K. Uzun , E. Çevik, M. Şenel
Department of Chemistry, Faculty of Arts and Sciences, Fatih University, B. Cekmece, Istanbul, 34500, Turkey
Correspondence to: E. Çevik, M. Şenel , Department of Chemistry, Faculty of Arts and Sciences, Fatih University, B. Cekmece, Istanbul, 34500, Turkey.
Email: |  |
Copyright © 2012 Scientific & Academic Publishing. All Rights Reserved.
Abstract
In the present study, Eupergit® C macroporous beads were functionalized with amino triazole. Cu2+ ions were chelated on the triazole modified Eupergit® C (EC), and subsequently, the metal chelated beads were used in the adsorption of invertase. The maximum reaction rate (Vmax) and the Michaelis-Menten constant (Km) were determined for the free and the immobilized enzymes. The characteristics of immobilized invertase such as the temperature activity curve, thermal stability, operational stability, and storage stability were evaluated. The results indicated that triazole functionalized Eupergit® C beads were well applicable for metal adsorption and enzyme immobilization.
Keywords:
Invertase; Immobilization; Eupergit® C; Triazole; Chelating Beads, Enzyme
Cite this paper:
K. Uzun , E. Çevik, M. Şenel , "Invertase Immobilization on a Metal Chelated Triazole-Functionalized Eupergit® C", American Journal of Chemistry, Vol. 1 No. 1, 2011, pp. 16-21. doi: 10.5923/j.chemistry.20110101.03.
1. Introduction
The employment of free enzymes in biotechnological applications is limited owing to the high cost and the impracticality of recovering and separating the enzymes from the reaction medium at the end of the enzymatic pr˚Cess. In order to overcome these difficulties, enzymes were immobilized to obtain a catalyst that would be separable from the reaction medium. Furthermore, the immobilization would provide the enzymes with good properties such as resistance to temperature and denaturants, as well as a longer stability as compared to their free counterparts[7]. Until now, different methods have been developed for enzyme immobilization. Some examples of such methods are cross-linking, covalent attachments, adsorption and entrapment/encapsulation of biological molecules within polymeric gels or carbon paste[8, 13, 1, 4]. Among these methods, the adsorption to a solid support material is the oldest prot˚Col of the physical adsorption method; this method is the most commonly used since it is easiest to perform. The most significant advantages of this method are the stability of enzyme activity after immobilization, and the ability of reuse of the enzyme and support material for different purposes owing to the reversibility of the method. Reversible enzyme immobilization is a very powerful tool that may be considered for solving the cost problem associated with the current methods. Reversible immobilezation provides the possibility of using such enzymes in an immobilized form, thereby providing the advantages of both using immobilized enzymes as well as saving time and cost[12] S. Akgöl and A. Denizli, J. Mol. Catal. B: Enzym. 28 (2004), p. 7.[14, 5].Eupergit® C, which is comprised of epoxy-activated bead polymers formed from a hydrophilic acrylamide with ally glycidyl (epoxide) groups as the active components responsible for binding, is commonly used for enzyme immobilization through different functional groups (amino, sulfhydryl, hydroxyl, phenolic ones) of enzymes[6]. Invertase catalyzes the hydrolysis of sucrose to glucose and fructose, and is known as the invert sugar. However, sucrose crystallizes more readily than invert sugar, and therefore, the latter is widely used in the production of non-crystallizing creams, for example in making jam and artificial honey[11] J.S. Melo and S.F. D'Souza, J. Bi˚Chem. Biophys. Methods 42 (2000), p. 133. Article | PDF (61 K) | View Record in Scopus | Cited By in Scopus (11)[10]. Despite the fact that invertase has little potential for commercial use in its immobilized form, it is still considered to be one of the most studied enzymes since it is a model enzyme for experimental purposes[15].In the present study, triazole functionalized Eupergit® C beads were prepared, chelated with Cu2+ ions and used for the invertase immobilization. Cu2+ ions coordinate with the triazole groups of the Eupergit C®-Tri (EC-Tri) beads and the enzyme binds the beads via chelated metal ion. The protein adsorption capacity, coupling efficiency, Michaelis-Menten kinetic constants (Km and Vmax), and the reuse and the storage stability of the free and the immobilized enzymes were evaluated. | Figure 1. Modification of Eupergit C® with aminotriazole. |
2. Materials and Methods
2.1. Materials
Invertase (β-fructofuranosidase) type V (EC No.3.2.1.26) was purchased from Sigma and used as received without further purification. Eupergit C® was kindly donated by Röhme GmbH & Co., Degussa (Darmstadt, Germany). 4-amino-1,2,4-triazole was obtained from Fluka. All other chemicals were of analytical grade and were used without further purification.
