Naema A. Hikmat1, Bushra B. Qassim1, Mohammed T. Khethi2
1Department of Chemistry, College of Science, University of Baghdad, Baghdad, Iraq
2Thi-Qar University, Thi-Qar City, Iraq
Correspondence to: Naema A. Hikmat, Department of Chemistry, College of Science, University of Baghdad, Baghdad, Iraq.
Email: |  |
Copyright © 2014 Scientific & Academic Publishing. All Rights Reserved.
Abstract
The aim of this work is to investigate the key parameters and mechanism affecting the removal of Pb(II) metal ion from aqueous streams, using agricultural waste materials adsorbents such as leaf base (petiole) and fiber of the date palm, not as nature form but after being modified to activated carbon for increasing their sorption capabilities. Using Batch mode, the study showed the equilibrium time was relatively fast and equilibrium was reached after about 30 and 40 min, for Pb(II) by leaf and fiber respectively, the obtained result indicated that over pH range (6.5-7) there is an increased optimum of adsorption, (0.5g) of adsorbent weight is very ideal to obtain the best adsorption, the removal of Pb(II) ions by adsorbents decreased with the increase of temperature, indicating that the adsorption process was exothermic in nature. The isotherms of Pb(II) ions on the two adsorbents obeyed Langmuir isotherm better than Freundlich isotherm. Thermodynamic parameters such as (ΔGo), (ΔHo) and (ΔSo) have been evaluated. It has been found that the sorption process was feasible, spontaneous and exothermic in nature. Calculated kinetic parameters showed that the pseudo first order model is best fit to the experimental data. Adsorption process is best method for removal of metal from waste water because it is simple, time saving, inexpensive, high efficiency regeneration of adsorbents.
Keywords:
Thermodynamic, Kinetic, Adsorption, Lead, Petiole, Fiber, Palm
Cite this paper: Naema A. Hikmat, Bushra B. Qassim, Mohammed T. Khethi, Thermodynamic and Kinetic Studies of Lead Adsorption from Aquesous Solution onto Petiole and Fiber of Palm Tree, American Journal of Chemistry, Vol. 4 No. 4, 2014, pp. 116-124. doi: 10.5923/j.chemistry.20140404.02.
1. Introduction
Toxic heavy metal ions get introduced to the aquatic streams by means of various industrial activities via mining, refining ores, fertilizer industries, tanneries, batteries, paper industries, and pesticides etc., which process a serious threat to environment, as in [1].Various regulatory bodies have set the maximum prescribed limits for the discharge of toxic heavy metals in the aquatic systems. However the metal ions are being added to the water stream at a much higher concentration than the prescribed limits by industrial activities, thus leading to the health hazards and environmental pollution as in Table 1.Table 1. Permissible limits and health effects of Lead in drinking water, as in [2] 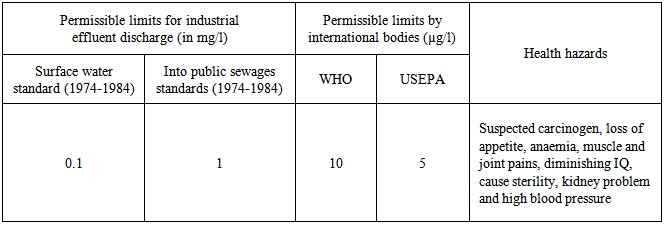 |
| |
|
Conventional methods for removal of metal ions from aqueous solutions include chemical precipitation, ion exchangers, chemical oxidation/reduction, reverse osmosis, electro dialysis, ultra filtration etc.; Conventional techniques have their own inherent limitations such as less efficiency, sensitive operating conditions, and production of secondary sludge and further the disposal is a costly affair.Another powerful technology is adsorption of heavy metals by activated carbon for treating domestic and industrial waste water. However the high cost of activated carbon and its loss during the regeneration restricts its application. Since 1990’s the adsorption of heavy metal ions by low cost renewable organic materials has gained momentum. The utilization of seaweeds, moulds, yeasts, and other dead microbial biomass and agricultural waste materials for removal of heavy metals has been explored, as in [3]. Recently attention has been diverted towards the biomaterials which are by products or the wastes from large scale industrial operations and agricultural waste materials, as in [4].The major advantages of biosorption over conventional treatment methods include: low cost, high efficiency, minimization of chemical or biological sludge, no additional nutrient requirement, and regeneration of adsorbents and possibility of metal recovery, as in [5].Agricultural materials particularly those containing cellulose show potential metal biosorption capacity. The basic components of the agricultural waste materials biomass include hemicelluloses, lignin, extractive, lipids, proteins, simple sugars, water hydrocarbons, starch contain a variety of functional groups that facilitates metal complexion which helps for the sequestering of heavy metals, as in [6].Agricultural waste materials being economic and eco-friendly due to their unique chemical composition, availability in abundance, renewable, low in cost and more efficient are seem to be viable option for heavy metal remediation.Several studies reveal that various agricultural waste materials such as rice bran, rice husk, wheat bran, wheat husk, saw dust of various plants, bark of the trees, groundnut shells, coconut shells, black gram husk, hazelnut shells, walnut shells, cotton seed hulls, waste tea leaves, Cassia fistula leaves, maize corn cob, jatropa de-oiled cakes, sugarcane bagasse, apple, banana, orange peels, soybean hulls, grapes stalks, water hyacinth, sugar beet pulp, sunflower stalks, coffee beans, arjun nuts, cotton stalks etc., has been tried . These promising agricultural waste materials are used in the removal of metal ions either in their natural form or after some physical or chemical modification, as in [6]. This paper describes a study of the use of petiole and fiber of date palm as an adsorbent for removal of lead from aqueous solution and waste water. It was found that petiole and fiber of date palm is an excellent adsorbent for removal of lead, as in [7].
