R. Suhas1, L. P. Ravi Kumar1, A. Ramesha Baba2, D. Channe Gowda1, N. M. Made Gowda3
1Department of Studies in Chemistry, Manasagangotri, University of Mysore, Mysore 570 006, India
2Postgraduate Department of Chemistry, Maharani’s Science College for Women, Mysore, India
3Department of Chemistry, Western Illinois University, 1 University Circle, Macomb, IL 61455, USA
Correspondence to: D. Channe Gowda, Department of Studies in Chemistry, Manasagangotri, University of Mysore, Mysore 570 006, India
N. M. Made Gowda, Department of Chemistry, Western Illinois University, 1 University Circle, Macomb, IL 61455, USA.
Email: |  |
Copyright © 2012 Scientific & Academic Publishing. All Rights Reserved.
Abstract
Four peptides (Ps) with varying chain lengths, VP, GVP, VGVP, GVGVP, and a poly pentapeptide (PPP or poly(GVGVP) have been synthesized by a classical solution phase method and characterized. Oxidations of these peptides, including their constituent amino acids (AAs), V and P, have been studied using the oxidant, quinolinium dichromate (QDC), in perchloric acid medium at 25℃. Each reaction has been monitored spectrophotometrically at the λmax of 440 nm. The reactions follow an identical kinetics with the following general, experimental rate law: rate = k′ [QDC][substrate][H+], where substrate = AA/P/PPP. Effects of dielectric constant of the medium and the added salts have been studied. The reaction stoichiometry has been determined and the products have been characterized. The dependence of the reaction rate on temperature has been determined and the activation parameters have been evaluated. A plausible mechanism has been envisaged based on the kinetic results. An apparent correlation has been noted between the rate of oxidation and the length of the peptide chain. The polymer, poly(GVGVP), has the slowest rate as compared to peptides, ranging from dipeptide to pentapeptide, which in turn have slow rates than their individual amino acid constituents. This is consistent with the dependence of the reaction rate on chain length.
Keywords:
Elastin Polymer, Oxidation, Quinolinium Dichromate, Mechanism, Rate Law
1. Introduction
Oxidative stresses/reactions play an important role in a variety of biochemical events ranging from normalmetabolism to ageing and disease processes[1,2]. Peptides and proteins represent major targets for modification in these reactions and the identification of sites and structure of modifications may lead to a mechanistic understanding and approach for prevention. The changes in proteinconformations due to oxidative damage are often related to changes in the hydrophobicity of the protein sequences. Thisphenomenon is associated with the physiological conditions of protein modifications and is necessary to understand the mechanism involved in biological processes. Therefore, the protein-based polymers of elastin were chosen as a model system to identify the amino acid residues, which are sensitive to the metal-catalyzed oxidation. These protein-based polymers have their origin in repeating sequences of the mammalian elastic protein, elastin[3,4].The most prominent repeating sequence occurs in bovine elastin, which can be represented as( Val1-Pro2-Gly3-Val4-Gly5 )n , where n is eleven without a single substitution. Another repeating sequence was found in porcine elastin (Val1-Pro2-Gly3-Gly4)n , which has not been found to occur with n greater than 2 without substitution[5]. The monomers, peptides, oligomers, and polymers of these repeats have been synthesized and conformationally characterized[6]. These polymers have a number of medical and non-medical applications[7,8]. Extensive studies have been reported from our laboratory on the kinetics of oxidation of amino acids, peptides and their polymers with various oxidants including transition metal ions with higher oxidation states[9-13]. The kinetics and mechanistic studies of oxidation of various substrates by Cr(VI) have been well explored and found that chromic acid as one of the most versatile oxidizing agents available. Recent development of newer Cr(VI) reagents[14-19] for the oxidation of organicsubstrates continues to be a subject of interest. The reagent employed in the present study, quinolinium dichromate(C9H7N+H)2Cr2O72- (QDC), has been found to be a novel oxidant [20] that deserves further evaluation. This prompted us to investigate the mechanisms involved in the oxidation of amino acids (AAs), peptides (Ps), and polypentapeptide (PPP) as constituents of elastin sequences with varying chain length by QDC. Since chromium is one of the essential elements needed for proper metabolism of insulin, protein, fat and carbohydrate, Cr(VI) taken up by the cells undergoes reduction to form its trivalent form through intermediate oxidation states, Cr(V) and Cr(IV). With this perspective in mind, we have been exploring the oxidative behaviors of transition metal ions toward amino acid monomers, peptides and polymers of repeating sequences of elastin.