2.2. Modification of Eupergit C® and Adsorption of Invertase
Eupergit C® is a spherical acrylic polymer (having particles with diameter of 150 µm) containing epoxide groups as reactive components. These groups function as active components for the covalent binding of ligands containing amino, mercapto, or hydroxyl groups. Eupergit C®-Tri (EC®-Tri) beads were prepared by the conventional method on Eupergit C® supports which involved direct triazole binding on polymers via oxirane groups as shown in Figure 1. Eupergit C® was incubated with an excess amount of triazole in 1.0 M phosphate buffer solution (pH 7.0) at 25˚C. After incubation for 4 days, the beads were collected on a sintered-glass filter, washed with 1.0 M NaCl for three times, and subsequently washed with the same buffer solution. The excess of epoxide groups on the Eupergit C®-Tri beads were bl˚Cked by incubation with glycerol for 4 h at 25˚C.Chelates of Cu2+ ions with EC®-Tri beads were prepared by mixing 0.1 g of beads with 5 ml aqueous solutions containing 50 ppm Cu2+ ions at a constant pH value of 5.0 (adjusted with HCl and NaOH). The flask was shaken at 25℃ for 24 h. The concentration of Cu2+ ions in the resulting solution was determined using a graphite furnace atomic adsorption spectrometer (Analyst 800/Perkin Elmer, USA). The amount of adsorbed Cu2+ ions was calculated by determining the concentrations of the Cu2+ ions in the initial solution and in the equilibrium. Invertase was adsorbed onto the Cu2+ chelated Eupergit C®-Tri beads at various pH values, either in acetate buffer (0.1 M, pH 3.0-5.5) or in phosphate buffer (0.1 M, pH 6.0-9.0). The initial invertase concentration was determined as 1 mg/ml. The adsorption experiments were conducted for 3 h at 25˚C while shaking continuously. At the end of the time period, the enzyme adsorbed beads were removed and washed three times with the same buffer solution. The enzyme adsorbed beads were then stored at 4˚C in fresh buffer solution until use. The amount of adsorbed invertase (Q) was calculated using the following equation:
where, Q is the amount of adsorbed invertase (mg protein/g Eupergit C®-Tri beads); Co and Ce are the initial and equilibrium concentrations in the solution (mg/ml); V is the solution volume (ml); and m is the mass of the Eupergit C®-Tri beads (g).
2.3. Invertase Desorption Studies
The invertase adsorption and desorption cycle was repeated 10 times in order to evaluate the reusability of EC®-Tri-Cu2+ beads. Invertase desorption from EC®-Tri-Cu2+ beads was carried out with 25 mM EDTA. The beads were washed several times with phosphate buffer (0.1 M, pH 7.0), and were subsequently reused in enzyme immobilization.
2.4. Enzyme and Protein Assay
The enzymatic activities of the immobilized and freeinvertasewere determined by Nelson’s method[11]. Various concentrations of sucrose solution were preincubated for 10 min at 25°C, and the enzyme electrode was placed in sucrose solutions for specific reaction times (2, 4, and 6 min). After removing the immobilized enzymes, 1 mL aliquots were drawn and added to 1 mL Nelson’s reagent to terminate the reaction. Subsequently, the tubes were placed in a boiling water bath for 20 min. The tubes were then cooled down and 1 mL of arsenomolybdate reagent was added. As a final step, 7 mL of distilled water was added to each test tube and mixed by vortexing. Once the mixing was complete, the absorbances for the blank and the substrate solutions were determined at 540 nm using a double beam spectrophotometer. One unit of invertase activity was defined as the amount of enzyme required to release 1 μmol glucose from sucrose per minute at a pH value of 5 and a temperature of 25°C.The protein concentrations of polymer networks were determined by Lowry method[9] using bovine serum albumin as the standard. The quantity of entrapped protein was calculated by subtracting the amount of protein recovered in the combined washings of the invertase-EC from the amount of protein used for immobilization.
