M. G. Abrahamyan1, L. I. Matveenko2
1Yerevan State University, Yerevan, Armenia
2Institute for Space Researches, Russian AS, Moscow, 117997, Russia
Correspondence to: L. I. Matveenko, Institute for Space Researches, Russian AS, Moscow, 117997, Russia.
Email: |  |
Copyright © 2012 Scientific & Academic Publishing. All Rights Reserved.
Abstract
In frame of the hydrodynamics whirlwind mechanism of bipolar outflow formation from disk system the data of observations of active starburst region a dense molecular cloud of Orion KL in H2O maser radiation is analyzed. It is shown that the theory as a first approximation explains the basic observant laws of active region, structure accompanying protostar formation: a disk- a bipolar outflow, including its mechanism ejection and self-collimation, excitation maser radiation.
Keywords:
Maser H2O Radiation, Orion KL, Hydrodynamics, Whirlwind, Bipolar Outflow, Collimation
Cite this paper: M. G. Abrahamyan, L. I. Matveenko, Initial Stage of Star Formation, International Journal of Astronomy, Vol. 2 No. 3, 2013, pp. 23-29. doi: 10.5923/j.astronomy.20130203.01.
1. Introduction
Due to gravitational instability in gaze-dust complexes local active zones containing protostar are formed. This process has collective character. The mechanism of star formation and whirlwind structures accompanying them so far is not clear[1,2]. Direct researches of these structures were inaccessible because of their rather small angular sizes and rather low level of radio emission. The discovery of powerful Н2О maser radiation partially removed this restriction. But a tool with extraordinary high angular permission was necessary. Long-distance radio-interferometry solved that problem (VLBI[3]). It has been established that the lines of Н2О radiation have a maser origin which accompanies star formation processes. As have been shown in further research of gas-dust complexes, including observations with the ultrahigh angular resolution, maser radiation is an ideal indicator of these processes and allows defining three-dimensional structure, kinematics of active area at the initial stage of star formation. In some cases powerful flashes of maser radiation, for example, in object W 49 or dense molecular cloud ОМС-1 of the Orion nebula are observed[3, 4].Studies of Orion KL with the ultrahigh angular permission are conducted by us from the moment of the development of radio-interferometry, including on a global network. It is established that in cloud ОМС-1 compact maser sources are concentrated in 8 active zones[5]. This confirms V.A. Ambartsumyan's hypothesis about collective behavior of the starburst processes. In 1979 in Orion KL the increase of maser radiations on the speed v ≈ 8 km/s was registered[6]. During period of activity 1979-1987 radiation of separate flashes reached 8 MJy, and their duration did not exceed one month[7]. Radiation was defined high organizing structure: a chain of compact maser sources in length ~ 9 а.u. (Fig.1). Sources are concentrated in 4 groups, and their sizes did not exceed 0.02 AU. The