S. J. Gemanam1, J. K. Aondoakaa1, T. Sombo2
1Department of Physics, Benue State University, Makurdi, Nigeria
2Department of Physics, Federal University of Agriculture, Makurdi, Nigeria
Correspondence to: T. Sombo, Department of Physics, Federal University of Agriculture, Makurdi, Nigeria.
Email: |  |
Copyright © 2017 Scientific & Academic Publishing. All Rights Reserved.
This work is licensed under the Creative Commons Attribution International License (CC BY).
http://creativecommons.org/licenses/by/4.0/

Abstract
Evaluation of Protective Shielding Thickness of Benue State University Teaching Hospital Makurdi Diagnostic Radiology Room was carried out using the Radarlat100 radiation meter. The workload of 125 (mA-min/wk.) and use factor 0.56 of the diagnostic X-ray machine was used to determine the shielding parameters at an operating potential of 150 kVp. The primary and secondary protective barriers of 3.95mm each were calculated. The wall thickness around the diagnostic room was measured to be 3.0 x 102 mm. This shows that the protective shielding thickness is adequate compared to the international recommended standard value of 74.0 ± 7.4 10-1 mm even for the highest peak voltage.
Keywords:
Protective Shielding Thickness, Diagnostic Radiology Room
Cite this paper: S. J. Gemanam, J. K. Aondoakaa, T. Sombo, Evaluation of Protective Shielding Thickness of Benue State University Teaching Hospital Makurdi, Diagnostic Radiology Room, Nigeria, International Journal of Biophysics , Vol. 7 No. 1, 2017, pp. 1-4. doi: 10.5923/j.biophysics.20170701.01.
1. Introduction
Radiation shielding is the science and practice of protecting people and the environment from the harmful effects of ionizing radiation. In order words it is a term applied to concepts, requirements, technologies and operations related to protection of people (radiation workers, members of the public, and patients undergoing radiation diagnosis and therapy) against the harmful effects of ionising radiation. It has its origins early in the twentieth century [1]. Over the years, X-rays have become an important tool in medical diagnosis and therapy. Therefore, the overt balancing of benefits from nuclear and radiation practices against radiation risk, and efforts to reduce the residual risk, has become a major feature of radiation protection [2].There are many types of radiations which may be injurious to health; the primary ones of concern being X-rays, gamma rays and neutron particles. It is widely accepted that if adequate shielding is provided for these forms of radiation, then the effects from the others can be considered negligible [3]. Theoretically, all materials could be used to attenuate the radiation to safe limits, however, due to certain characteristics, lead, copper and concrete are among the most commonly used materials [4]. In spite of numerous works on the determination of shielding parameters by researchers such as Ogbaje (2000), Okunade and Awodele (2001), Singh et al., (2010), Agba et al.; (2011), Ismail R. H (2014) and Shahid et al., (2014) etc. the National Council on Radiation Protection and Measurements (NCRP) provides the widely accepted methodology for radiation shielding design, which has been reviewed and the new recommendations are contained in NCRP, (2005) [5]. This work is aimed at complimenting the works of other researchers on determination of shielding parameters of diagnostic X-ray rooms of hospitals situated in Benue State with a view to determining the adequacy or otherwise of primary and secondary protective shielding of X-ray rooms based on NCRP, (2005) and NCRP, (2015) [5] [6] recommendations.