3. Properties of Free and Immobilized Enzymes
3.1. Optimum PH
The effect of pH on the activity and the stability (incubated for two hours in buffer solution) of the free and the immobilized invertase was assayed in acetate buffer (0.1 M) in the pH range of 3.0-6.5 and in phosphate buffer (0.1 M) in the pH range of 7.0-9.0 using the standard activity assay pr˚Cedure mentioned above.
3.2. Optimum Temperature
The effect of temperature and thermal stability on the activity of both free invertase and bound invertase was studied in the temperature range of 20˚C to 80˚C and assayed under the standard assay conditions. The thermal stability of invertase was evaluated by measuring the residual activity of invertase exposed to various temperatures ranging between 20˚C and 80˚C in phosphate buffer (0.1 M, pH 7.5) for 15 minutes. After heating, the samples were quickly cooled to 25˚C and assayed immediately for determining the enzymatic activity as done earlier.
3.3. Storage Stability and Reusability
The immobilized invertase was stored in phosphate buffer (0.1 M, pH 7.5) at 4 C and 25˚C for 35 days and its activity was measured at frequent intervals. The immobilized invertase was repeatedly used in order to test its reusability under the standard assay conditions. After each activity assay, the samples were washed with the buffer and then stored until the next assay in the buffer solution.
4. Results and Discussion
4.1. Adsorption of Invertase
The adsorption of invertase for Cu2+-chelated triazole functionalized Eupergit® C beads is presented in Figure 2. The adsorption could ˚Ccur for specific interactions between invertase and the Cu2+ chelated EC-Tri beads. It can be observed from the graph that the adsorption efficiency is related to the concentration of the invertase in the adsorption. However, this was found to level off at an invertase concentration of 0, 8 mg/ml and the maximum adsorption for beads was obtained as 72, 8 mg/g. In addition, it can be observed that the adsorption could not be achieved for EC-Tri beads without Cu2+ chelation. These results indicate that copper chelation is important for the adsorption of enzyme[12]
4.2. Kinetic Parameters
The kinetic parameters, Michaelis constants Km and Vmax for free and immobilized invertase were determined using the Lineweaver–Burk plot. For the free enzyme, Km was found to be 65.7 mM whereas Vmax was calculated as 153.2 µM min-1 mg-1enzyme. The Km value was found to be 27.6 mM for EC-Tri–Cu2+-invertase. The Vmax value of immobilized invertase was estimated from the data as 45.2 µM min-1 mg-1enzyme. As expected, the Km and Vmax values were significantly affected after adsorption onto the Cu2+ incorporated EC-Tri beads.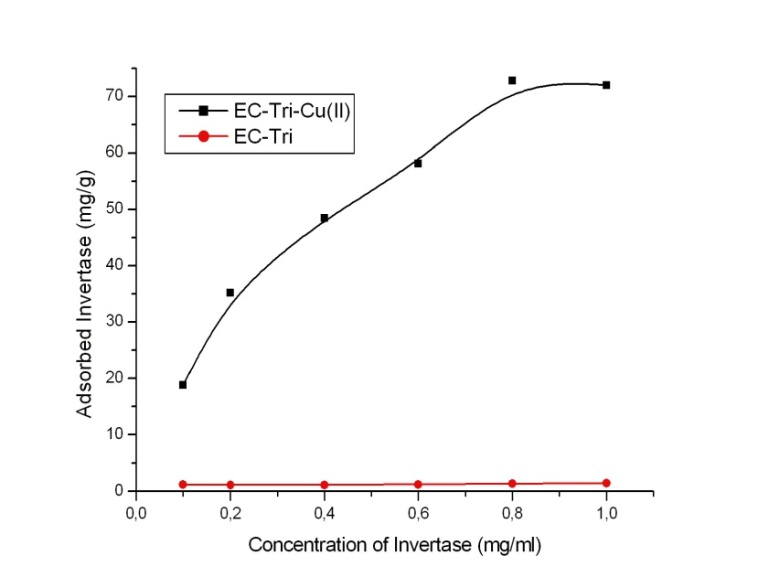 | Figure 2. Effect of invertase concentration on the adsorption efficiency of EC-Tri –Cu2+ |
