T. Sombo1, T. A. Ige2, E. H. Agba3
1Department of Physics, Federal University of Agriculture, Makurdi, Nigeria
2Department of Medical Physics, National Hospital, Abuja, Nigeria
3Department of Physics, Benue State University, Makurdi, Nigeria
Correspondence to: T. Sombo, Department of Physics, Federal University of Agriculture, Makurdi, Nigeria.
Email: |  |
Copyright © 2015 Scientific & Academic Publishing. All Rights Reserved.
Abstract
The mathematical models describing the effect of gamma irradiation dose on the dielectric dispersion properties (Dielectric Spread Parameter (α), dielectric decrement (Δ), and dielectric relaxation time (τ ) of Bovine liver tissue at low and radiofrequency has been developed via dielectric measurements obtained using Gamma Irradiator (GS1000), impedance meter (Booton 7200) and signal generators (Lodstar SG416013 and Harris G85793). The coefficient of fit between the irradiation dose and the dielectric spread parameter (α); dielectric decrement (Δ); and dielectric relaxation time (τ) were found to be 94.0%, 74.6% and 96.0% at low frequency and; 97.8% , 99.8% and 98.2% at radiofrequency. This demonstrates that changes in the irradiated Bovine liver tissues is ‘dose dependent’. The models can be used in evaluating the structural changes in Bovine liver tissue exposed to gamma irradiation dose regime usually encountered in radiology.
Keywords:
Dielectric relaxation time, Dielectric decrement, Dielectric spread parameter, Dielectric permittivity, a.c conductivity
Cite this paper: T. Sombo, T. A. Ige, E. H. Agba, Mathematical Modelling of the Effects of Gamma Irradiation on Dielectric Dispersion of Bovine Liver Tissues at Low and Radiofrequency, International Journal of Biophysics , Vol. 5 No. 1, 2015, pp. 12-17. doi: 10.5923/j.biophysics.20150501.02.
1. Introduction
Mathematical modelling of biological tissues provide reasonable mathematical approximation of the physio-chemical changes occurring in biological systems. One of the basic reasons for modeling biological systems is to increase the level of understanding regarding the cause-effect relationship operative in biological systems (Gerber, 1982 and Whitehead et al., 1981).Dielectric properties of biological tissues have been frequently used to study changes in material composition, cell structure and water content under certain physical conditions (Laogun, 1986; Laogun, et al., 1997). Data on the dielectric properties of mammalian tissues is useful in understanding the basic biophysical interaction mechanisms of electromagnetic fields with mammalian tissues (Stuchly et al., 1982). In-vitro investigations of the effects of electromagnetic radiation on the structural properties of mammalian tissues are often designed to simulate actual effects of radiations on humans especially where in-vivo measurements on the tissues is not feasible. Hence, the need for mathematical models. These studies are prompted by the pride of place given to the use of ionizing radiations in diagnostic and therapeutic radiology (Laogun et al., 2005).The use of gamma radiation in radiology has greatly advanced the practice of medicine. However, recent researches revealed that gamma-rays are capable of causing serious damage to mammalian tissues (Pethig, 1991; UNCEAR, 1993; AAMP, 1996; Russel and Bradley, 2007; Mallidi et al., 2007). The greatest damage induced by gamma-radiation appears as a consequence of uncontrolled production of free radicals or complex ions in living organisms (Jozanov-Stankov, 2003).Modeling the effects of gamma irradiation on tissues will lead to generation of dielectric data which may form the basis for the development and administration of immune-prophylaxic drugs as a counter measure for acute radiation exposures. The information will also assist radiologists in establishing the basis for determination of threshold dose for induction of radiation illness in mammalian tissues.This work seeks to measure the dielectric dispersion properties of gamma irradiated and non-irradiated mammalian tissues; to establish the relationship between gamma irradiation doses and the corresponding changes induced in the irradiated Bovine liver tissues so as to provide the tool for prediction of effects of gamma-irradiation on Bovine liver tissues; and finally to establish threshold dose for induction of morphological changes in gamma irradiated Bovine liver tissues at low and radiofrequencies.