2. Materials and Method
The radiation meter (Radalart 100) and measuring tape 5m (16Ft) long where use for measurement of radiation at BSUTH to determine the exposure of X-ray machine at 1m from the source. The X-ray machine used in this work is a 3 – phase static conventional X-ray machine situated at the X-ray department of BSUTH. Details of the research method are reported elsewhere [7]. The following exposures and shielding parameters; primary exposure Xp, Incident exposure
Scattered Xs, Tube output K, Workload W, Occupancy factor, Exposure towards primary/secondary barrier of concrete thickness were calculated using the following equations:The following formulas were used to estimate the exposure and shielding parameters: | (1.1) |
Where W is the workload in (mA-min/wk)  | (1.2) |
Where X is the exposure rate in mR (mili Roentgent)The exposure per week contributed by the primary exposure (Xp), scatter exposure (Xs) and the leakage exposure (Xl) will be computed using the following equations (Bushberg et al., 2002) [8]. | (1.3) |
Where Xp is the primary exposure, W is the workload and K is the tube outputThe incident exposure at 1m
is corrected for distance to the scattered, dsec as; | (1.4) |
Where Xp is the primary exposure,
is the scattered distance and
is the incident exposure. The scatter exposure per week, Xs, at a distance of 1m from the scattering source (i.e. the surface of the patient) is calculated as; | (1.5) |
S is the scattered fraction which is 0.15%,
is the incidence exposure and Xs is the scattered exposure in mR/week.  | (1.6) |
Where 1.67 mR/ (mA max-min) is the maximal leakage radiation, and W is the workload in (mA-min/week). The division of Xp, Xs and XL by dpri, dsec and dleak respectively will yield the exposure levels at 0.3m beyond the wall in the adjacent room.The total weekly exposure X, without shielding at a point 0.3m beyond the wall in an area adjacent to an X-ray room is calculated using the following equation given by Bushberg et al., (2002)  | (1.7) |
Where
is the primary exposure,
is the primary distance,
is the primary use factor,
is the scattered exposure while
is the secondary distance.
is the leakage exposure while
is the leakage distance.
3. Results
The experimental results were obtained after detailed computations were carried out from the measured radiographic parameters/shielding distances at the Benue state university teaching hospital makurdi. These results are presented in tables 1 to 4 below.Table 1. Measured Radiographic Parameters obtained at BSUTH 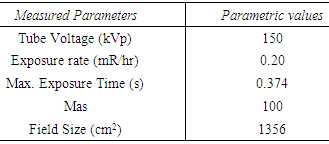 |
| |
|
Table 2. Measured Shielding Distances obtained At BSUTH 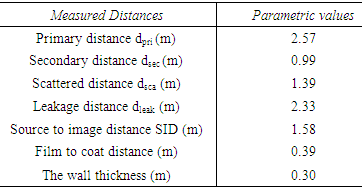 |
| |
|
Table 3. Computed Exposure Levels obtained at BSUTH 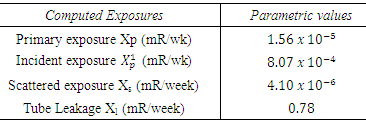 |
| |
|
Table 4. Shielding Parameters obtained at BSUTH 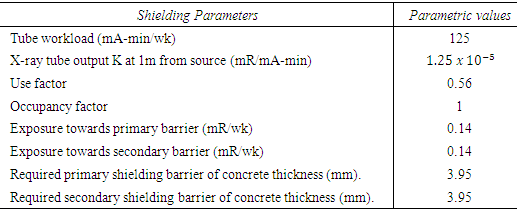 |
| |
|