4.3. Optimum Temperature
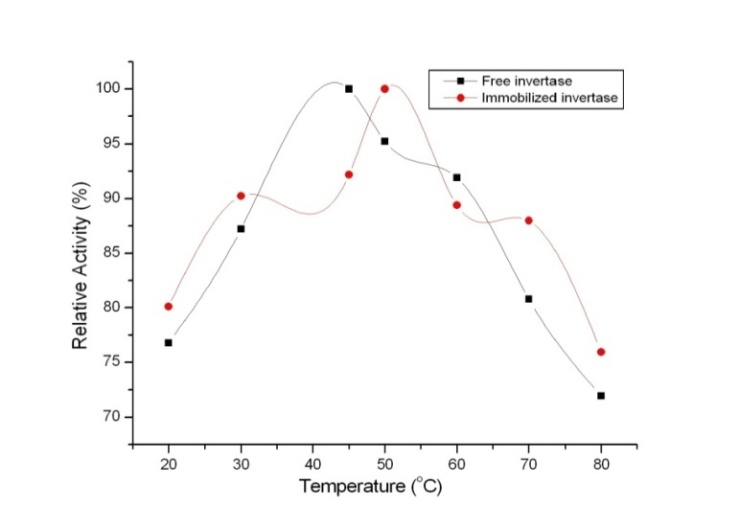 | Figure 3. Optimum temperature of the free and immobilized invertase |
The maximum activity for the free and adsorbed enzyme preparations was observed in the temperature range of 45-50 ˚C (Figure 3). The activities obtained in the temperature range of 20–80˚C were expressed as a percentage of the maximum activity. The temperature profiles of the free and adsorbed invertase were investigated at the invertase concentration of 0,8-mg/ml and pH value of 5.0. It was found that for free enzyme, the relative activity increased with increasing temperature in the range of 20–45˚C and exhibited a maximum at 45˚C. In this temperature range, the thermal deactivation was probably slow and had no appreciable effect on the rate of the catalyzed reaction. Subsequently, an increase was observed in the relative activity with the increasing temperature. It was found that the activity of free enzyme decreased at temperatures higher than 45˚C, probably owing to thermal deactivation. However, the activity of adsorbed invertase increased continuously with increasing temperature in the range of 20–50˚C. Thus, it is clear that the adsorption of the enzyme by method of chelation contributed to the increase of the optimum temperature of the enzyme. This result is similar to literature[3]. On the other hand, it resulted in an increase in the activation energy of the enzyme to reorganize an optimum conformation for binding to its substrate. Thus, it can be concluded that the adsorption caused a significant improvement in the thermal stability of invertase.
4.4. Optimum PH
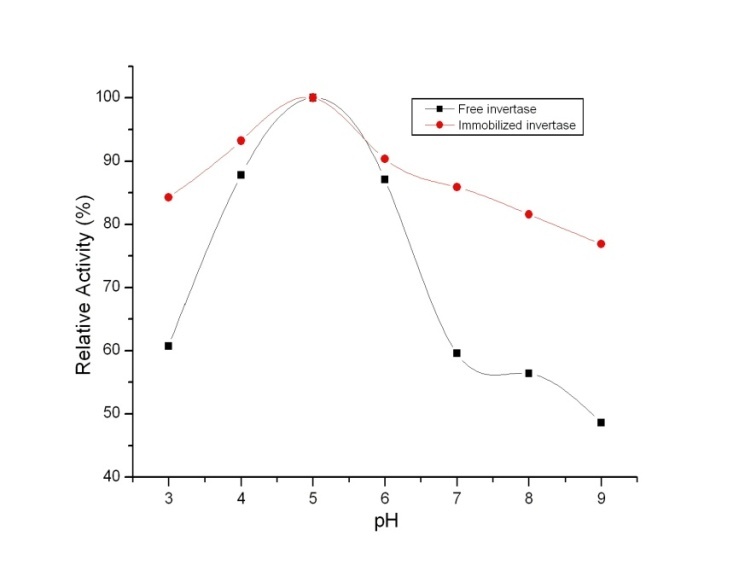 | Figure 4. pH profiles of free and immobilized invertase |
Enzymes are very delicate molecules; they can be easily denaturized by removing them from their natural medium. Therefore, the pH value of the immobilization is important. The effect of pH on the free and the immobilized preparations was investigated in the pH range of 3.0 to 9.0 in acetate and phosphate buffers, and the results are presented in Figure 4. It was found that the immobilized invertase preparation has definitely the same optimum pH as the free enzyme (pH: 5.0). The maintenance of optimum pH for the free and immobilized invertase can probably be attributed to the fact that the concentration of the charged species in the domain of the immobilized enzyme is similar to that in the bulk solution[2]. However, activities of the immobilized preparations were higher than those of the free enzyme in the pH range of 3.0 to 9.0. This can be explained by the multi chelate interactions that protect the immobilized enzyme against quick denaturization as compared to the free enzyme.