2. Materials and Methods
2.1. Lead Standard Solution
Lead solutions were prepared by dissolving the appropriate amount of Pb(NO3)2 with several metal concentrations: (5, 10, 15 and 20) mg/L.
2.2. The Adsorbents
Two types of adsorbents were used in this work, Leaf Base (Petiole) and Fiber of Palm dates production. The adsorbent of date palm tree of type (Sukkary) was obtained from Nasiriya City south of Iraq.Table 2 shows the main components of the Leaf Base (Petiole) and Palm Fiber according to Food and Agriculture Organization (FAO), as in [8]. The pattern of sorption of metals on plant materials is attributable to the active groups and bond present on them, as in [9]. The active groups, such as amino, carbonyl and hydroxyl, present in the adsorbent are responsible for binding metal ions. Table 2. The chemical analysis of leaf base and fiber of the date palm 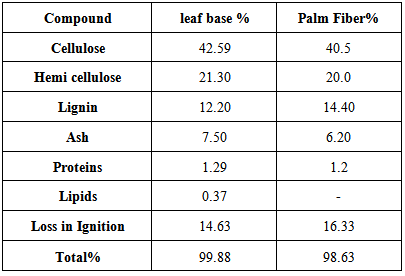 |
| |
|
2.3. Characterization of Adsorbents
The base of the leaves was cut and the external layer was removed completely. The leaf base (petiole) and fiber were divided into sections, dried at (110℃) for one day, left to cool at room temperature and kept in air light containers. The sections were ground to obtain a yellowish tint and brown powder respectively which is insoluble in water, acids, alkali hydroxides, and ether. It swells with little amount of water. The BET surface area of the powders were measured before and after activated to petiole and fiber charcoal powders at 900℃; Table 3, the BET surface area of petiole has been found to equal 210 m2.g-1 and 322 m2.g-1 for palm fiber, these values are very high comparative with other carbons which have a surface area about 10-100 m2.g-1.The adsorption capacity of carbon is strongly attributed to the chemical structure of its surface.Table 3. The BET surface area of by-products before and after activation 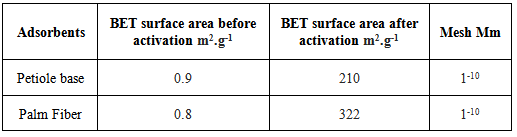 |
| |
|
2.4. Adsorption Isotherm
The adsorption isotherm for heavy metals solutions were determined by using Batch adsorption experiment: (25 ml) of heavy metal solution of a known concentration ranged from (5 mg/L) to (20 mg/L) were added separately to volumetric flasks containing (0.5 g) of each adsorbents. At a certain temperature 25℃ and optimum pH, the flasks were shaken in a thermostatically controlled shaker incubator at a constant speed (10 cyclers per minutes) for the required equilibrium time. The mixtures were then separated by centrifugation at (1500 rpm) for (10 minutes) and were filtered by using (No.42) Whatman filter paper. The metal Equilibrium concentrations were measured by using Atomic Absorption Spectrometer (AAS) and comparing the experimental data with the calibration curve. The amount of heavy metals adsorbed was calculated from the initial and final concentrations and the volume of solution according to the following equation, as in [10]: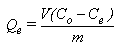 | (1) |
Where, V = Volume of solution (L)Co = initial concentration (mg/L)Ce = equilibrium concentration (mg/L)m = weight of adsorbent (g)The amount of adsorption is expressed by (Qe) which is defined as the quantity of adsorbate in (mg) held by weight of adsorbent in (1g).