diameter of circles below on the left on Fig.1 corresponds to the logarithm of brightness temperature ~lgTb. The maximum value of brightness temperature is equal Tb = 1017 K. Here are presented resulting speeds of components. Speeds of sources concerning local system of rest (v=7.65 km/s) presented below on the right of Fig.1 and correspond to homogeneous rotation vrot = Ωr, where r is the distance of a source from the rotation axis. The high organizing structure - a chain of component corresponds to tangential directions of the disk’s spiral structure - to an arm on which the substance flows down to the center[8]. The disk of diameter ~27 AU has bent edges and is observing from the edge (Fig.1). In the limits +/- 6.0 а.u. the gradient of rotational velocity equals dv/dr = 0.167 km/s/AU which corresponds to the period of rotation Т ≈ 178 years[8-11]. The rotational velocity of an external part of the disk (R ≥7 а.u.) is vrot ≈ 1.2 km/s. In the keplerian approach the equivalent mass of the central body and a disk does not exceed M <0.01
Environment (molecules of water steam) strengthens radiation on 2-3 order on the velocity v = 7.65 km/s in a bandwidth ~ 0.5 km/s, which explains mega-maser level of radiation of flashes and preservation of their velocity . Chain of maser radiating sources is presented below on the left. Diameter of circles ~ lgTb, maximum brightness temperature Tb = 1017 K. Profile of rotation velocity of sources in local system of rest depending on their position - below on the right.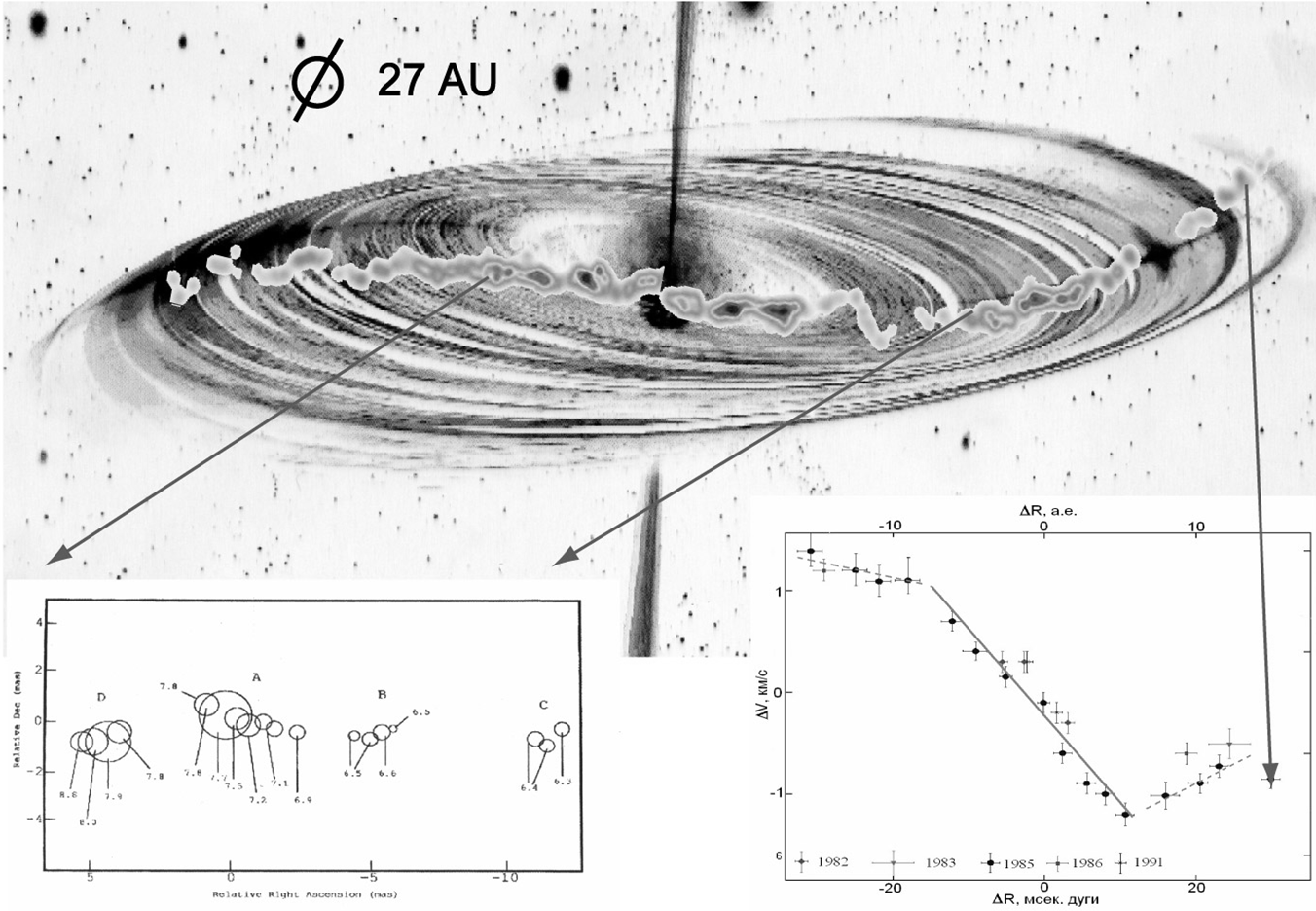 | Figure 1. Structure of the active region of Orion KL in Н2О maser radiation |
In "silence" period (epoch 1995) radiation on the speed v = 7.65 km/s did not exceed 1 kJy which was defined by a bipolar stream. This structure "was absent" in high activity period, probably because of the powerful radiation blending of the central group of a chain. There was no radiation from the component chain. One remote fragment of a stream on the distance ~25а.u. was observed only (Fig. 2[7-9]). "Disappearance" of radiation a chain component, possibly, was caused by that tangential directions of structure had a high orientation and concentrated in an azimuthal plane[11]. Lately the precession of a disk has caused their "disappearance".During the second period of activity 1998-1999 brightness of a bipolar stream has essentially increased. The maximum values of density of the maser radiation flow reached 4 MJy. A bipolar stream has the helical form with the dispersing step, defined precession of the axes of ejector, rotating with increasing angular speed. The period of precession corresponds to T ≤ 10 years[9, 11]. Wide wings in a profile of a bipolar stream’s radiation line can be caused its rotation with a period of about 5 months[12].The sizes of cross-section of a stream vary slightly, which testifies to the high efficiency of collimation mechanism, operating on distance exceeding 25 а.u. Velocity of water molecules in streams on an ejector exit ~ 5 km/s, and on distance ~1.5 AU increase to ~ 40 km/s.