4.5. Storage Stability
Another significant feature of immobilized enzymes is their storage stability which is an advantage when compared with free enzymes which tend to lose their activities considerably quickly (Figure 5). In general, if an enzyme is in aqueous solution, it is not stable during storage, and the activity is gradually decreased. The free invertase and the immobilized invertase were stored in pH 5.0 acetate buffer at 4˚C and 25˚C. The activities were then measured over a period of 35 days (Figure 6). It was found that for 25˚C, the free enzyme lost its 25% activity, and the immobilized enzyme lost about 22% of its activity. On the other hand, for 4 ˚C, the free enzyme lost about 19% of its activity whereas the immobilized enzyme lost about 10% of its activity during this period. This decrease in enzyme activity was explained as a time-dependent natural loss in enzyme activity; however, this could be prevented to a significant degree upon adsorption. The result clearly indicates that the immobilized invertase exhibits an improved stability over that of the free enzyme. 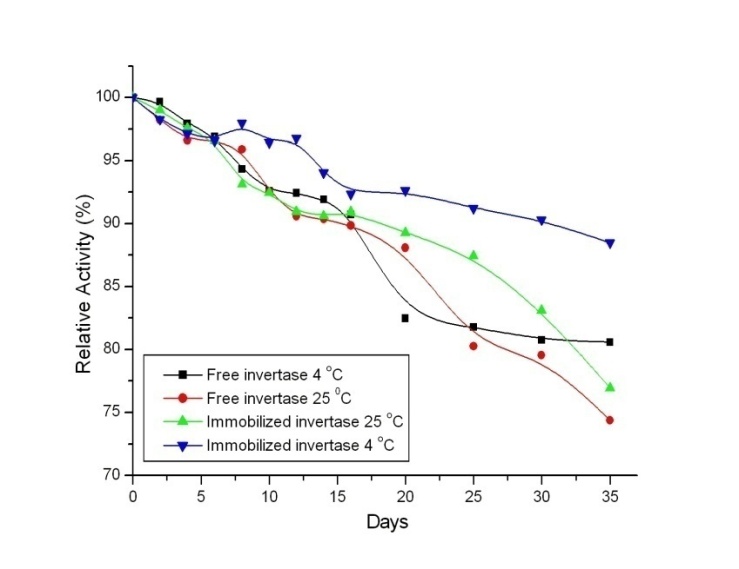 | Figure 5. Storage Stability of the free and immobilized invertase at 4 and 25˚C |
4.6. Effect of PH on the Adsorption
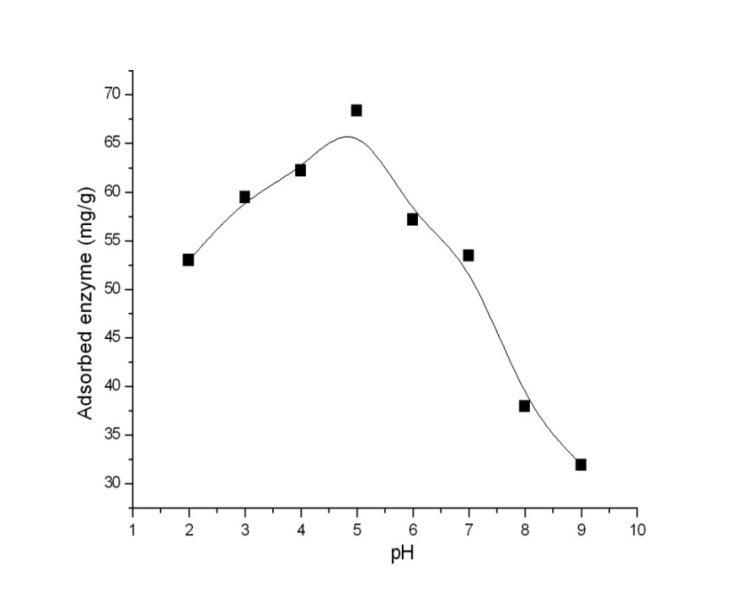 | Figure 6. Effect of pH on invertase adsorption onto of Cu2+ chelated functionalized Eupergit® C beads: invertase concentration, 0,8 mg/ml; time, 3 h; T, 20˚C |