4. Discussion
The measured radiographic parameters and shielding distances obtained after detailed computations shows that the diagnostic unit of Benue State University Teaching Hospital has a workload of 125mA-min/week. This value falls within the range of 100mA-min/week – 1000mA-min/week for a less busy radiographic room and a very busy radiographic room respectively as recommended by the National council on Radiation Protection and Measurements NCRP, (2005). The present work also lies between the workload range of 73mA-min/week to 530mA-min/week for Orthopaedic facilities and 500mA-min/week for one in absence of precise data for shielding design purposes, recommended by Bushong S.C. and Glaze A. (1983) [9].Table 5 below compares the workload obtained at Benue State University Teaching Hospital with results of Agba et al., (2011) carried out at Federal Medical Centre Makurdi and compares with those of E. O Esien-Umo (2007) [10] carried out at Ahmadu Bello University Teaching Hospital Zaria and the work of Okunade, A.A and Awodele, M.K, (2001) [11] carried out at Ibadan and the recommendation of NRCP.49.Table 5. Compared Results of workload and use factor from different researchers  |
| |
|
It is observed from the above table that the results of workload obtained at Benue State University Teaching Hospital are higher than those obtained by Agba et al., (2011) at Federal Medical Centre Makurdi and Okunade at Ibadan due to the frequent examinations carried out at the hospital, but less than those of E. O Esien-Umo (2007) and the NCRP recommendations. This work lies between those of Agba et al., (2011) and NCRP 49 findings. Hence the research work is consistent and in good agreement with the published works compared above.For the primary shielding calculations, the exposure per week (X) without shielding at a position 0.3m beyond the primary/secondary protective barriers obtained at Benue State University Teaching Hospital are 0.14mR/week for both primary and secondary barriers respectively. The results are less than the recommended exposure limit (Xlimit) of 2 mR/week Bushberg et al., (2002).From the alternative formula by Zuk, W.M. (2002) [12] for estimating attenuation in concrete of X-ray generated at 50 to 300 kVp, the primary barrier thickness required to shield the radiology room of Benue State University Teaching Hospital Makurdi was estimated to be 3.95 mm for both primary and secondary. This is shown in fig 1.0 below.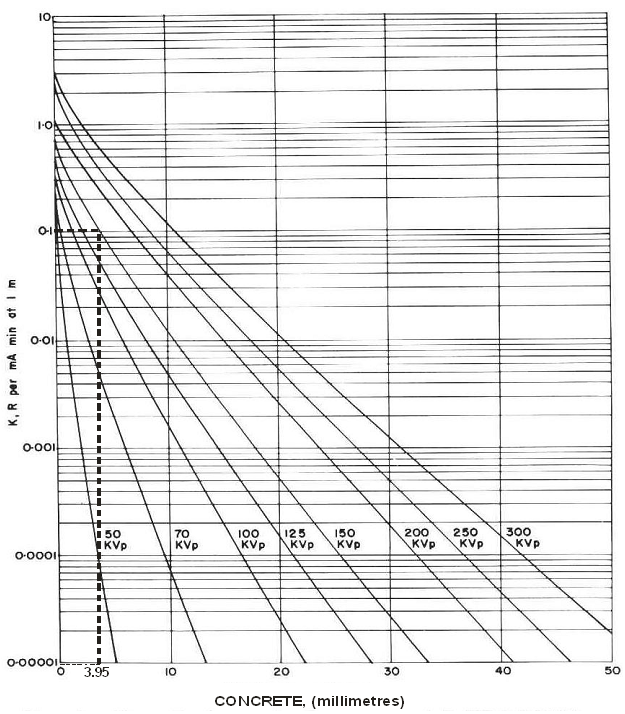 | Figure 1. Estimated attenuation in concrete of X-ray generated at 150kVp |
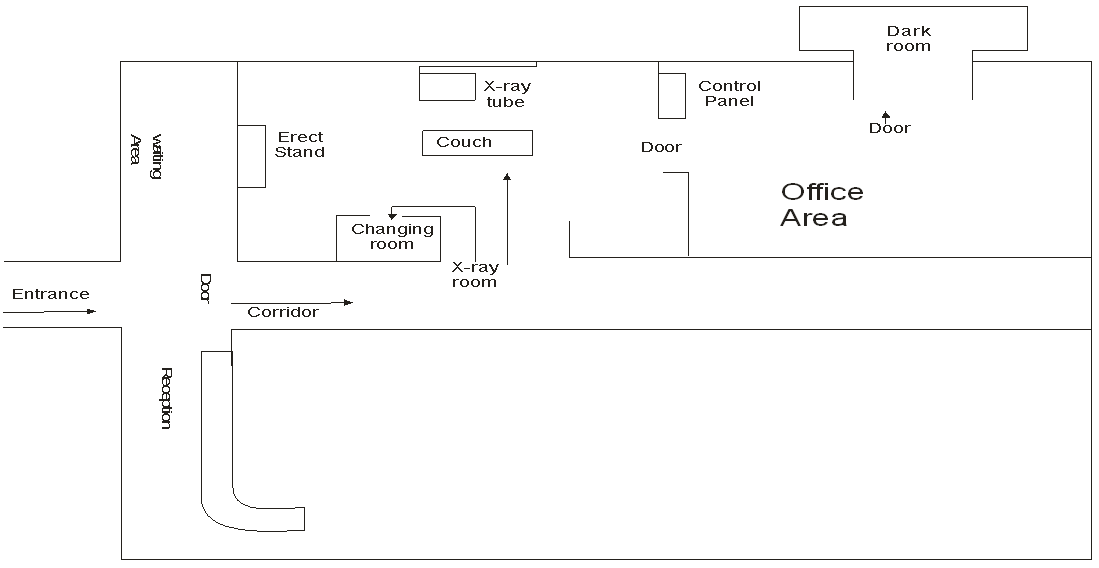 | Figure 2. Diagrammatic overview of X-ray room in BSUTH |
The wall thickness at the BSUTH radiology room was measured to be 300 mm. The value of exposure towards the primary and secondary barrier is very small compared to the wall thickness; thus the room is adequately shielded.
