Diliman.
References
[1] | Sannino, A., et al., "Biodegradable Cellulose-based Hydrogels: Design and Applications" Materials, Vol. 2, 2009 pp. 353-373. |
[2] | Li, Y., et al., "In situ hydrogel constructed by starch-based nanoparticles via a Schiff base reaction" Carbohydrate Polymers, Vol. 110, 2014, pp. 87-94. |
[3] | Fernandes, EM, "Bionanocomposites from lignocellulosic resources: Properties, applications and future trends for their use in the biomedical field" Progress in Polymer Science, Vol. 28, 2013, pp. 1415-1441. |
[4] | Jeong, B., et al., “Thermosensitive sol-gel reversible hydrogels” Advanced Drug Delivery Reviews, Vol. 64, 2012 pp. 154-162. |
[5] | Ahmed, EM, "Hydrogel: Preparation, characterization, and applications" Cairo University Journal of Advanced Research, 2013. |
[6] | Tsistilianis, C., Physical Hydrogels in: Encyclopedia of Polymer Science and Technology. |
[7] | Van Tomme, S.R., et al., “Self-gelling hydrogels based on oppositely charged dextran microsphere” Biomaterials, Vol. 26, 2005, pp. 2129-2135. |
[8] | Rong J., In-Situ Forming Biomimetic Hydrogels for Tissue Regeneration, Biomedicine, 2012. |
[9] | Brown, W., et al., Introduction to Organic Chemistry, 3rd edition. John Wiley and Sons, Inc., 2005, pp. 513-522. |
[10] | Kenawy, E.R., et al., “Physically crosslinked poly(vinyl alcohol)-hydroxyethyl starch blend hydrogel membranes: Synthesis and characterization for biomedical applications” Arabian Journal of Chemistry, Vol. 7, 2013, pp. 372-380. |
[11] | Weng, L., et al., "Thermal Gelation of Cellulose in a NaOH/Thiourea Aqueous Solution" Langmuir, Vol. 20, 2003, pp. 2086-2093. |
[12] | Nie, W., et al., "Rapidly in situ forming chitosan/ε-polylysine hydrogels for adhesive sealants and hemostatic materials" Carbohydrate Polymers, Vol. 96, 2013, pp. 342-348. |
[13] | P.J. Flory, Statistical thermodynamics of networks and network swelling in: Principles of Polymer Chemistry,’ Cornell University Press, Ithaca, pp. 464-469. |