2. Methodology
The liver tissue samples were excised from an adult white Fulani cow which was duely certified healthy by the veterinary doctor attached to Gwagwalada central abattoir in Gwagwalada area council, Abuja, Nigeria. The excised tissue samples were thoroughly washed with double distilled water and preserved in laboratory oven maintained at a temperature of 37±0.50C for six hours so as to remove water from its surface. The eight liver tissue samples were irradiated using the following gamma irradiation doses 1.0Gy, 4.0Gy, 11.0Gy, 20.0Gy, 43.0Gy, 60.0Gy and 85.0Gy respectively.The dielectric sample cells used in this research were constructed and calibrated in line with the method of Laogun (2005) and Agba et al. (2008). The gamma irradiator (GS1000) located at the Gamma Irradiation Facility (GIF) unit of National Nuclear Technology Centre, Abuja was used for irradiation of the Bovine liver tissue samples at the dose rate of 0.36kGy/hr. Dielectric measurements were carried out using Booton 7200 capacitance meter in conjunction with signal generators (Lodstar, SG416013 and Harris, G857993). The effective capacitance DC and Dissipation factor (tan δ) of the gamma irradiated and non-irradiated Bovine liver tissue samples were first measured after which the dielectric permittivity (ε’), the dielectric loss factor (ε’’) and a.c conductivity (σ) were obtained using the equations below: | (1) |
 | (2) |