3. Results and Discussion
3.1. Factors Affecting Adsorption of Heavy Metals by Adsorbents
3.1.1. Contact Time Effect
The effect of period of contact time between the adsorbent and adsorbate on the removal of the metal ions was determined by keeping pH, temperature, weight and initial concentration constant, the rate of Pb(II) removal by petiole base and fiber of palm with contact time. The rate of uptake of Pb(II) metal ion was quite rapid, 30 and 40 min by petiole and fiber respectively. The obtained results are shown in Table 4 and in Figure 1.Table 4. Effect of contact time on rate of Pb(II) removal by petiole base and fiber of palm at 25±2℃, adsorbent dose (0.5)g, initial concentration 5mg\L and pH (6.5) 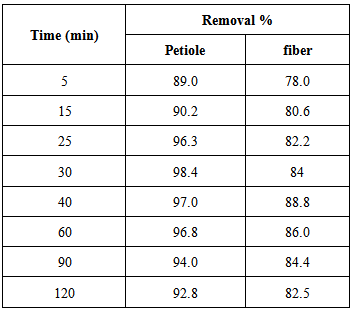 |
| |
|
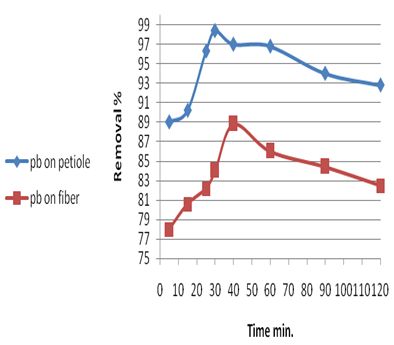 | Figure 1. Effect of contact time on rate of Pb(II) removal by petiole base and fiber of palm at 25±2℃, adsorbent dose (0.5)g, initial concentration 5mg\L and pH(6.5) |
The Adsorption Removal efficiency (AR), at time t, is calculated from the following relation: 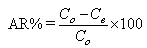 | (2) |
3.1.2. Effect of pH
The pH of the solution has a significant impact on the uptake of heavy metals since it determines the surface charge of the adsorbent and the degree of ionization and speciation of the adsorbate, as in [11]. The effect of the variation in the pH of the solution on adsorption extent of Pb(II) on the petiole and fiber were examined in a series of Pb(11) experiments at varying pH between 3.5 and 9. The obtained results are shown in Table 5 and in Figure 2.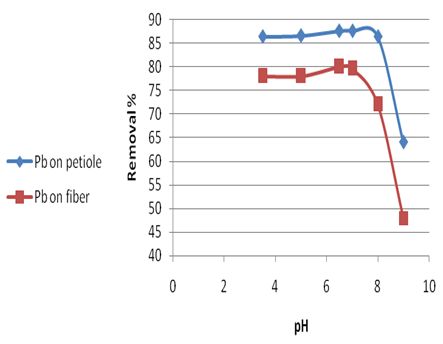 | Figure 2. Effect of pH on rate of Pb (II) removal for the petiole and fiber surfaces at 25±2℃, adsorbed dose (0.5)g, initial concentration 5mg\L and contact time 30 and 40min for petiole and fiber respectively |
Table 5. Effect of pH on rate of Pb(II) removal for the petiole and fiber surfaces at 25±2℃, adsorbent dose( 0.5)g, initial concentration 5mg\L and contact time 30 min 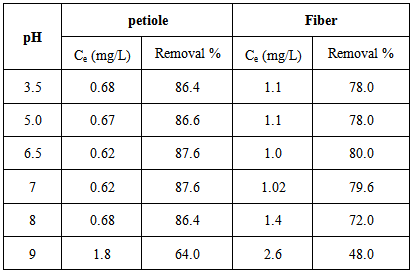 |
| |
|
These results indicated that in the pH range (6.5–7.0) there is an increase optimum of adsorption amounts for element ions. However the adsorption amounts for element ions decrease at pH above (7) due to the decreases of the negative charge of the surface, or caused by contamination which attributed to extended of metals ions to precipitation in base medium solutions (not precipitation may become significant mechanism in the metal removal process).
