Elahe Hosseini Nezhad, Mohammadali Ghorbani, Mehrnoosh Zeinalkhani, Alireza Heidari
Institute for Advanced Studies, Tehran, 14456-63543, Iran
Correspondence to: Alireza Heidari, Institute for Advanced Studies, Tehran, 14456-63543, Iran.
Email: |  |
Copyright © 2012 Scientific & Academic Publishing. All Rights Reserved.
Abstract
Here we aim to absorb yellow GX anionic pigments through Mg-Fe layered-double-hydroxide nanoparticles, which were synthesized from Iron(III) nitrate nonahydrate and Manganese(II) nitrate hexahydrate with a molar ratio of Mg/Fe=2:1 by a hydrothermal process (>100℃). The synthesized nanoparticles were studied using the Fourier transform infrared spectroscopy, X-ray diffraction, and scanning electron microscope. The obtained data were analyzed by the Debye–Scherrer method. The absorption of yellow GX anionic pigments by these nanoparticles was investigated through UV-Vis photometry at different pH, reaction time, and temperatures. The results suggest that a rise in temperature leads to a gradual increase in absorption.
Keywords:
Debye–Scherrer Method, FTIR Spectroscopy, Mg-Fe LDH, SEM, Yellow GX Pigment, XRD
Cite this paper: Elahe Hosseini Nezhad, Mohammadali Ghorbani, Mehrnoosh Zeinalkhani, Alireza Heidari, A New Technique to Absorb Yellow GX Anionic Pigments, American Journal of Chemistry, Vol. 3 No. 1, 2013, pp. 6-9. doi: 10.5923/j.chemistry.20130301.02.
1. Introduction
Water sanitation is a significant issue in the public health management. Most industrial wastes severely damage the aquatic ecosystem. Such wastes consume a considerable amount of oxygen to be neutralized, and decrease the water's dissolved oxygen level. On the other hand, most of these wastes such as heavy metals, mercury, lead, copper, pigments, and so forth are toxic and cause aquatic poisoning. The water treatment method depends on the type of contaminant. Pigments in sewage are classified as an environmental contaminant. Such materials are being pumped into the sewage system by various industries like textile, tanning industry, paper industry, printing industry, pharmaceutical industry, and cosmetics industry[1]. Among them, the sewage of the textile industries is considered one of the most challenging types of sewage due to azo compounds. The azo colors are the most common type of synthetic colors, comprising the main part of textile sewage. Azo compounds can contaminate the natural ecosystems like soil, surface water, and groundwater[2]. In order to protect the environment and minimize the deleterious effects of various residues, we should find and employ efficient and cost-effective solutions with least side effects. Pigments are hardly filtered out in the sewage recycling processes, which include: absorption by activated carbon and LDHs, chemical oxidization by substances like chlorine, ozone, and peroxide, ion exchange, electrochemical treatment, biological treatment methods, and absorption by absorbents such as natural zeolite, and montmorillonite. The use of LDHs to filter out pigments from sewage is increasing, owing to their ability to exchange ion, high flexibility, low cost, and simple operation. LDH is one of the most effective absorbents and also one of the natural or synthetic absorbents with a wide range of applications and properties. The mineral absorbents are an appropriate candidate to solve a major part of the environmental problems[3].The LDHs is a group of mineral compounds whose structure can be described based on the layer structure of brucite[
]. The layers of brucite that are accumulated are electrically neutral and the ion
on each layer is surrounded by six
ions, such that the magnesium cation is placed in the center of an octagon resulting from the hydroxide ions (Figure 1).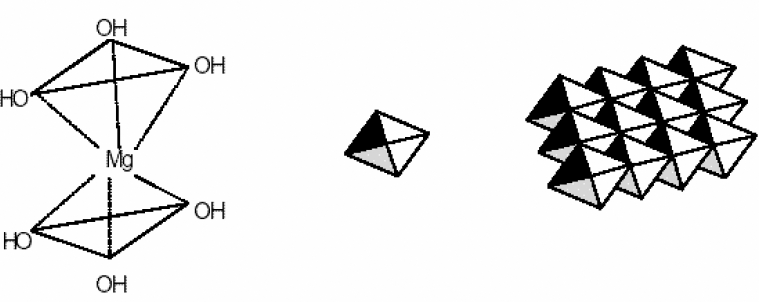 | Figure 1. Brucite octagonal and layer structures |
LDHs can be synthesized by a change in the brucite structure and substituting a trivalent cation like ferric or iron (III) for a part of magnesium ions. In this case, the formulation of the new layer compound is
and the layers are positively charged. The anions (
) such as
,
,
and so forth can be placed between two layers to neutralize the positive charge of the layers and to stabilize[4]. Moreover, the water molecules can be placed between layers through bonding hydrogen with the groups OH Therefore, the LDH general formulation can be introduced as

where n represents the number of water molecules and
(it means that the ratio of the bivalent to trivalent metal can be between 1 and 5).