2. Experimental
Unless otherwise stated, all the amino acids used except glycine were of L-configuration. All t-butoxycarbonyl amino acids, 3-ethyl-1-(N,N-dimethyl)aminopropylcarbodiimide (EDCI), 1-hydroxybenzotriazole (HOBt) and trifluoroacetic acid (TFA) were purchased from Advanced Chem. Tech. (Louisville, KY). N-methyl morpholine (NMM) was purchased from Sigma Chemicals Co. (St. Louis, MO). Quinoline, chromium trioxide and perchloric acid were from Merck Chemicals (Mumbai, India). All solvents and reagents used were of analytical grade. The progress of the reaction was monitored by TLC on a glass coated with silica gel with the solvent system of chloroform/methanol/acetic acid in the ratio, 95:5:3 (v/v/v). The synthesized peptides were characterized by the 1H-NMR, M.P., elemental and HPLC amino acid analysis data.
2.1. Peptide Synthesis and Polymerization
Peptides of elastin sequences were prepared by theclassical solution phase method involving a stepwise approach reported earlier[20,21]. In brief, the Boc group was used for temporary Nα-protection and its removal was achieved with 4N HCl in dioxane. The C-terminal carboxyl group was protected by the benzyl ester and its removal was effected by hydrogenolysis using HCOONH4 as hydrogen donor and 10% Pd on carbon as the catalyst[22].
2.1.1. Synthesis of Poly(GVGVP)
A solution of Boc-GVGVP-OH (2.64g, 5.00 mmol) was subjected to deblocking with TFA (26 mL) to obtain the TFA salt of GVGVP. The solution of TFA salt (1.0 M) in DMSO (10 mL/g of peptide) was polymersized for 2 days using EDCI (1.91g, 10.0 mmol), HOBt (0.765g, 5.00 mmol) and NMM (0.88 mL, 8.00 mmol) as base[23]. The crude polymer product was dissolved in water, dialyzed using 3500 Da molecular weight cut-off dialysis tubing for 1 week and lyophilized. It was then dialyzed using 50 kDa molecular weight cut-off dialysis tubing for 1 week and lyophilized.
2.2. Preparation of Quinolinium Dichromate
The oxidant, QDC, was prepared by the reaction of quinoline with chromium trioxide, following the procedure reported earlier[20]. Purity of the product was verified by IR, 1H-NMR and elemental analysis. Even though QDC in aqueous solutions appeared to be stable for more than three months, it was prepared afresh and used. All other reagents were prepared from AR grade chemicals. Double distilled water was used throughout the study.
2.3. Preliminary Studies
An absorption spectra of QDC solution, recorded using an Analytic Jena Specord-50 spectrophotometer with quartz cells of 1 cm path length, showed a λmax at 440 nm. Aqueous solutions of QDC were standardized iodometrically.Similarly, aqueous solutions of AA, P, and PPP of desired strength were freshly prepared prior to the experiment. The required acidity was maintained using a standardized HClO4 solution.
2.4. Kinetic Measurements
Kinetic runs were performed under pseudo-first-order conditions of [substrate] >> [QDC]. Mixtures of solutions containing the requisite amounts of substrate, perchloric acid (to maintain a known H+ concentration), and water (to keep the total volume constant) were placed in stoppered boiling tubes. Each mixture was thermally equilibrated in a water bath at 25℃. To initiate the redox reaction in each solution mixture in the tube, was added a known amount of pre-equilibrated, standard QDC stock solution and stirred to give a known overall concentration. The progress of the reaction was monitored for two half-lives by withdrawing aliquots at varying time intervals and measuring the absorbance of the unreacted QDC in each sample at 440 nm. The reaction was quenched appropriately prior to the absorbance measurement. Plots of log (absorbance) vs. time were linear. The pseudo-first-order rate constants, kobs, calculated from the slopes of plots were reproducible within ±3% error.
3. Results and Discussion
3.1. Dependence of the Rate on [QDC] and [Substrate]
The oxidation kinetics was studied under pseudo–first -order conditions of [substrate] >> [QDC] by varying the concentration of QDC at constant [AA/P/PPP], [HClO4], and temperature (Table 1). Plots of log absorbance vs. time were linear indicating that the rate of reaction is first order in QDC. The data in Table 1 show that a significant decrease in reactivity occurs with increasing concentration of Cr(VI). As the concentration of Cr(VI) increases, a progressively smaller portion of the total QDC forms acid chromate ions resulting in decreased rate constant.At constant [QDC], [H+] and temperature, the rate constant increased with an increase in [substrate] (Table 1). Plots of log kobs vs. log [AA/P/PPP] (Figure. 1) were linear with a unit slope confirming the first order dependence of the rate on each [substrate].