Proteins have no net charge at their isoelectric points, and therefore the maximum adsorption from aqueous solutions is usually observed at their isoelectric points. The isoelectric pH of invertase is 4.6. The influence of pH on the immobilization is shown in Figure 6. The highest immobilization efficiency (68,36 mg/g) was obtained at pH 5.0, which is the optimum pH for both the free and the immobilized enzymes. Lower immobilization efficiencies were obtained at both lower and higher pH values. It can be clearly seen that it had slightly shifted toward more neutral pH values. This was probably owing to the preferential interactions between invertase molecules and Cu2+ incorporated polymeric matrix at neutral pH values.
4.7. Adsorption and Desorption Cycle
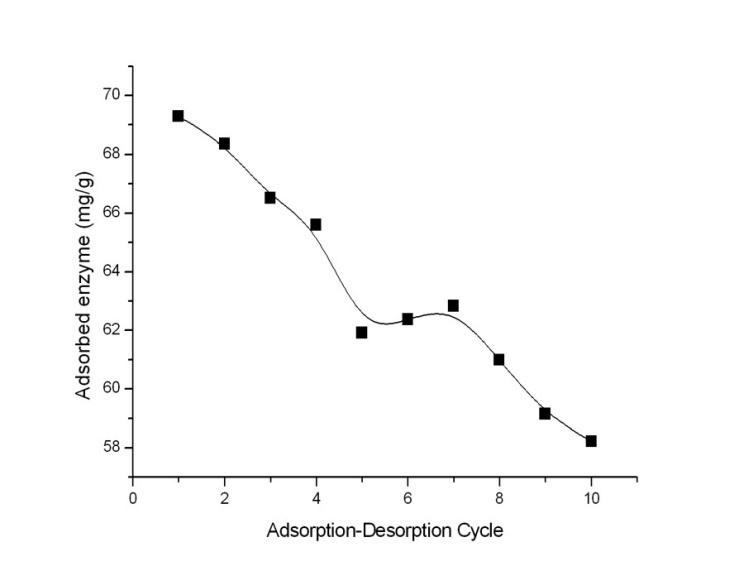 | Figure 7. Repeated use of immobilized invertase; invertase concentration 0,8 mg/ml; pH 5,0; time, 3 h; T, 20˚C |
The most important advantage of immobilization is the ability for repeated use of the enzyme. Desorptions of invertase from Cu2+chelated functionalized Eupergit® C beads were applied in a system containing 25 mM EDTA at room temperature for 2h. Immediately after this, the beads were repeatedly used in the adsorption of invertase. It was found that for as long as 10 successive adsorption–desorption cycles (Figure 7), the beads eminently maintained the adsorption capacity. Besides, the enzyme activities of the preparations did not significantly change during this adsorption–desorption cycles. After the 10 reactions, it was found that the adsorbed invertase conserved an activity of 82%, thereby demonstrating the usefulness of the enzyme-loaded beads in bi˚Catalytic applications. These results indicated that the beads could be repeatedly used in enzyme immobilization, without detectable losses in the initial adsorption capacities and activities.