3.1.3. Adsorbent Weight Effect
The effect of different weight of adsorbents, that range from (0.1-1.2) g for adsorbent were assessed and the percentage of adsorption for different weights was determined by keeping all other factors constant. The research work aimed to find the amount of adsorbent that is required to attain surface saturation at certain conditions. The results are presented in Table 6 and Figure 3. It is very clear from this table and figure that the tested adsorbents doses (0.5g) of adsorbent weight were very ideal to obtain at best adsorption for Pb(II).Table 6. Effect of the adsorbent weight change on rate of Pb(II) removal for the petiole and fiber surfaces at 25 ± 2℃., pH (6.5), initial concentration 5mg\L and contact time 30and 40min for petiole and fiber respectively 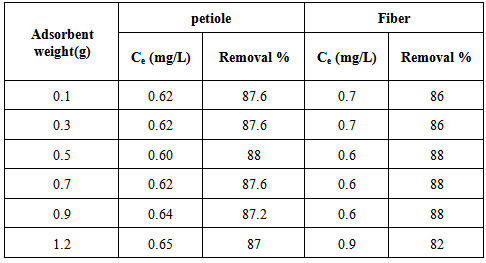 |
| |
|
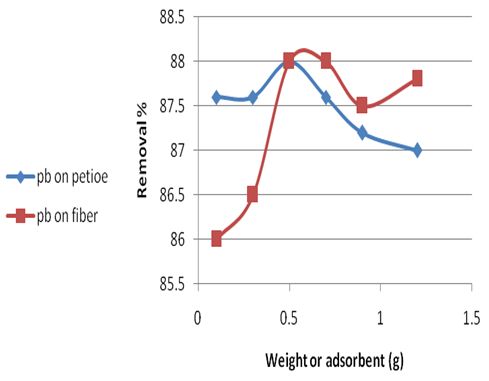 | Figure 3. Effect of the adsorbent weight change on rate of Pb(II) removal for the petiole and fiber surfaces at 25 ± 2 ℃, pH(6.5), initial concentration 5mg\L and contact time 30 and 40min for petiole and fiber respectively |
3.1.4. Effect of Initial Metal Concentration
The effect of initial metal concentration on rate of Pb(II) removal was carried out at 25±2℃ using different initial metal ion concentrations in the range (5, 10, 15 and 20 mg/L) at optimum pH and adsorbent dose, are shown in Table 7 and Figure 4, which indicate that the percentage removal decreases with the increase in the initial ion concentration, this is because there were no more adsorption sites on the adsorption surface of the adsorbent material, as in [12]. The results showed that the maximum removal percentage of Pb(II) was 96% by using fiber at a metal initial concentration 5mg/L.Table 7. Effect of initial concentration for Pb (II) removal on the petiole and fiber of palm at 25±2℃, pH (6.5), adsorbent dose (0.5)g, initial concentration 5mg\L and contact time 30 and 40min for petiole and fiber respectively 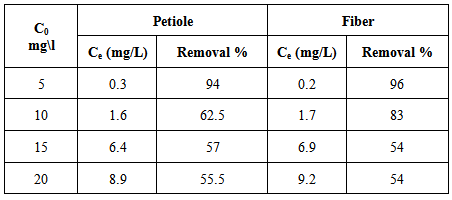 |
| |
|
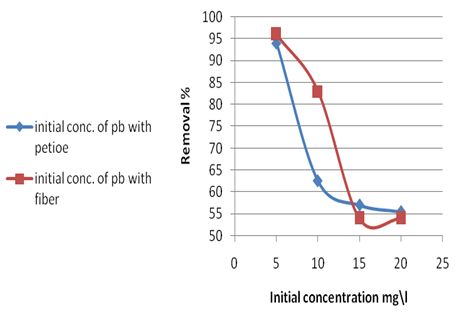 | Figure 4. Effect of initial concentration on rate of Pb(II) removal for petiole and fiber at 25±2℃, adsorbed dose(0.5)g, pH (6.5-7), initial concentration 5mg\L and contact time 30 and 40min for petiole and fiber respectively |
The results suggest that the available binding sites on the biomass may have different affinities towards specific metal ions. The binding strength of a metal ion to the biomass is dependent upon different factors such as hydration effects, hydrolysis effects and covalent binding of a metal ion. Hydration effects can dominate when the binding is weak. The larger ions, such as Pb(II), are less strongly hydrated and preferably accumulated at the interface.
3.2. Adsorption ISotherms
The relationship between the amount of a substance adsorbed per unit mass of adsorbent at constant temperature and its concentration in equilibrium is called the adsorption isotherm. The equilibrium adsorption isotherms are important in determining the adsorption capacity of Pb(II) metal ions. Two theoretical isotherm models were used; Langmuir and Freundlich model.