3.2. Dependence of the Rate on [H+]
The effect of perchloric acid concentration on the rate was studied in the range 2.5 to 4.0 M while keeping all other conditions the same. The rate increased with an increase in the concentration of HClO4 (Table 1). Plots of log kobs vs. log [HClO4] (Figure. 2) were linear with a unit slope showing the first-order behavior of H+ for all substrates.
3.3. Dependence of the Rate on Cr(III) and added Salts
The effect of varying concentrations of Cr(III) (which is the reduction product of the oxidant) on the rate was investigated at constant [oxidant], [substrate], and ionic strength. The reaction rate was not affected by the presence of Cr(III) (1.00 x 10-4 M – 8.00 x 10-4 M) indicating that it was not involved in a pre-equilibrium with the oxidant. Furthermore, the rate of reaction remained unaffected with increasing ionic strength of reaction medium effected by adding salts such as NaCl (0.010 M to 0.50 M) and NaClO4 (0.010 M to 1.0 M) indicating that the rate determining step does not involve ion-ion interactions. Table 1. Effects of varying concentrations of reactants on the rate in acid solutions at 25℃ 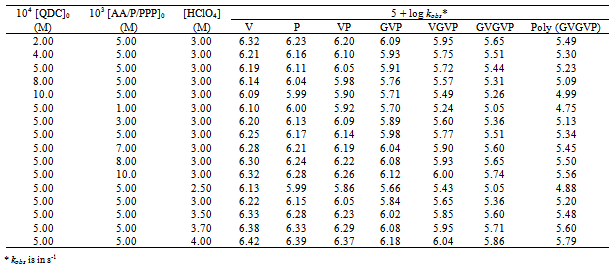 |
| |
|
Table 2. Effect of varying dielectric constant on the reaction rate at 25℃ [AA/P/PPP]0 = 5.00 x 10-3 M, [QDC]0 = 5.00 x 10-4 M, and [HClO4] = 3.00 M 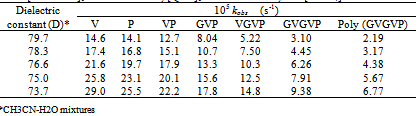 |
| |
|
Table 3. Effect of temperature on the reaction rate for the oxidation of AA/Ps/PPP by QDC in acid solutions [AA/P/PPP]0 = 5.00 x 10-3 M, [QDC]0 = 5.00 x 10-4 M, and [HClO4] = 3.00 M 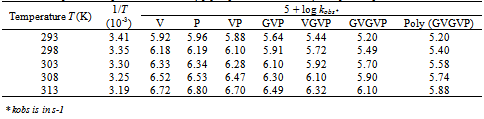 |
| |
|
3.4. Effect of Solvent Composition or Dielectric Constant
The effect of dielectric constant (D) on the rate was studied by varying the composition of the CH3CN-water mixture in the solvent. The rate increased with increasing proportion of CH3CN or decreasing D (Table 2). Plots of kobs vs. 1/D were linear (Figure. 3) with a positive slope suggesting that the relatively less polar transition state as compared to the ground state is stabilized with decreasing solvent polarity (D)[24]. 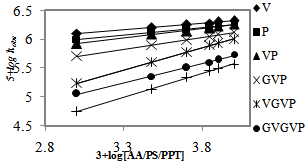 | Figure 1. Effects of [AAs/Ps/PPP] on the reaction rate at 25℃, [QDC] = 5.00 x 10-4 M, and [HClO4] = 3.00 M |
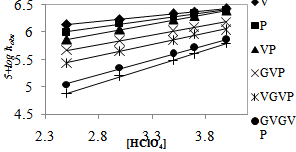 | Figure 2. Effects of [HClO4] on the reaction rate at 25℃, [AAs/Ps/PPP] = 5.00 x 10-3 M, and [QDC] = 5.00 x 10-4 M |
3.5. Kinetic Isotopic Effect
The kinetic isotope effect (kD2O/kH2O = 2.0) on the rate of the acid catalyzed reaction indicates a pre-equilibrium protonation[25,26]. Also, the solvent isotope effect indicates that the hydroxyl group is not involved in a pre-equilibrium or in the rate determining step. The reactions were carried out at different temperatures ranging from 25-40℃ (Table 3). Using Arrhenius plots of log (kobs) vs 1/T (Figure. 4) and Eyring plots of log (kobs/T) vs 1/T (Figure not shown), activation parameters, namely, energy of activation (Ea), entropy of activation (ΔS≠) , enthalpy of activation (ΔH≠) and Gibbs free energy of activation (ΔG≠), were calculated for all substrates, Aas /Ps/PPP (Table 4). The negative ΔS≠ values for all substrates indicate the involvement of a rigid, associated species with a loss of degrees of freedom in the transition state, which might form due to salvation, there by lowering the entropy of activation. Furthermore, each substrate reacts with QDC through the same proposed mechanism. This is supported by the positive values of ΔH≠ and ΔG≠. A plot of ΔS# vs Ea. is linear suggesting that all the substrates, AAs/Ps/PPP, studied follow a similar oxidation mechanism.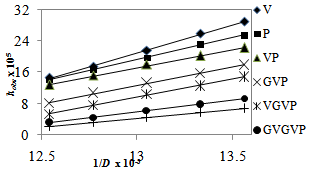 | Figure 3. Effects of dielectric constant (D) on the reaction rate at 25℃, [AAs/Ps/PPP] = 5.00 x 10-3 M, [QDC] = 5.00 x 10-4 M, and [HClO4] = 3.00 M |