2. Structure of an active zone of Orion KL in Н2О maser radiation
1. The active zone is located in the central part of the dense molecular cloud ОМС-1 whose size ~ 1 pc, hydrogen density in a core of cloud n(H2) ≈ 108 sm-3, the maintenance of molecules of water n(H2) / n(H2О) = 104, the kinetic temperature of gas does not exceed several tens K.2. The observant data in Н2О maser radiation corresponds to a disk and a bipolar stream.- Diameter of a rotating disk ≈ 27 AU. - The gradient of the rotational velocity of central part of the disk is equal ≈0.167 km/s/a.u. which corresponds to rigid body rotation with the period ≈ 178 years. - Velocity of rotation H2О in an external part of a disk (r ≥ 7 а.е.) it is equal vrot ≈ 1.2 km/s. - Distance between nozzles (bright fragments) 0.004 AU[12].- Velocity of water steam ≈ 5 km/s on the ejector exit and accelerated to 40 km/s on distance 1.5 AU. - The period of activity makes 1 - 2 years. - During a silence, the surrounding matter is tightened to a disk in 10-15 years - then comes a new phase of activity.3. Conclusions: the Structure accompanying an initial phase of a protostar formation corresponds to an anticentrifug mechanism which is soaking up the surrounding substance, and ejects it as a bipolar outflow. The given model is based on observable velocity maser lines - water steam. However velocity of the Н2О molecules can essentially differ from velocity of the molecular hydrogen making the basic part of matter. On a disk accretion, streams of hydrogen with ice and water molecules inclusions take part. Velocity of molecules of hydrogen owing to essentially smaller mass will exceed considerably the velocity of molecules of water and the more so, ice. The high- velocity hydrogen component of a stream sublimate ice blows off and excites molecules of water steam, causing observing maser radiation. For maintenance colliding a rating s velocity of molecules of hydrogen should exceed velocity of raised molecules more than on △v = √ (∆vr2 + ∆ vφ2) ≥10 km/s, and in different areas of a disk the contribution ∆vr and ∆vφ can be miscellaneous.Thus, proceeding from pumping conditions, velocity of molecules of hydrogen in all the visible area of maser radiation exceeds velocity Н2О molecules by more than 10 km/s. Molecules of water steam are accelerated about 5 km/s on an exit of a nozzle to 40 km/s at a distance of 1.5 AU[11]. The velocity of the stream will be not less than 50 km/s. It means that a profile of velocity of rotation of accreting matter in a disk it will considerably differ from the profile on Fig. 1. In particular, velocity of a hydrogen velocity on a peripheral part of a disk will be not 1.2 km/s, and ≥11.2 km/s and the equivalent mass of the central body will be ≥ 1 M ⨀.
3. The Hydrodynamic Theory of Bipolar Outflow Formation
The above-mentioned observational data can be explained, basically qualitatively and in some aspects quantitatively, within the framework of the hydrodynamic whirlwind mechanism of the astrophysical jet formation[15-17]. As a simple model, we will consider strongly flattened spheroidal (disc-shaped) gravitating body of mass M, with semi axes a≫b which is in an accreting disk of thickness 2h with homogeneous mass density ρ. It is possible to neglect self-gravitation of the accreting disc and consider it in a gravitational field of the spheroidal body. Let during the moment t = 0 rotational profile of the accreting disk is expressed by the formula 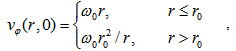 | (1) |
that represents homogeneous rotation to area r ≤ r0 and differential, corresponding to the disk accretion with conserving angular momentum - out of it. The structure (1) is known as a Rankin whirlwind[14-17]. In the formula (1) angular speed ω can vary in time and dependence on t in different areas of a disk can be different.As further on we are basically interested in the r ≤ r0 area of the accreting disk, we will consider the gravitational field in it as homogeneous with intensity | (2) |
Ω - an order of the angular velocity of disc-shaped spheroid of the average mass density ρ*. Obviously it represents angular speed ω0 of the r ≤ r0 accreting disk’s area at the initial moment t = 0.Dynamics of an accreting disk is described by the axially-symmetric Nave-Stokes equations in cylindrical co-ordinates[18]: | (3) |
 | (4) |
 | (5) |
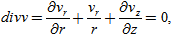 | (6) |
where vr, v φ, vz is the radial, azimuthal and longitudinal components of speed, and ν is the factor of kinematic viscosity. It is supposed that vr, v φ do not depend on z.The continuity equation (6) supposes the following exact solutions 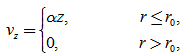 | (7) |