3.2.1. Langmuir ISOTherm
The general form of the Langmuir equation is (Langmuir, 1918). The Langmuir isotherm equation:  | (3) |
and in linearized form is: | (4) |
where Qm and b are Langmuir constants related to the sorption capacity, and sorption energy, respectively, Ce is the equilibrium concentration in mg/L, and Qe is the amount of adsorbate adsorbed per unit weight of adsorbent (mg/g).
3.2.2. Freundlich ISOtherm
Freundlich equation could be written as follows: | (5) |
and in linearized form is: | (6) |
where Ce is the equilibrium concentration in mg/L, Qf is the amount of adsorbate adsorbed per unit weight of adsorbent (mg/g). Kf is a parameter related to the temperature and n is a characteristic constant for the adsorption system under study. The linear plots of Ce/Qe versus Ce and log Ce versus log Qe are shown in Figures 5 and 6. The adsorption of metals ions on two adsorbents seems to follow the Langmuir isotherm as well as Freundlich isotherm. The values of correlation coefficient of Langmuir and Freundlich and the constants for adsorption of the two adsorbents are summarized in Table 8. The Langmuir, experimental data were better fitted to the Langmuir equation than the Freundlich equation and this indicate that saturated monolayer coverage is formed on the surface of the adsorbent.Table 8. Freundlich and Langmuir isotherm constants for Pb(II) uptake by petiole and fiber 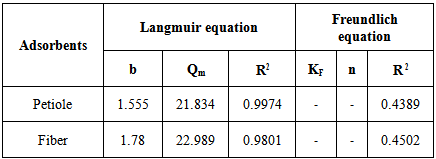 |
| |
|
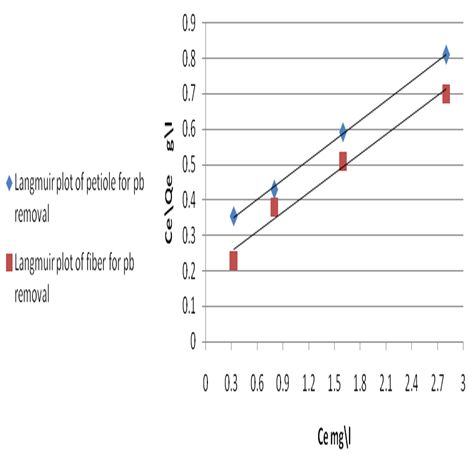 | Figure 5. Langmuir plot of petiole and fiber for Pb removal at 25±2 oC |
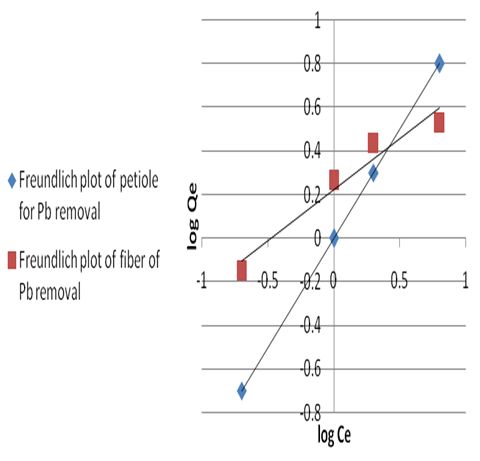 | Figure 6. Freundlich plot of petiole and fiber for Pb removal at 25±2℃ |
Table 9. Amount of Pb(II) uptake by petiole at 25±2℃ with proper calculations for the application of Langmuir and Freundlich equations 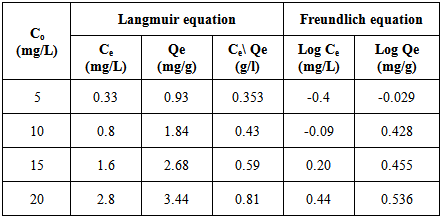 |
| |
|
Table 10. Amount of Pb(II) uptake by fiber at 25±2℃ with proper calculations for the application of Langmuir and Freundlich equations 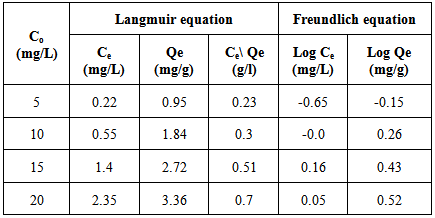 |
| |
|
3.2.3. The Adsorption Isotherm of Pb(ІІ) on Petiole and Fiber at Different Temperature