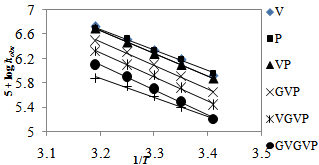 | Figure 4. Arrhenius plots of log kobs vs 1/T: [AAs/Ps/PPP] = 5.00 x 10-3 M, [QDC] = 5.00 x 10-4 M, [HClO4] = 3.00 M |
The acrylonitrile monomer was added to each reaction mixture and kept for 2h in dark in an inert N2 atmosphere at room temperature. It was diluted with methanol. A white precipitate of the polymer product formed clearly indicating the in situ formation of free radicals in the reaction system. Reaction mixtures containing AA/P/PPP (5.00 x 10-4 M), HClO4 (3.00 M) and QDC (5.00 x 10-3 M) were stirred for 24 h at 25℃. The unconsumed QDC was then determined iodometrically to calculate mole ratios. For example, the stoichiometric results indicated that 6 moles of QDC were required to oxidize 3 moles of the tripeptide (GVP) to form three aldehydes (as products of constituent amino acids, G, V, and P), carbon dioxide, ammonium ion and Cr(III) species. The stoichiometric equation is presented in Scheme 1.After the reaction mixture was kept for 24 h at 25℃ for completion of the reaction, the products were extracted with diethyl ether and subjected to column chromatography on silica gel (60-120 mesh) using a gradient elution(dichloromethane to chloroform). Pure aldehydes were estimated to be 72-75%, which was evidenced by their 2,4-DNP derivatives. Furthermore, the products were qualitatively analyzed by IR spectroscopy. The C = O stretching bands between 1730-1728 cm-1 for all oxidation products confirm the presence of aldehydic moiety. The products were thus confirmed (for tripeptide GVP, chosen as a representative of the series for the ease of explanation) as formaldehyde, propionaldehyde and isobutyraldehyde for Gly, Val and Pro, respectively. Ammonia and carbon dioxide were detected by the conventional tests[11,12]. However, it was also observed that the aldehydes determined do not undergo further oxidation under the same reaction conditions as indicated by negative qualitative tests for the corresponding acids. 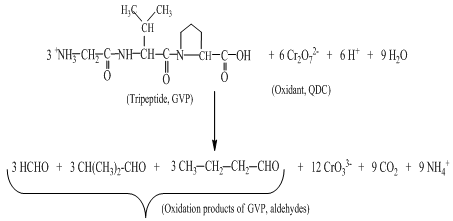 | Scheme 1. Stoichiometric equation for the oxidation of a substrate (GVP is used as a representative of the substrate series) by QDC in acid medium |
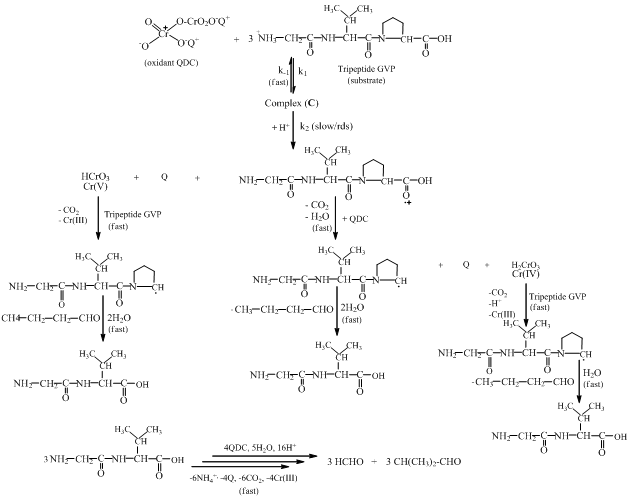 | Scheme 2. A plausible mechanism for the QDC oxidation of GVP, which is used as a representative of the substrate series. Raman spectral studies shown have that at basic pH 11 and acid pH 1 the Cr(VI) ions exist predominantly in the HCrO4- and Cr2O72- forms, respectively[28]. Other spectral studies of aqueous solutions of K2Cr2O7 have proven that Cr2O72- is the predominant hexavalent chromium species[29]. In the present study, since a high acid concentration range of 2.50-4.00 M HClO4 was used, the dichromate ion (or its protonated form) would be the reactive oxidant species |
3.10. Oxidation Mechanism and Rate law