5. Conclusions
The protective shielding parameters evaluated at Benue State University Teaching Hospital Makurdi are in conformity with the recommended maximum limits of the National Council for Radiation Protection and Measurements (NCRP 49 and 151) protocols. This implies that the walls of the diagnostic room of the hospital investigated have adequate protective shielding and does not require any additional structural shielding barriers.
ACKNOWLEDGEMENTS
The authors are grateful to the management of Benue State University Teaching Hospital, (BSUTH) Makurdi for giving them Ethical clearance and cooperation to use the X-ray unit of Radiology department for this research.
References
[1] | Nuclear Energy Agency. An analysis of principal nuclear issues (1994). https://www.oecd-nea.org/brief/brief-10.html. |
[2] | Martin, J. E. (2006). ”Physics for Radiation Protection: A Handbook”. Second Edition. WILEY- VCH Verlag GmbH & Co. KGaA, Weinheim. |
[3] | Mackenzie, D (2010). A guide to the use of Lead for Radiation Shielding. Vol1, pp 3-6. |
[4] | Karson, Z (2010). A guide to the use of Lead for Radiation Shielding. Vol. 1, pp 3-6. |
[5] | NCRP, (2005). Structural Shielding Design and Evaluation of Medical use of X-ray and Gamma rays of energies up to 10Mev. National Council on Radiation Protection Report 49, Bethesda, Maryland, pp. 31-56. |
[6] | NCRP, (2015). Structural Shielding Design and Evaluation for Megavoltage x-and Gamma-ray Radiotherapy Facilities. Report prepared through a joint effort of NCRP Scientific Committee 46-13 on Design of Facilities for Medical Radiation Therapy and the American Association of Physicists in Medicine. Modified 8-6-2015. |
[7] | Agba E.A, Gemanam S. and Sombo T. (2011). Protective shielding parameters for diagnostic X-ray rooms in some selected hospitals in Makurrdi and Gboko towns of Benue State, Nigerria: Nigerian Journal of Physics Vol.22. |
[8] | Bushberg, J.T.; Seibert, J. A; Leidholdt, E.M and Boone, J. M (2002). The essential physics of medical imaging. Lippincott Williams & Wilkins. ISBN 978-0-683-30118-2. |
[9] | Bushong S.C. and Glaze A. (1983) “Radiographic workload and usefactors for Orthopaedic Facilities. Health Physics, pp44, 533-59. |
[10] | E. O Esien-Umo (2007). X-ray shielding barrier estimation: A case study of radiology department, Ahmadu Bello University teaching hospital, shika-zaria. Jourrnal of Radiation Safe. Vol5. Pp. 3-4. |
[11] | Okunade, A. A and Awodele, M.K, (2001): “Determination of Workload and Use factors for Diagnostic Medical X-ray Rooms”. Nig. Journal of Physics vol.13. |
[12] | Zuk, W. M. 2002: “X-ray Equipment in Medical Diagnosis Part A” www.google.com, Ontario 2005. |