Where εo = permittivity of free space, k = cell constant, C = effective capacitance, ε’’ = dielectric loss factor, σ = dielectric conductivity, ε’ = dielectric permittivity, and f = frequency of measurement.The dielectric decrement of the tissue samples was obtained from the difference between the dielectric permittivity values measured at limiting frequency and static frequency. The dielectric relaxation time (τ) and the dielectric spread parameter (α) were evaluated using the frequency of the peak values of dielectric loss-factor for each irradiation dose and the Cole-Cole plots of dielectric loss factor (ε’’) versus dielectric permittivity (ε’) respectively. All measurements were carried out at the temperature of 28.0±0.50C in the low and radiofrequency ranges of 0.5kHz-100kHz and 0.5MHz-50MHz respectively. The dielectric structural parameters were then modeled using curve fitting procedure.Histological investigations using optical microscopy provides information about the structural properties of mammalian tissues at cellular level. Dielectric measurements were complemented by histological examination of the gamma irradiated and non-irradiated bovine tissues so as to explore the level of electromagnetic radiation induced tissue damage.The examination procedure involves preparation of microscope slides for the irradiated and non-irradiated Bovine tissue samples. This involves the following steps: Crossing, Dehydration (Ethanol), Clearing (Xylene), Infiltration (Molten Parafin wax), Sectioning, Staining, Mounting and Examination.The micrographs of the Gamma Irradiated and non irradiated Bovine tissue samples were obtained with the aid of Motic Binocular Microscope (1820 Motic SFC-18 series) and a Digital Cannon Camera.