Based on the stoichiometric and kinetic data andliterature studies, a suitable mechanism has been proposed for the oxidation of the tripeptide, GVP (Scheme 2). The observed stoichiometries of G, V, P, VP, VGVP, GVGVP and poly(GVGVP) may be represented in the same way as that shown for GVP (Scheme 1). Michel and Machiroux [27], who studied the Raman spectra of chromate,chlorochromate and dichromate species, have found that the protonated form of chromate ion, HCrO4-, does not exist in aqueous solutions of Cr(VI) compounds. They have also reported that of all the hexavalent chromium species, the only ones present in significant concentrations in dilute mineral acid solutions are HCrO4- and Cr2O72-. The equation (1) below shows the two Cr(VI) ions in equilibrium with each other and with a Kd value of 35.5 suggesting that there will be a relatively higher concentration of Cr2O72- and the dilution of solution favors the HCrO4- formation[27,28]. | (1) |
The first-order dependence of the rate on acid suggests the involvement of a H+ in a fast pre-equilibrium step in Scheme 2. This is consistent with the reports of the involvement of such species in chromic acid oxidations[30,31]. In each case, QDC interacts with the substrate to form a complex C. Then C interacts with H+ in the rate determining (slow step) to form a free radical cation of the amino acid and the intermediate Cr(V). This step followed by subsequent fast steps leads to the formation of end products.In the proposed mechanism, the formation of aldehydic products is envisaged via the reaction of the intermediate peptide free radical. The trend observed in the present study is that, as the length of the peptide chain increases, the rate markedly decreases. Thus, poly(GVGVP) which is a long chain system of the series has the slowest rate of oxidation. On the other hand, the peptides involved in the investigation with differing chain lengths, dipeptide to pentapeptide, have relatively slow rates as compared to their free AA monomers. Thus, AAs which have a simple chemical composition and less charge undergo oxidation at a faster rate. On the contrary, when the molecular complexity is gradually increased, the distance between the functional groups increases resulting in weaker electrostatic interactions between molecules and hence decreasing the rate of oxidation. The proposed mechanism in Scheme 2 below accounts for the observed experimental results.From the slow step, | (2) |
By applying the steady state concept to the intermediate complex C and solving for [C], one gets eq. (3). | (3) |
In Scheme 2, the substrate is represented by the tripeptide, GVP.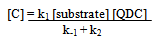 | (4) |
 | (5) |
4. Conclusions
The sensitivity of QDC towards the oxidation of substrates, AAs/Ps/PPP, has been tested. The observed kinetic data reveal that all substrates are susceptible to the oxidative stress under similar conditions with varying rates of reaction. The oxidation follows a first order rate dependence on each of the participants, [substrate], [QDC] and [acid]. Under identical experimental conditions, the rates of oxidation differ. The rate of PPP was slower than that of Ps, which is in turn slower than its free AAs or monomers. The rate change is due to the increased distance between the functional groups and the resultant weaker electronic effects. This shows that the rate of oxidation decreases as the chain length increases. It is clear that the reactive species of Cr(VI) not only oxidizes AA residues, but also their peptides. Thus, the order of reactivities observed was found to be: poly(GVGVP) < GVGVP < VGVP < GVP < VP < P < V.