and
represent the other cations can be used to synthesize LDHs. The LDHs show numerous properties through the ion exchange process. The amount of present anions depends on the charge of hydroxide layers, which can be easily controlled by different ratios between
and
during the synthesis[5, 6]. Much attention to the metallic LDHs leads to a wide range of applications in chemical catalysts, optical catalysts, anion exchange, pharmaceutical chemistry, sensors, plastic additives, absorbents, flame prorogation, batteries, water treatment, absorbing heavy metals in the soil, absorbing halogens, and precipitating the heavy metals’ ions in the sewages[7]. These materials’ structure is plotted in Figure 2.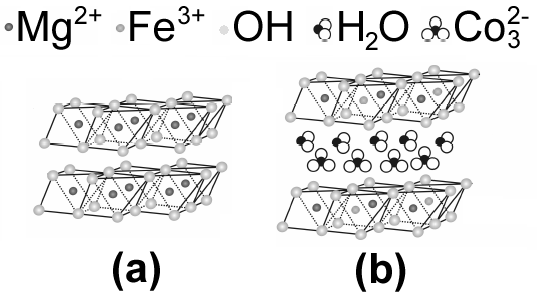 | Figure 2. (a) Brucite layer structure; (b) LDH structure |
In this investigation, for the first time the Fe-Mg LDH nanoparticles (
) were synthesized through coprecipitation (CPT) as the absorbent of yellow GX (YGX) pigments. Then, the effective parameters (like the equilibrium time, pH, and temperature) to absorb YGX pigments from the aqueous solution were studied. The acidic YGX pigment is one the azo colors widely used in the industries of textile, leather, plastic, ink, and pigment. Figure 3 shows the formulation of YGX pigment.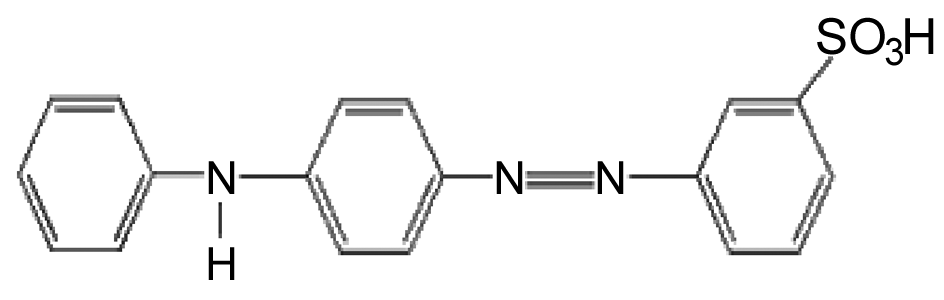 | Figure 3. Yellow GX pigment formulation |
2. Experimental
2.1. Synthesis of Fe-Mg LDH Nanoparticles
The Fe-Mg LDHs were prepared through the hydrothermal method (>100℃). A solution of
and
with the Mg/Fe molar ratio=2 was prepared in the solvents of deionized water, 1-propanol, and ethylene glycol with the ratio 1:2:3. Afterwards, it was mixed with a 0.5-molar soda solution under nitrogen gas such that the pH remains about 9.5. The obtained gelatinous material was heated at 160℃ for 24h under the nitrogen gas. This mixture was centrifuged and washed several times, and dried in the oven in the end.
2.2. Pigment Absorption
To absorb pigment by LDH as an ion exchange interaction, 50 mg of the prepared Fe-Mg LDH nanoparticles was added to 50 ml of pigment dissolved in the deionized water with the concentration 50 mg/lit. The container containing this mixture was mixed at 35, 45, and 55℃ for a specific time. The YGX solution’s pH was adjusted from 2 to 12 by NaOHand
. After a specific reaction time (at least 20 min) that an amount of YGX being absorbed in LDH, the YGX particles remained in the solution (not absorbed by LDH) werefiltered out by centrifugation and the amount of not-absorbed YGX particles was studied by an UV spectrophotometer.