The adsorption of Pb(II) on the petiole and fiber surfaces were studied at room temperature (25℃) as well as at the other two temperatures (30.0 and 40.0) ℃, the initial concentration of heavy metal 5mg\L, adsorbents dosage (0.5)g and pH (6.5-7). The obtain results are shown in Table 11 and Figure 7; the plot of removal percentage versus (Co), to obtain the general shape of the adsorption isotherms which represents the isotherms of metal ions on the petiole and fiber at different temperatures.Table 11. Removal percentage of Pb(ІІ) ions by petiole at different temperatures 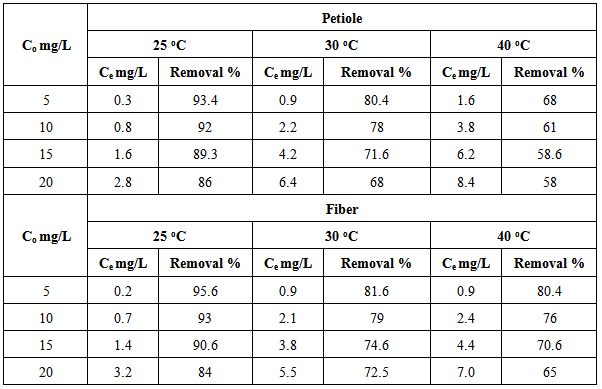 |
| |
|
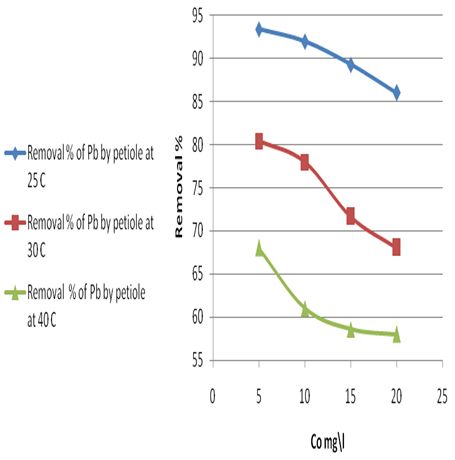 | Figure 7a. Removal percentage of Pb(ІІ) ions by petiole surface at different temperatures |
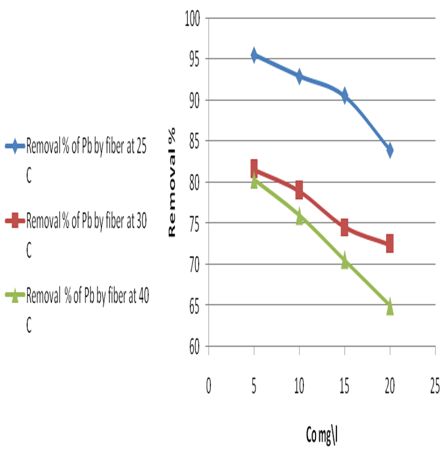 | Figure 7b. Removal percentage of Pb(ІІ) ions by fiber surface at different temperatures |
It was noticed a decrease in the amount of metal ions (which was adsorbed on petiole and fiber surfaces) with the increase of temperature and that adsorption process appeared to be exothermic. Thus, the low temperatures are the favorite for adsorption of metals ions on petiole and fiber.The decrease in adsorption of metal ions with the increase of temperature could be explained by the fact that the thickness of the boundary layer decreases with the rise in the solution temperature, due to the increased tendency metal ions to desorb from the solid phase to the liquid phase. It is well understood that lignocelluloses materials such as petiole are not pure polymers. Their main constituents are cellulose, lignin, hemi cellulose and extractives where chemical and physical forces interact with the ionic lead to the result complexity. Consequently, the removal of metal ions can be governed by any four basic processes; adsorption, absorption, ion exchange and chelating. However this change in removal rate of Pb(II) metal ions could be attributed to one or more of these different processes, the most effected method may be ions exchange and adsorption.