References
[1] | E. R. Stadtman, Science 257 (1992) 1220 |
[2] | B. S. Berlett, E. R. Stadtman, J. Biol. Chem. 272 (1997) 20313 |
[3] | L. B. Sandberg, J. G. Leslie, C. T. Leach, V. L. Torres, A. R. Smith, D. W. Smith, Pathol. Biol. 33 (1985) 266 |
[4] | H. Yeh, N. Ornstein-Goldstein, Z. Indik, P. Sheppard, N. Anderson, J. C. Rosenbloom, G. Cicila, K. Yoon, J. Collagen Relat. Res. 7 (1987) 235 |
[5] | L. B. Sandberg, N. T. Soskel, J. B. Leslie, Engl. J. Med. 304 (1981) 566 |
[6] | D. W. Urry, M. M. Long, CRC Crit. Rev. Biochem. 4 (1976) 1 |
[7] | D. W. Urry, L. D. Mckee, T. Williams, D. B. Olsen, B. A. Cox, Medical Application of Bioelastic Materials, in: Biotechnological Polymers: Medical, Pharmaceutical and Industrial Applications, Technomic Publishing: Atlanta, Georgia, 1993, p. 82 |
[8] | D. W. Urry, D. T. McPherson, J. Xu, H. Daniell, C. Guda, D. C. Gowda, N. Jing, T. M. Parker, Polymeric Materials Encyclopedia, in: J. C. Salamone (Ed.), CRC Press: Boca Raton, 1996, p. 7263 |
[9] | G. R. Srinivas, K. Abiraj, A. R. Baba, D. C. Gowda, Int. J. Chem. Kinet. 38 (2006) 115 |
[10] | B. K. K. Gowda, H. S. Prasad, K. S. Rangappa, D. C. Gowda, Int. J. Chem. Kinet. 34, (2001) 39 |
[11] | D. C. Gowda, B. K. K. Gowda, K. S. Rangappa, J. Phys. Org. Chem. 14 (2001) 716 and references cited therein |
[12] | B. K. K. Gowda, K. S. Rangappa, D. C. Gowda, Int. J. Chem. Kinet. 34 (2002) 39 and references cited therein |
[13] | K. Abiraj, A. R. Baba, G. R. Srinivas, D. C. Gowda, J. Phys. Org. Chem. 19 (2006) 68 |
[14] | E. J. Corey, G. Schmidt, Tetrahedron Lett. 20 (1979) 399 |
[15] | M. N. Bhattacharjee, M. K. Chaudhuri, H. S. Dasgupta, N. Roy, D. T. Khathing, Synthesis 4 (1982) 588 |
[16] | E. J. Corey, P. Barette, P. A. Magriotis, Tetrahedron Lett. 24 (1985) 5855 |
[17] | F. Ciminale, M. Camporeale, R. Mello, L. Troisi, R. Curci, J. Chem. Soc. Perkin Trans. 2 (1989) 417 |
[18] | G. G. Sharma, M. K. Mahanti, Bull. Soc. Chim. Fr. 128 (1991) 449 |
[19] | S. A. Chimatadar, S. B. Koujalagi, S. T. Nandibewoor, Transitional Met. Chem. 27 (2002) 204 |
[20] | K. Balasubramanian, V. Prathiba, Ind. J. Chem. 25B (1986) 326 |
[21] | A. R. Baba, D. C. Gowda, K. U. Sankar, Ind. J. Chem. 44B (2005) 1487 |
[22] | M. K. Anwer, A. F. Spatola, Tetrahedron Lett. 22 (1981) 4369 |
[23] | A. R. Baba, D. C. Gowda, Lett. Pept. Sci. 8 (2002) 309 |
[24] | E. S. Amis, Solvent Effects on Reaction Rates and Mechanisms, Academic Press, New York, 1966 |
[25] | K. B. Wiberg, Chem. Rev. 55 (1955) 713 |
[26] | C. J. Collins, N. S. Bowmann, Isotopic Effects in Chemical Reactions, Van Nostrans-Reinhold, New York, 1970 |
[27] | G. Michel, R. Machiroux, J. Raman Spectrosc. 14 (1983) 22 |
[28] | H.A.A. Medein, Z. Naturforsch. 109 (1991) 223 |
[29] | M. Creslak-Golonka, Coord. Chem. Rev. 58b (1983) 1201-1205 |
[30] | G. Mangalan, S. Meenakshisundaram, J. Ind. Chem. Soc. 68 (1991) 77 |
[31] | K. B. Wiberg, Oxidations in Organic Chemistry, Academic Press, New York, 1965 |