2.3. Investigation Methods
The FTIR spectroscopy was employed to gain the qualitative data of the functional groups on such nanoparticles (
). Furthermore, the particle average size was obtained from the Debye–Scherrer equation and the samples’ crystalline property was observed in the XRD spectra peaks. The morphology of the nanoparticles was studied using an SEM. The ability of these nanoparticles to absorb YGX pigments was investigated through UV-Vis photometry at the wavelength 300-600 nm.
3. Results and Discussion
3.1. Investigation of 
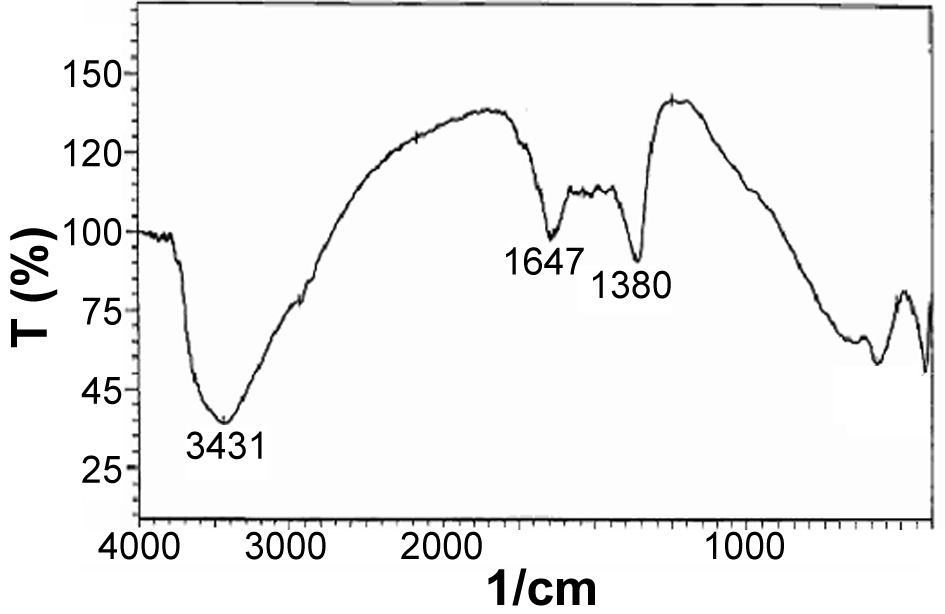 | Figure 4. IR spectrum of Fe-Mg LDH synthesized at 160℃ with ratio 2:1 in the water and alcohol solvents |
The FTIR spectrum of the synthesized samples was taken to study the vibration of nanoparticles. The peak in the wavenumber
is caused by the OH stretching vibrations of the hydroxyl groups on the layer surface and or the interlayer water molecules; the peak in the wavenumber
pertains to the OH bending vibrations of the water molecules[8]. The spectra observed in the low wavenumbers (
) appeared as the lattice vibrational spectra such as MOH and OMO[9]. The vibrational spectrum of the nitrate group was observed around
(Figure 4).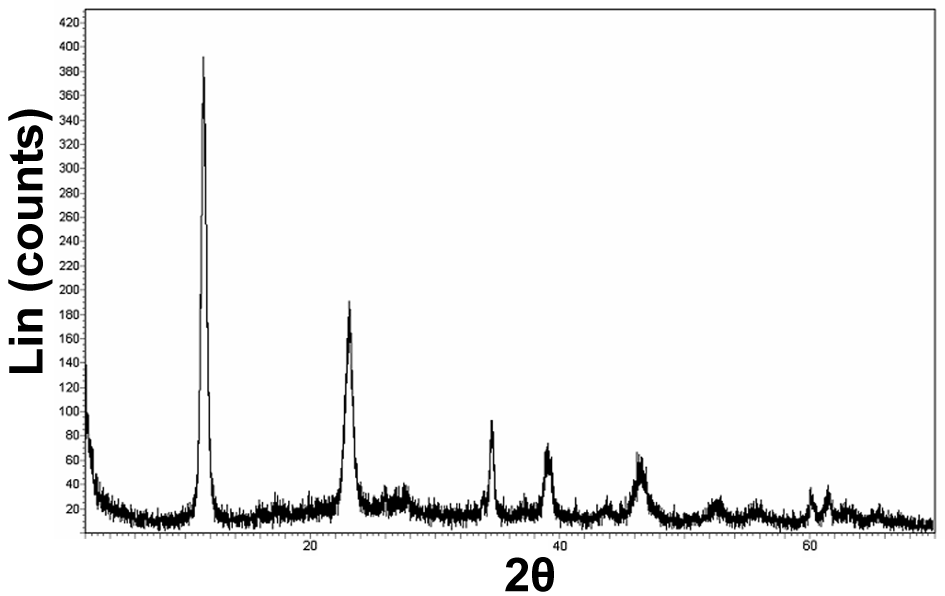 | Figure 5. XRD spectrum of Fe-Mg LDH synthesized at 160℃ with ratio 2:1 in the water and alcohol solvents |
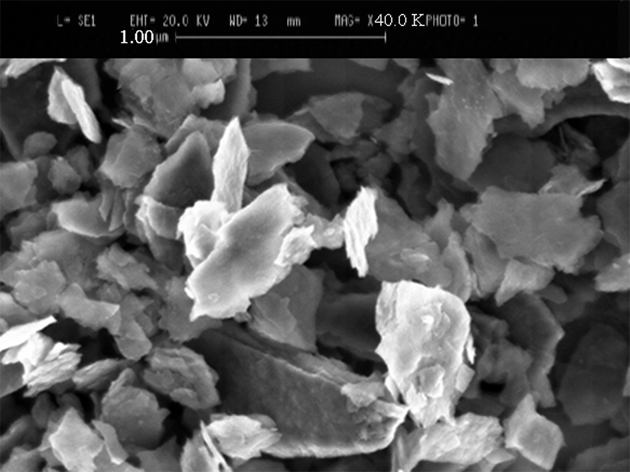 | Figure 6. SEM image of Fe-Mg LDH synthesized at 160℃ with ratio 2:1 in the water and alcohol solvents |
The XRD method was employed to study the samples’ crystalline properties. The X-ray diffraction patterns with copper K-alpha source and the wavelength 0.154 nm were recorded within