3. Results and Discussion
Table 1. Dielectric parameters obtained from Dielectric dispersion and Cole – Cole plots of ε" against ε' for gamma irradiated and non-irradiated Bovine liver tissue in the frequency range 0.5KHz to 100.0KHz 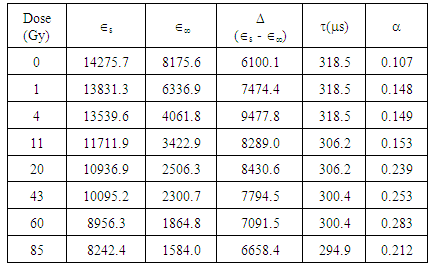 |
| |
|
Table 2. Dielectric parameters obtained from Dielectric dispersion and Cole - Cole plots of ε" against ε' for gamma irradiated and non-irradiated Bovine liver in the frequency range 0.5MHz to 50.0MHz 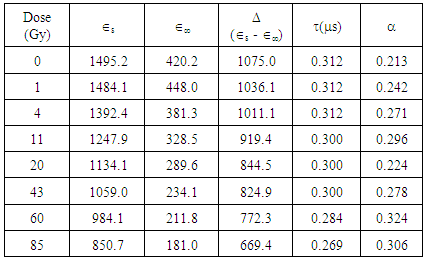 |
| |
|
4. Results and Discussion
The result of the research revealed that the dielectric permittivityε’, of the non-irradiated bovine liver samples is larger than those of the irradiated samples at low and high radiofrequencies. Consequently, reduction in the dielectric decrement was observed with increase in irradiation doses. Since the β-permittivity values of tissue at radiofrequencies depends on the charging and integrity of cell membranes, the observed decrease in permittivity ε’ may be attributed to changes in integrity and structures of the components of the cellular membranes in addition to the reduction in Maxwell-Wagner interfacial polarization effect (Laogun et al., 2005; Agba et al., 2008; Pethig, 1999 and Grant et al., 1978).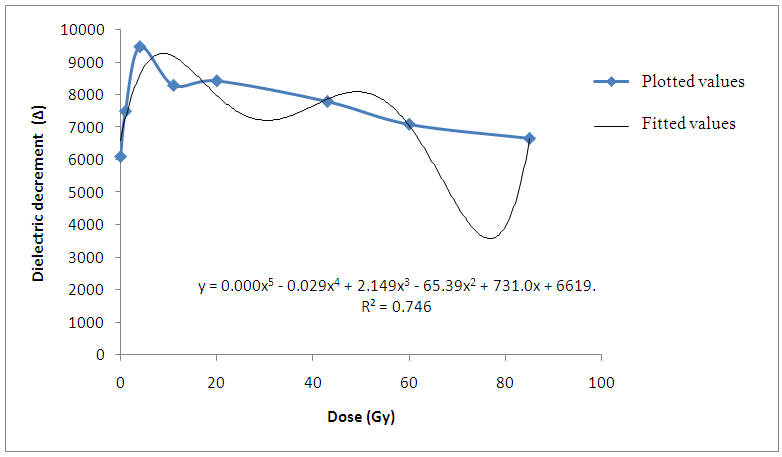 | Figure 1. Variation of Dielectric decrement with gamma irradiation dose for Bovine liver (KHz) |