3.3. Thermodynamic Studies
Thermodynamic parameters such as the free energy (ΔGo ), enthalpy (ΔHo ) and entropy (ΔSo) changes during adsorption can be evaluated from the Equations (7, 8 and 9 ) below, as in [13]; where Kc is the equilibrium constant, Ce is the equilibrium concentration in solution (mg.L-1) and CAe is the solid-phase concentration at equilibrium(mg.L-1). ΔGo, ΔHo and ΔSo are changes in Gibbs free energy (kJ.mol-1), enthalpy (kJ.mol-1) and entropy (J.mol-1K-1), respectively. R is the gas constant (8.314 J.mol-1.K-1) and T is the temperature in (K); Table 12. Plots of log Kc versus 1/T are used to calculate the values of ΔHo from the slope and ΔSo from the intercept resulted from plots. The ΔGo values were obtained using Equation (8).  | (7) |
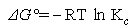 | (8) |
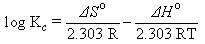 | (9) |
Table 12. Effect of temperature on the thermodynamic equilibrium constant for adsorption of metal ions on the petiole and fiber surfaces 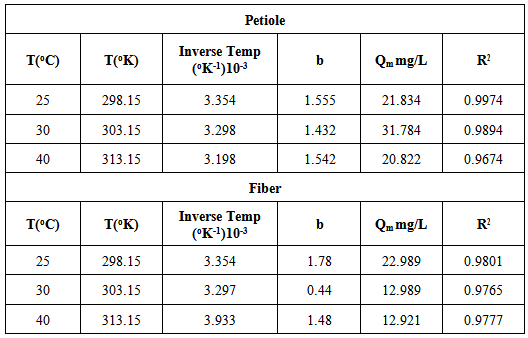 |
| |
|
Table 13 contains the basic thermodynamic values of metal ion adsorption on petiole and fiber surface at different temperatures. The enthalpy values of adsorption (ΔHo) of (metal-petiole) and (metal- fiber) systems were found to possess a negative value indicating an exothermic adsorption process. The negative values of entropy (ΔSo) for the adsorption of metal ion on the adsorbents indicates that the adsorbed species are more ordered on the surface, while the change in the free energy (ΔGo) value of metal ions adsorption on adsorbents was found to possess a negative value indicate that the process is feasible and the adsorption is spontaneous in nature.Table 13. Values of thermodynamic functions for the adsorption of metal ion on the petiole and fiber surfaces at different temperatures 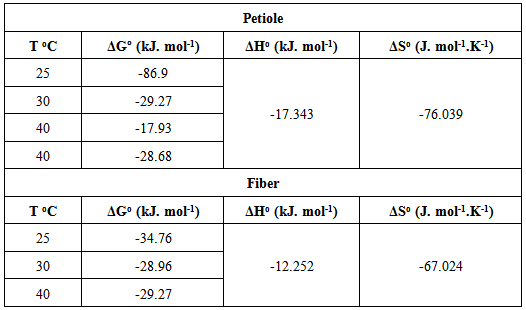 |
| |
|
3.4. Kinetic Studies
The kinetic adsorption data were investigated to understand the dynamic of the adsorption process in terms of the order of the rate constant. Assuming a pseudo-first-order kinetic, the Lagergren eq., as in [14] was used for the studied. This equation can be written as follows: | (10) |
where Qe and Qt the amount of metal ion adsorbed at equilibrium and at time (t) respectively. The plot of ln(Qe-Qt) versus time (t) gives a straight line and pseudo first order rate constant K min-1 can be calculated from the slope of that line since initial concentration of heavy metal was 5 mg/L and is given by Figure 8.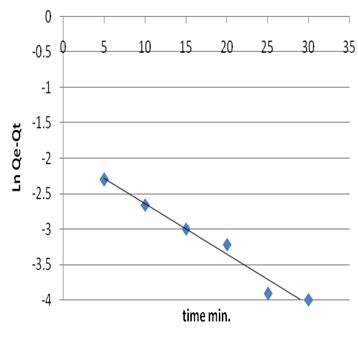 | Figure 8a. The applicability of the first order kinetic model to metal ion adsorption on petiole |
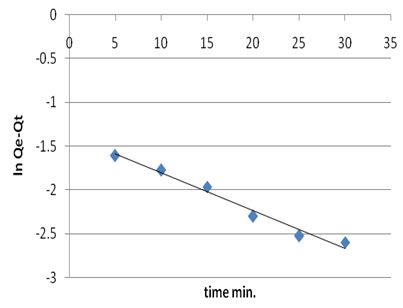 | Figure 8b. The applicability of the first order kinetic model to metal ions adsorption on fiber |
The kinetic constants and correlation coefficients of this model were calculated and are given in Table 14. The values of R2 for the pseudo-first order for most adsorption systems are satisfactory. Therefore, it has been concluded that the pseudo-first order adsorption model is more suitable to describe the adsorption kinetics of metal ions on adsorbents.Table 14. Kinetic parameters for adsorption of heavy metal on palm petiole and fiber surfaces at 25±2 ℃ 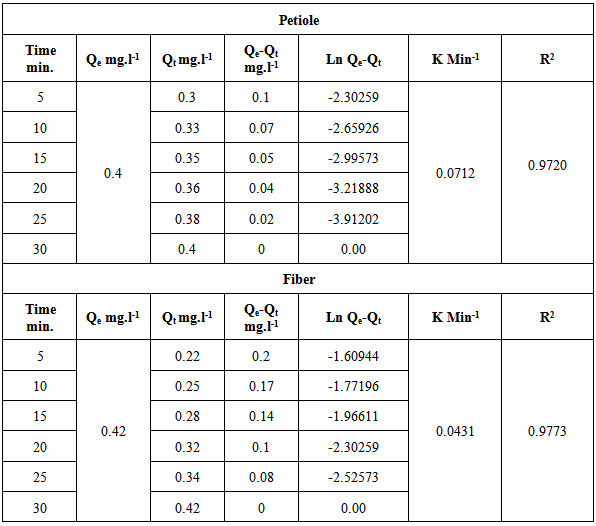 |
| |
|
4. Conclusions