. The peaks around 11, 22, 34, and 60° are the LDH peaks that respectively pertain to the crystal plates (003), (006), (009), and (110) (see Figure 5). The average particle size D (nm) is obtained from the Scherrer equation below: | (1) |
where
,
is the wavelength with copper K-alpha source,
the width of the peak at half peak height (radian), and
the diffraction angle (°)[10]. The peak in
pertains to the diffraction of the crystal plates (003) that we obtained the average particle size 28.55 nm from the peak width at half peak height.The SEM technique was utilized to study the morphology of the nanoparticles. As can be seen in Figure 6, the synthesized Fe-Mg LDH nanoparticles are plate-like.
3.2. Investigation of the Effect of pH, Reaction Time, and Temperature on YGX Absorption
The parameter pH is usually considered a significant factor in controlling the amount absorption in aqueous solutions. Figure 7 shows the effect of
under the room conditions for 48 h, the absorbate concentration 50 mg/lit, and the absorbent amount 50 mg. This amount represents the amount of the absorption of YGX pigments remained in the aqueous solution (it means that this amount is measured after the absorption process). As can be seen in this figure, increasing the amount of pH increases the amount of absorption. The maximum is at pH=6 and it decreases for pH>6. Therefore, the best condition for YGX absorption by the Fe-Mg LDH nanoparticles is an acidic or neutral medium. Hence, the other experiments were carried out in pH=6.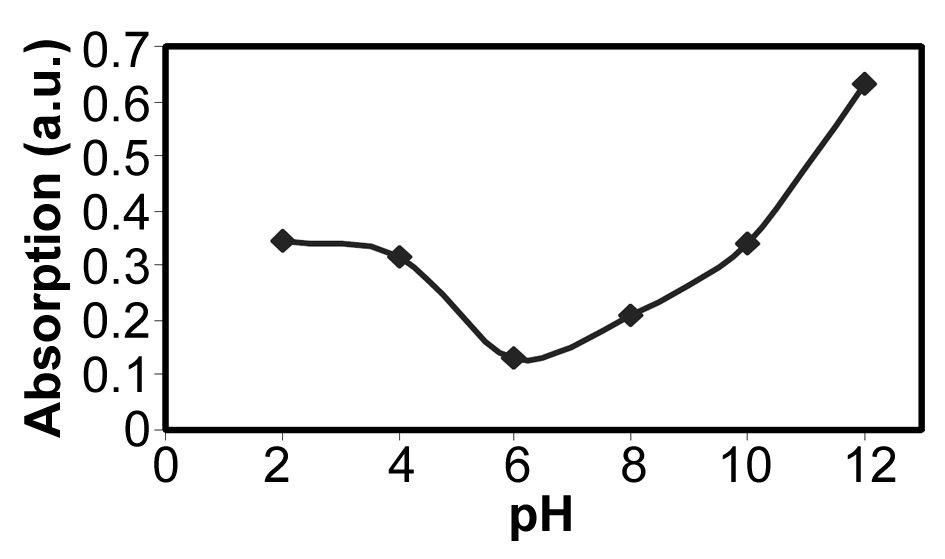 | Figure 7. pH effect on the YGX absorption remained in the aqueous solution in 48 h in the room temperature, ,  |