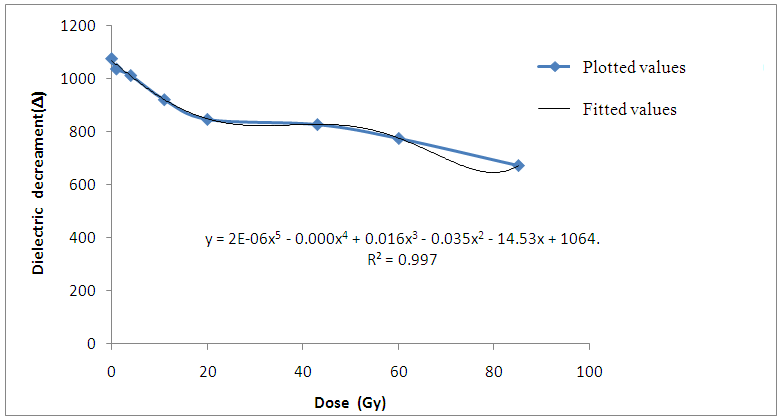 | Figure 2. Variation of Dielectric decrement with gamma irradiation dose for Bovine liver (MHz) |
The increase in dielectric loss ε’’ observed as the gamma-irradiation dosage increases can be attributed to more ionizations produced in the irradiated tissues which would in-turn contribute more polarizable charges or ions thereby increasing the movement of polarizable charges in the applied electric field.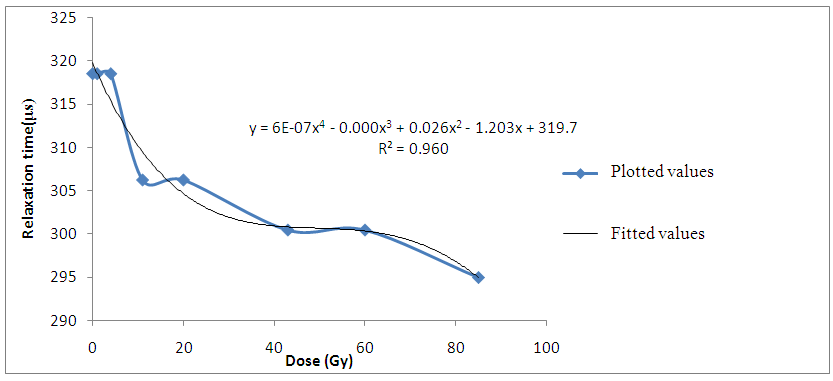 | Figure 3. Variation of Relaxation time with gamma irradiation dose for Bovine liver (kHz) |
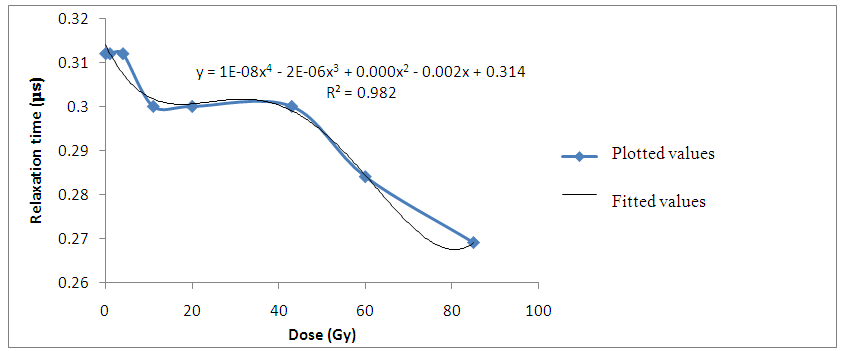 | Figure 4. Variation of Relaxation time with irradiation dose for Bovine liver (MHz) |
The decrease in relaxation time of the liver tissue samples with increasing gamma-irradiation doses also suggest that more ions are produced in the irradiated tissues. Hence, the decrease in time required to charge up the cell membranes of the irradiated liver tissue samples.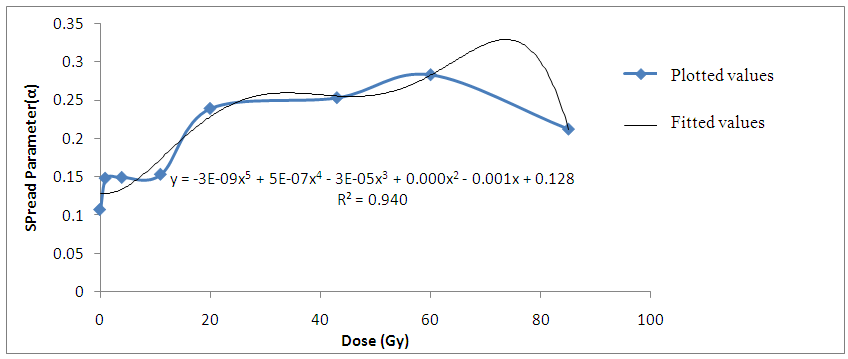 | Figure 5. Variation of Spread Parameter with gamma irradiation dose for Bovine liver (kHz) |
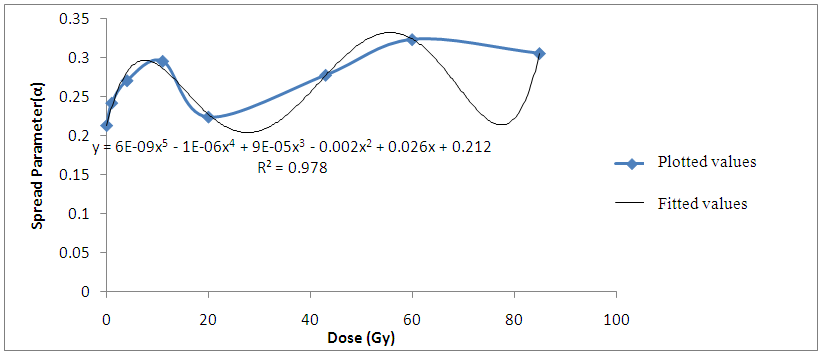 | Figure 6. Variation Spread Parameter with gamma irradiation dose for Bovine liver (MHz) |