5. Conclusions
In this study, Eupergit® C beads were functionalized with aminotriazole groups for the reversible immobilization of enzymes. Metal-chelating ligand release is a common problem that is encountered in any immobilized metal-chelate affinity adsorption technique, which causes a decrease in the adsorption capacity. It is well known that metal-chelating ligand leakage from the adsorbent causes contaminations that will interfere with the analysis of the purified protein. Therefore, the metal- chelating ligand immobilization step was eliminated in this approach. The Michaelis-Menten kinetic constants Km and Vmax of the free and immobilized invertase were determined which revealed that the affinity of invertase to sucrose decreased after immobilization. The properties of the free and the immobilized invertase were compared and the results indicated that the stability of the immobilized invertase towards temperature, pH reusability, and storage was enhanced by immobilization. To conclude, the aminotriazole functionalized EC beads revealed good properties as adsorptive beads and will therefore prove to be useful for applications in biotechnology.
References
[1] | Avnir, D., S. Braun, O. Lev and M. Ottolenghi, 1994. Enzymes and Other Proteins Entrapped in Sol-Gel Materials. Chem. Mater., 6: 1605-1614. |
[2] | Chen, Y., E.T. Kang, K.G. Neoh and K.L. Tan, 2000. Covalent immobilization of invertase onto the surface-modified polyaniline from graft copolymerization with acrylic acid, European Polymer Journal, 36: 2095-2103. |
[3] | Danisman, T., S. Tan, Y. Kacar and A. Ergene, 2004. Covalent immobilization of invertase on microporous pHEMA–GMA membrane. Food Chem., 85: 461-466. |
[4] | Dhawan, G., G. Sumana and B.D. Malhotra, 2009. Recent developments in urea biosensors. Bi˚Chem. Eng. J., 44: 42-52. |
[5] | Giorno, L., N. Li and E. Drioli, 2003. Use of stable emulsion to improve stability, activity, and enantioselectivity of lipase immobilized in a membrane reactor. Biotechnol. Bioeng., 84: 677-685. |
[6] | Kara, A., B. Osman, H. Yavuz, N. Beşirli and A. Denizli, 2005. Immobilization of α-amylase on Cu2+ chelated poly(ethylene glycol dimethacrylate-n-vinyl imidazole) matrix via adsorption. Reac. Funct. Polym, 62: 61-68. |
[7] | Katchalski, E.K., 1993. Immobilized enzymes—learning from past successes and failures. Trends Biotechnol., 11: 471-478. |
[8] | Kuswandi, B., R. Andres and R. Narayanaswamy, 2001. Optical fibre biosensors based on immobilised enzymes. Analyst, 126: 1469. |
[9] | Lowry, O., N. J. Rosebrough, AL. Farr and R.J. Randall, 1995. Protein measurement with the folin phenol reagent. J. Biol. Chem., 193: 265-275. |
[10] | Melo, J.S. and S.F. D’Souza, 2000. A simple approach for the simultaneous isolation and immobilization of invertase using crude extracts of yeast and Jack bean meal. J. Bi˚Chem. Biophys. Methods, 42: 133-135. |
[11] | Nelson, N., 1944. A photometric adaptation of the Somogyi method for the determination of glucose. J. Biol. Chem., 153: 375-380. |
[12] | Porath, J., 1990. Amino acid side chain interaction with chelate-liganded crosslinked dextran, agarose and TSK gel. A mini review of recent work J. Mol. Recogn. 2: 123-127 |
[13] | Rosen-Margalit, I. and J. Rishpon, 1993. Novel approaches for the use of mediators in enzyme electrodes. Biosens. Bioelec., 8: 315-323. |
[14] | Torres R., Mateo C., Fuentes M, Palomo J.M., Ortiz C., Fernandez-Lafuente R. and Guisan J.M., 2002. Reversible immobilization of invertase on Sepabeads-polyethylenimine: stabilization of a multimeric enzyme. Biotechnol. Prog., 18: 1221-1226. |
[15] | Tanioka, A., Y. Yokoyama and K. Miyasaka, 1998. Preparation and properties of enzyme-immobilized porous polypropylene films. J. Colloidal Interf. Sci., 200: 185-187. |