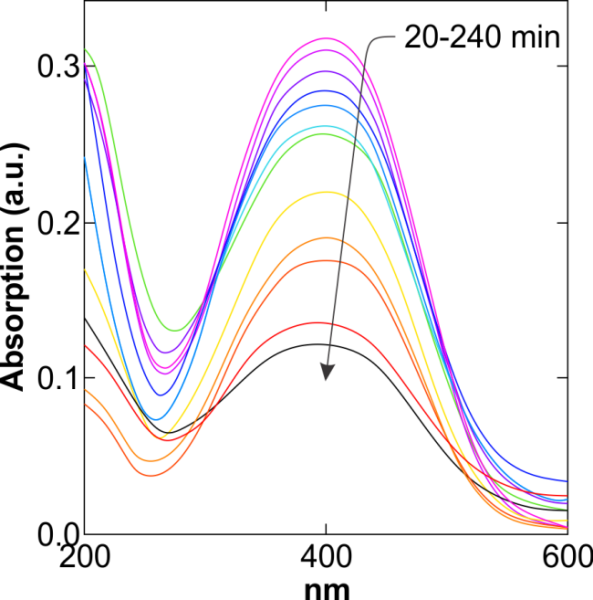 | Figure 8. UV-Vis spectra of YGX pigments remained in the aqueous solution vs. length (T=25℃, pH=6, , , and sampling every 20 min) |
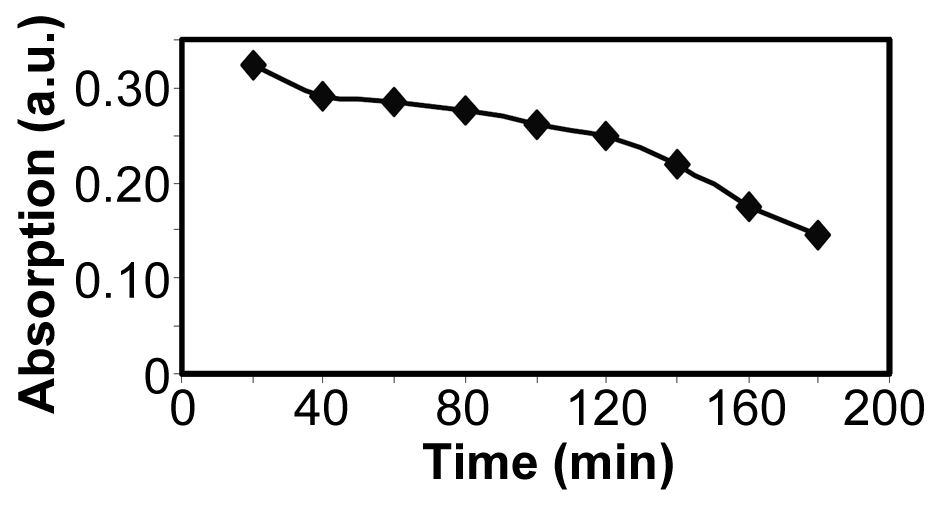 | Figure 9.Absorption of YGX remained in the aqueous solution vs. time (T=25℃, pH=6, , and ) |
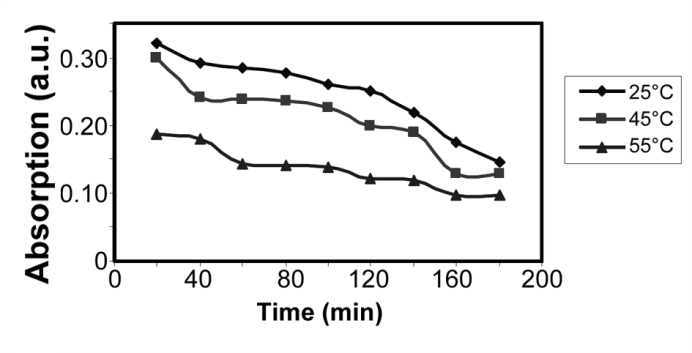 | Figure 10.Absorption of YGX remained in the aqueous solution vs. time for different temperatures (pH=6, , and ) |
The effect of the reaction time on the YGX absorption bythe Fe-Mg LDH nanoparticles was investigated over a range from 20 to 240 min under the room temperature, pH=6, and
. The results are plotted in Figures 8 and 9. As evident, increasing the reaction time increases the YGX absorption in the aqueous solution. It means the remained amount of YGX pigments declines.Temperature is a significant factor in the absorption process. Figure 10 shows the absorption curves for different single-temperature conditions. As can be observed, a rise in the temperature leads to a gradual increase in the amount of absorption as a result of the fact that the interaction between absorbent and absorbate is endothermic. The best results were achieved at 55℃. This endothermicity is due to the heterogeneous oxide surfaces.
ACKNOWLEDGMENTS
The work described in this paper was fully supported by grants from the Institute for Advanced Studies of Iran. The authors would like to express genuinely and sincerely thanks and appreciated and their gratitude to Institute for Advanced Studies of Iran.
References
[1] | Ramalho, P. A., Scholze, H., Cardoso, M. H., Ramalho, M. T., Oliveira-Campos, A. M., "Improved conditions for the aerobic reductive decolorisation of azo dyes by Candida zeylanoides", Enzyme Microb. Technol. (31) 848-854, (2002). |