Findings also revealed that the gamma-irradiated tissue samples have a larger spread spectrum parameter compared to the non-irradiated Bovine liver samples. The parameter (α) was found to increase as the ionization produced by gamma-irradiation thus increases the heterogeneous distribution of ions in the irradiated liver tissues (Laogun et al., 2005).The average coefficient of fit for spread parameter, relaxation time, and dielectric decrement for the Bovine liver tissues under investigation at low and radio frequency ranges were found to be 94.0% in kHz and 97.8% in MHz; 96.0% in kHz and 98.2% in MHz; and 74.6% in kHz and 99.8% in MHz respectively. This shows that there exist a strong relationship between gamma-irradiation dosage and degree of damage induced in the gamma-irradiated tissues and that the mathematical models can be effectively used to generate dielectric data and to predict the level of damage induced in Bovine liver tissue.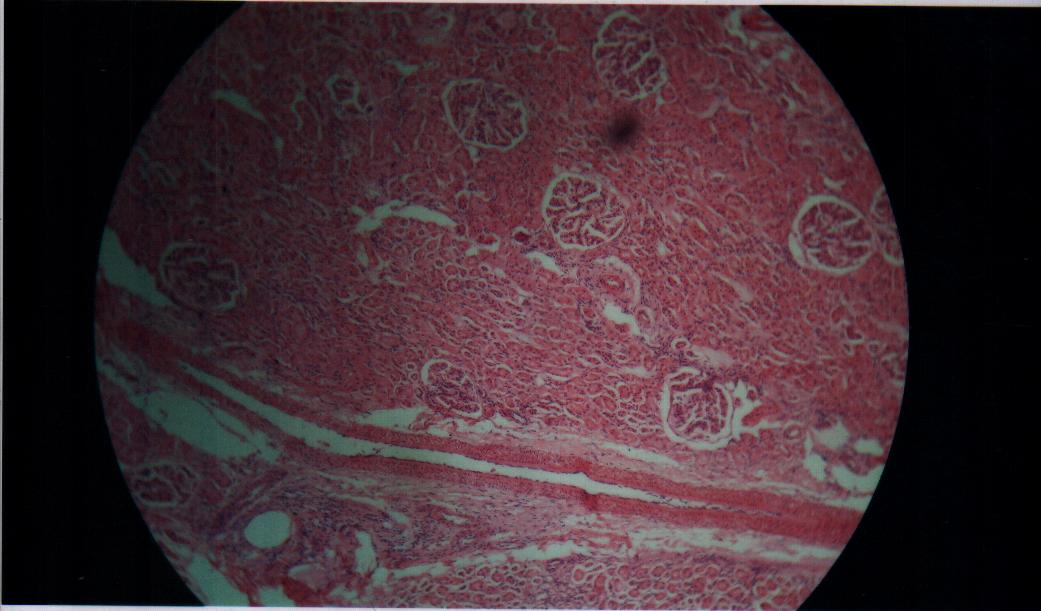 | Figure 7. Non –irradiated Bovine liver tissue |
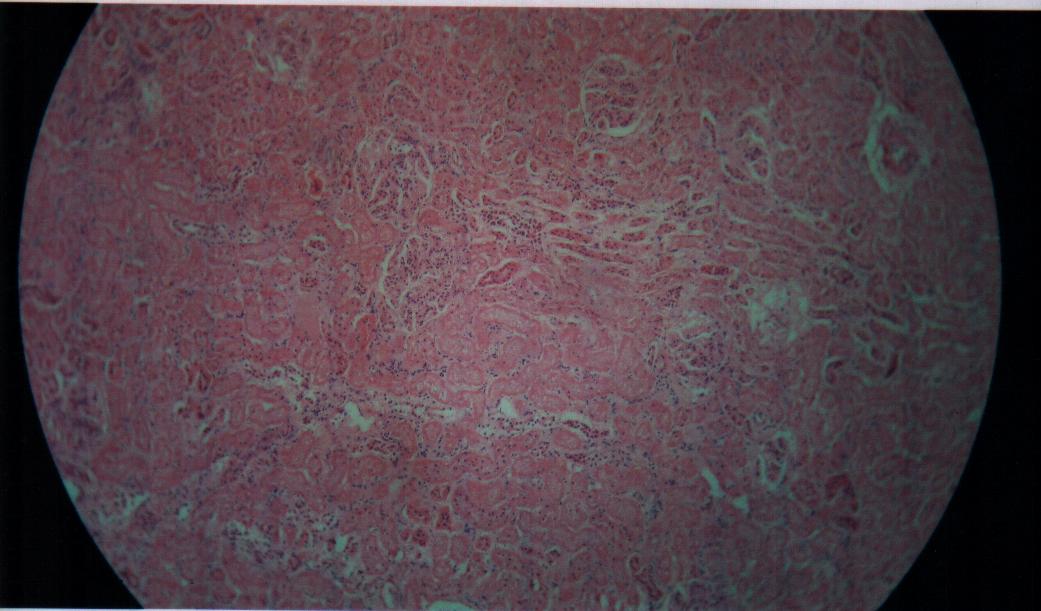 | Figure 8. Bovine liver tissue irradiated with gamma dose of 1Gy |
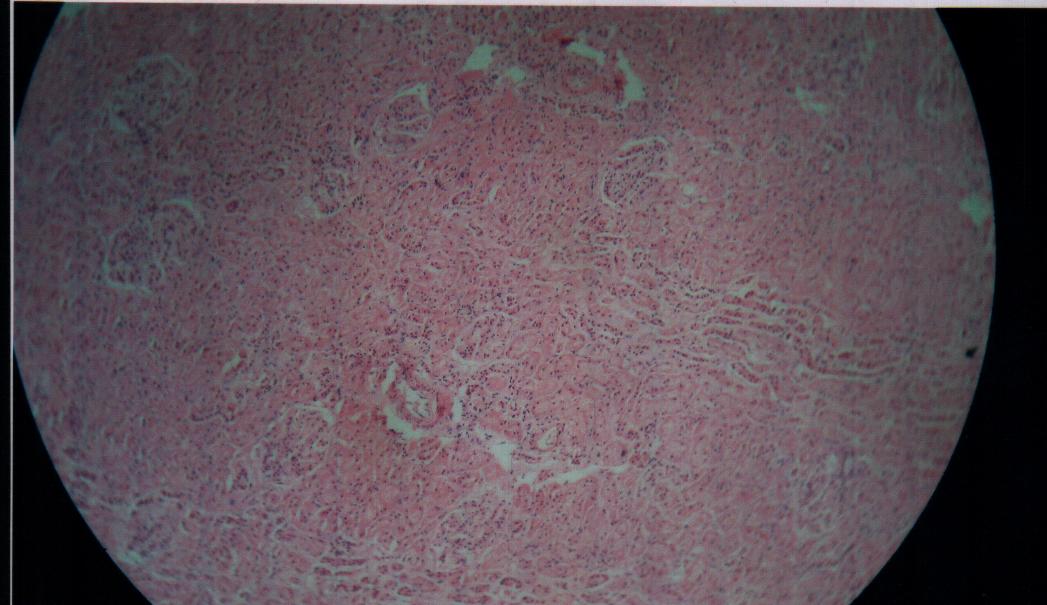 | Figure 9. Bovine liver tissue irradiated with gamma dose of 4Gy |
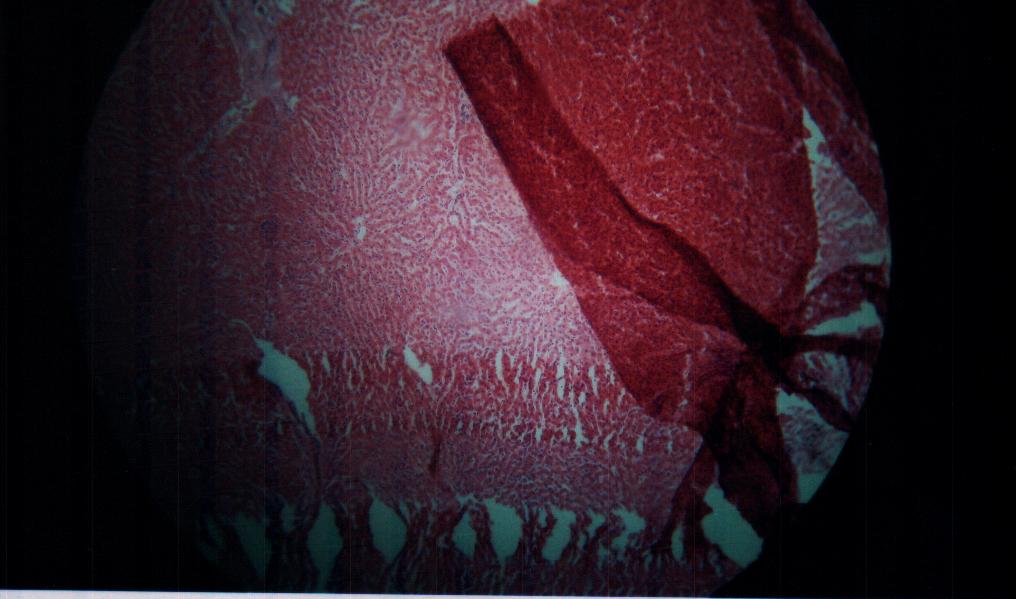 | Figure 10. Bovine liver tissue irradiated with gamma dose of 11Gy |
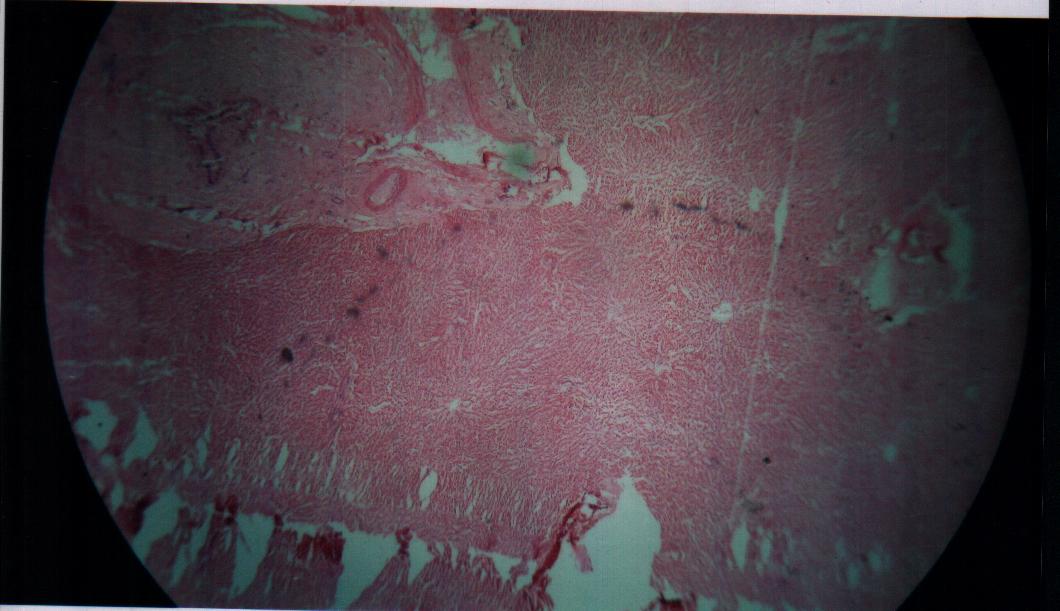 | Figure 11. Bovine liver tissue irradiated with gamma dose of 20Gy |
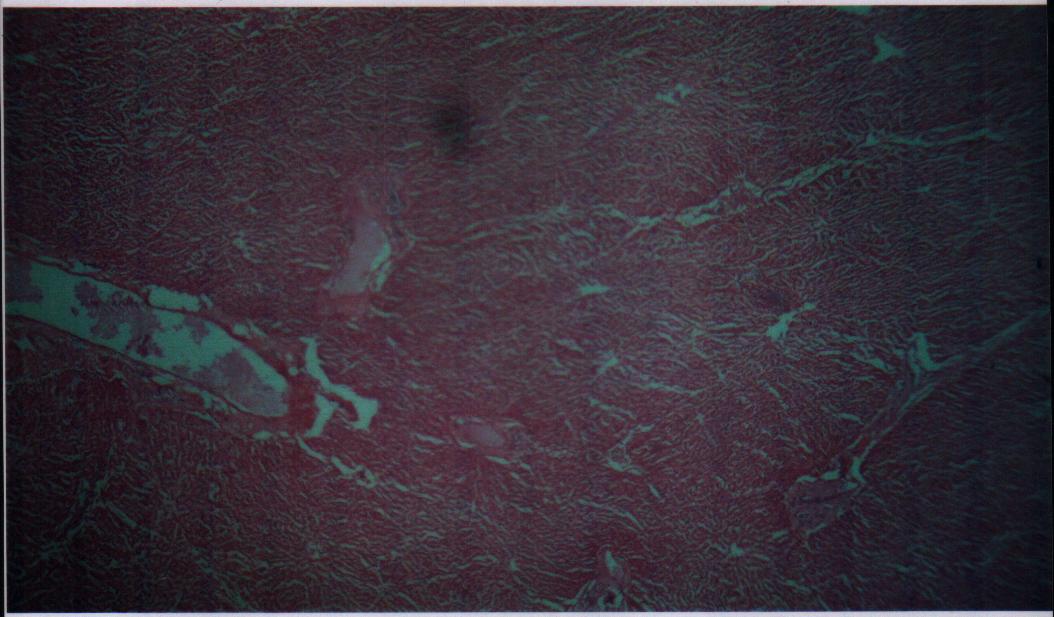 | Figure 12. Bovine liver tissue irradiated with gamma dose of 43Gy |
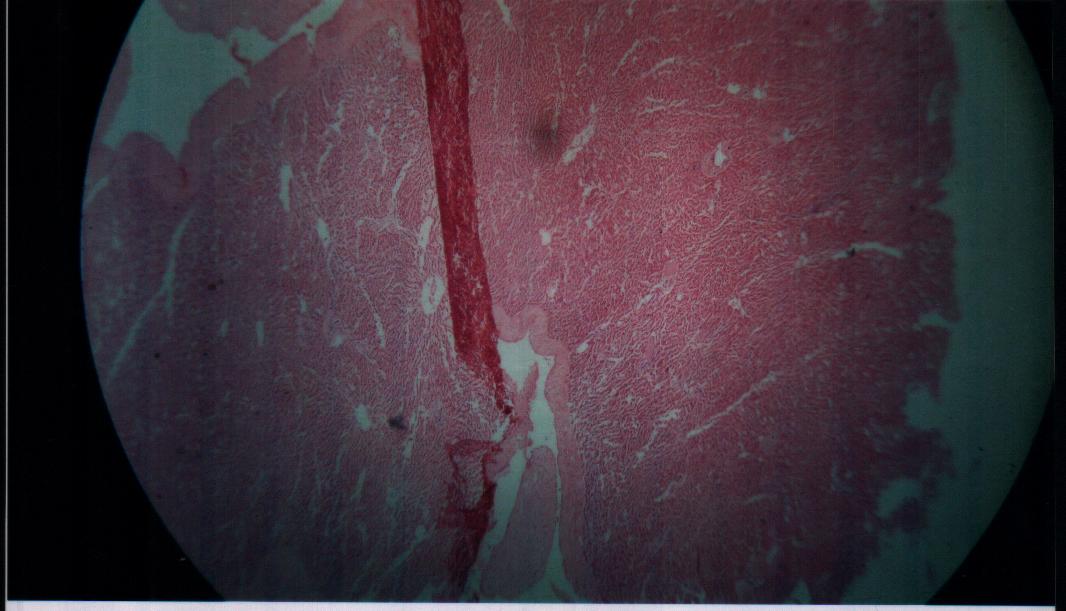 | Figure 13. Bovine liver tissue irradiated with gamma dose of 60Gy |