The present work has described the use of leaf base (petiole) and fiber of date palm as an adsorbent as these agricultural materials are locally available in Iraq, economic and eco-friendly. It was found that petiole and fiber of date palm is an excellent adsorbent for removal of lead from aqueous solution and waste water using Batch adsorption technique. The adsorption equilibrium time was 30 and 40 min. for heavy metal by leaf and fiber respectively. The results indicated that over pH range (6.5–7.0) there is an increase optimum of adsorption amounts for Pb(II) ions. The study showed that (0.5 g) of adsorbent weight is very ideal to obtain at best adsorption for studied metal ions. The isotherms of metal ions on the two adsorbent obeyed Langmuir relation better than Freundlich. Mostly, the thermodynamic parameters show the spontaneous and exothermic adsorption processes of heavy metal ions onto the surfaces of natural adsorbents. The study further demonstrated that the adsorption process was on first order in kinetic. Adsorbents which are used in the study can be used successfully for removal of lead from waste water, the maximum removal efficiency percentage was observed up to (98.4) and (96.5) by leaf base and fiber respectively. The maximum adsorption capacity was obtained for petiole and fiber at 21.83 and 22.98 mg/L respectively.
References
[1] | Kassim, T.I.; Al-Lami, A.A.; Al-Saadi, H.A. and Abood, S.M. 1995, Seasonal and spatial variation of epipelic and epileptic algae in Qadisia lake in Iraq. Basrah J. Sci., 13(1), 1-10. |
[2] | WHO 1984, Guidelines values for drinking water quality//Vol. 1, Recommendation, Word Health Organization: Geneva; p8. |
[3] | Ellis, J.B.; Shutes, R.B.; Revitt, D. M. and Zhang, T.T. 2007, Use of macrophysics for pollution treatment in urban wetlands .Res. Conser. Rec., 11, 1-12. |
[4] | EPA (Environmental Protection Agency), 2012, Parameters of Water Quality, Interpretation and Standard, ISBN 1-84096-015-3, Ireland. |
[5] | Devkov, Z. and Krstic, S. 2002, Use of algae for monitoring of heavy metals in the river Vardar, Macedonia. Medit. Mar. Sci., 99, 112. |
[6] | Lind, G.T. 1998, Hand book of common methods in limnology, 2nd Ed., London. |
[7] | Al-Rekabi, M. T. K. 2013, Environmental pollutants in Euphrates River-Determination, Removal and thermodynamic – kinetic studies, Ph.D. Thesis, Department of Chemistry, College Of Science, University of Baghdad. |
[8] | Zhang, L.; Zhao, L.; Yu, Y. and Chen, C., 1998, Removal of lead from aqueous solution by non-living Rhizopus nigricans. Water Res., 32: 1437–1444. |
[9] | Zhou, J. L.; Kiff, R. J. 1991. The uptake of copper from aqueous solution by immobilized fungal biomass. J. Chem. Technol., 52, 317–330. |
[10] | Zulkali, M. M. D.; Ahmed, A. C. and Norulakmal, N. H. 2006, Oriza sativa husk as heavy metal adsorbent: optimization with lead as model solution. Biores. Technol., 97, 21–25. |
[11] | Imamoglu, M. and Tekir, O. (2008), Removal of copper (II) and lead (II) ions from aqueous solutions by a new precursor hazelnut husks. Desalination, 228 (1-3), 108-113. |
[12] | Tsai, W. T. and chen, H. R. (2010), Removal of Malachite Green from aqueous solution using low-cost chlorella-based biomass. j. Hazard. Mater., 175 (1-3), 844-849. |
[13] | Sujana, M. G., Pradhan, H. K. and Anand, S. (2009), Studies on sorption of some geometrical for fluoride removal from aqueous solutions. J. Hazard. Mater., 161(1), 120-125. |
[14] | Lagergren, S. (1898), About the theory of so called adsorption of soluble substances, der sogenannten adsorption geloster stoffe kungliga Svenska Vetenskapsalka de Miens Handlingar, 24, 1-39. |