[2] | McMullan, G., Meehan,C., Conneely, A., Kirby, N., Robinson, T.,Nigam, P., Banat, I., Marchant, R., Smyth, W., "Microbial decolourisation and degradation of textile dyes. Applied Microbiology and Biotechnology", (56) 81.87, (2001). |
[3] | Leroux, F., Besse, J.P., "Polymer interleaved layered double hydroxide: a new emerging class of nanocomposites", Chem. Mater. (13) 3507.3515, (2001). |
[4] | Orthman, J., Zhu, H.Y., Lu, G.Q., "Use of anion clay hydrotalcite to remove colored organics from aqueous solutions", Sep. Purif. Technol., 31, 53.59, (2003). |
[5] | Cavani, F., Trifiro, F., Vaccari, A., "Hydrotalcite-type anionic clays: preparation, properties and applications" ,Catal. Today (11) 173.301, (1991). |
[6] | Carpani, I., Berrettoni, M., Blaring, B., Giorgetti, M., Scavetta, E., Tonelli, D., "Study on the intercalation of hexacyanoferrate (II) in a Ni, Al based hydrotalcite", Solid State Ionics, (168)167.175, (2004). |
[7] | Rives, V., "Layered Double Hydroxides: Present and Future" Nova Science Publishers, Inc., New York, 251- 411, (2001). |
[8] | Abello, S., Medina, F., Tichit, D., Ramirez, J.P., Groen, J.C., Sueiras, J.E., Salagre, P., Cesteros, Y., "Aldol condensations over reconstructed Mg.Alhydrotalcites: structure.activity relationships related to the rehydration method", Eur. J. Chem., (11) 728.734, (2005). |
[9] | Cullity, B.D., Stock, S.R., "Elements of X-Ray Diffraction", 3rd ed., PEARSON Prentice Hall, Nj , Lecture 8, (2001). |
[10] | Zhe-Ming, N., Sheng-Jie, X., Li-Geng W., Fang-Fang X., Guo-Xiang P., "Treatment of methyl orange by calcined layered double hydroxides in aqueous solution: Adsorption property and kinetic studies", J.colloid and interface Sci. (316) 284-291, (2007). |