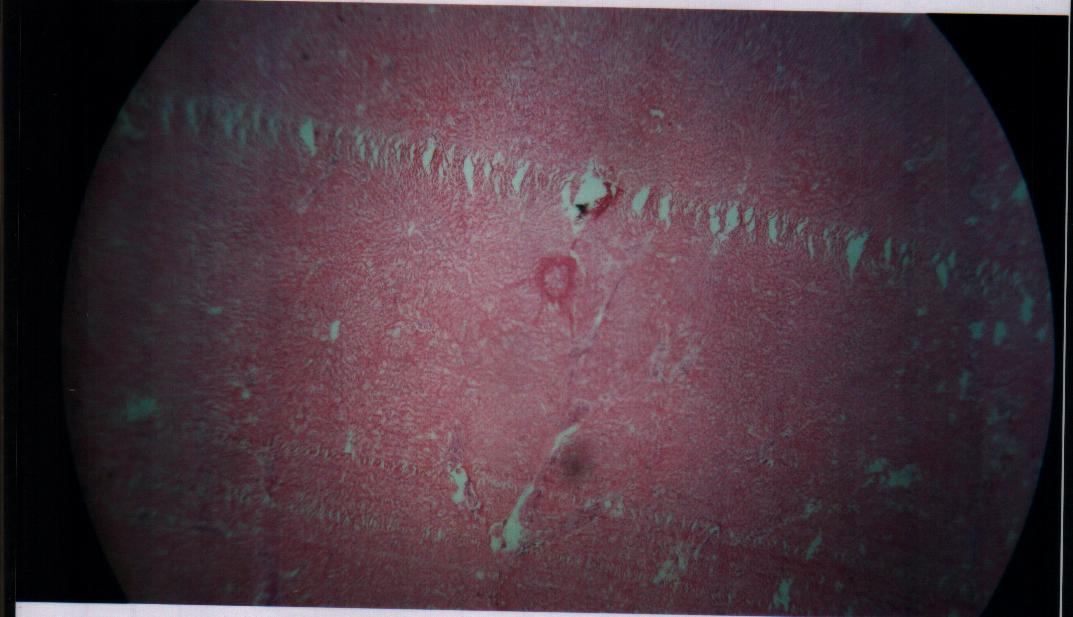 | Figure 14. Bovine liver tissue irradiated with gamma dose of 85Gy |
5. Conclusions
The threshold dose for induction of morphological changes in the irradiated liver tissue was observed at 11Gy. This work reveals that gamma-irradiation dosage usually encountered in diagnostic and therapeutic radiology has measurable effect on mammalian tissues and that the changes are dose dependent. Finally, the high degree of coefficient of fits demonstrates that this models are capable of predicting possible morphological changes in gamma-irradiated tissues.
ACKNOWLEDGEMENTS
We are grateful to Director General, Nigeria Atomic Energy Commission and the Director, Gamma Irradiation Facility, Nigeria Nuclear Technology Centre Abuja for irradiation of our samples in the course of this research. We are also grateful to the Technologists in the Department of Physics and BiologicalSciences, Benue State University, Makurdi, Nigeria for their assistance throughout the period of this research.
References
[1] | AAMP (1996).A Report of the Biological Effects Committee of the American Association of Physicist in Medicine published by the American Institute of Physics. New York, USA (3rd print). |
[2] | Agba E.H., Laogun A.A and Ajai N.O (2008). Comparism of the Effect of Diagnostic X-rays on the Radiofrequency Dielectric Properties of Bovine Liver with Bovine Kidney Tissues. Nigerian Journal of Physics, 20(1), 11. |
[3] | Grant E.H., Sheppard R.J and South G.P (1978). Dielectric Behaviour of Biological Molecules in solution. Clarendon Press, London. |
[4] | Gerber, A. (1982). The use of mathematical models in formulation of pollution control policies. An over vein, water science and technology, Great Britain. Vol. 14 pp. 1045-1053. |
[5] | Laogun, A.A (1986). Influence of pH on the dielectric properties of egg-white Lysozome in Aqueous Solution. J.Vol. Liq. 32, 111-119. |
[6] | Laogun, A. A. N. OAjayi and E.H Agba (2005): Influence of x-rays on the radiofrequency dielectric properties of Bovine kidney tissues. Nigerian Journal of Physics. Vol. 17 p. 117. |
[7] | Laogun, A.A., Agba, E.H and Ajayi, N.O (1997). A Comaprison of Dielectric Behaviour of Human Haemoglobin SC with SS and AA in solution. Journal of Physics, med. Biol., 42, 707-713. |
[8] | Mallidi, S.M., Bhilwade, H.N., Khan, M.Z. and Chamby, R.C. (2007). Gamma Ray Induced Genetic Changes in Different Organs of Chick Embryo using peripheral blood micronucleus test and comet assay at http://www.siencedirect.com/science? |
[9] | Pethig, R. (1991). Dielectric Properties of tissues. In Renato Dulbecco (ed), Encyclopedia of Human Biology. Vol. 3 DI - GI, Academic Press, San Diego. |
[10] | Russel K.H and Bradley J.R (2007). Intermediate physics for medicine and biology. Pp. 457-463. |
[11] | UNSCEAR 1993 Annex H: Radiation effects on the developing human brain. |
[12] | Whitehead, P. and O’Connell, E. (1981). A System of model of stream flow and water quality in the Bedford River System.Pergamon press, Great Britain, vol. 115, No. 10, pp. 1157-1171. |