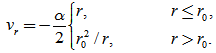 | (8) |
Here α is a generally time-dependent parameter and characterizing gradients of vr, vz in area r ≤ r0.These formulas specify in occurrence of rotation converging to an axis radial and longitudinal along an axis of rotation of bipolar streams of matter. Converging to a rotation axis the radial flow of matter transfers energy and the angular moment to area r ≤ r0 therefore angular speed of this area will start to grow. Taking into account (1) and (8) from the equation (4) it is received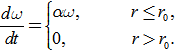 | (9) |
Considering α = const, we receive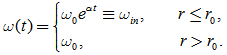 | (10) |
Hence, cylindrical area r ≤ r0 of an accreting disk starts to rotate exponentially accelerated rate while the external area continues to rotate at the initial angular velocity. It leads to discontinuity of rotation velocity on the cylindrical surface r = r0: | (11) |
where v0 = ω0r0 - initial velocity of rotation on a cylindrical surface r = r0.If the parameter α is proportional ω: α = βω, (α0 = βω0), that, integrating (9), we will receive  | (12) |
which corresponds to "explosive" instability of area r ≤ r0: formally, for final time Т = 1/α0 angular velocity, and together with it radially-converging and vertical bipolar streams speeds tend to infinity. Actually "explosive" increase will be broken at some level by effects of compressibility of environment.Let's especially notice that relations (12) concern only to area r ≤ r0. In r > r0 area, as ω = ω0, α0 = βω0 are constant.Velocity (1), (7), (8) identically turn zero the viscous terms in equations (3) - (5) while diagonal components of the viscous tensor are distinct from zero. It leads to the following capacity of kinetic energy dissipation on the unit length of a whirlwind: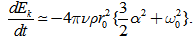 | (13) |
Let's notice that the energy dissipation does not depend on time and remains constant, despite the fast growth of angular velocity of rotation.Let's define pressure distribution in a developing whirlwind. Taking into account (1), (7), (8) from the equations (3), (5) it is found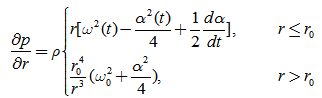 | (14) |
 | (15) |
Integrating these equations, for a difference of pressure between circles r = r0, z = h, and r, z during any moment of time we will receive | (16) |
If such two circles are on the same isobar the left part (16) will vanish. Such isobar in area r ≤ r0 is described by the formula | (17) |
And in area r > r0 - | (18) |
The isobar described by formulas (17) represents a funnel going deep in time. And the external part of a funnel (18) does not vary in time. r ≤ r0 the isobar funnel develops in areas faster, moving deep into accreting disk (fig. 2). The funnel bottom is located on a whirlwind axis r = 0 and the law of change of its position in time, in a case α = constant, expressed by the formula: | (19) |
and at "the explosive" law of increase:
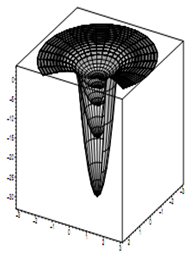 | Figure 2. Evolutions of the isobar funnel (different shapes correspond to different equidistant times of vortex evolution) |
Accepting in (19) zin (0, τ) = b, we will receive time τ, for which bottom of a funnel will concern poles disc-shaped spheroid: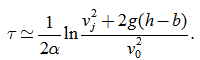 | (20) |
Here we have neglected a term vr2 (r0), and have considered a condition b2≪h2 in (19).Research has shown[14-16] that exponential growth of angular velocity of r ≤ r0 area stops and the whirlwind saturates when jump of rotational velocity (11) on a surface r = r0 reaches sound velocity cs. It occurs because of the absolute instability of a tangential velocity discontinuity surface. As a result around the surface r = r0 arises the thin transitive turbulent layer (with an abnormal high turbulent viscosity[15]) which breaks inflow of energy from external area and stops the further acceleration of a whirlwind. In a case α = constant the whirlwind reaches the saturation in time 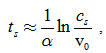 | (21) |
and at "explosive" increase of speeds
Let's accept further α = constant.The thickness of a transitive layer ℓ is defined by the equation | (22) |
where ℓ0 has the order of quantity of free run length of disc particles. The maximum value of factor of turbulent viscosity in a transitive layer thus appears equal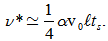 | (23) |
Time of saturation of a whirlwind (21) surpasses time (20) for which bottom of a funnel will concern poles of more dense disc-shaped spheroid. Hence, the sated whirlwind will interact with spheroid on a circular area of radius
As a result of this interaction, first, the whirlwind will soak up its more dense matter outside, forming narrower and denser stream. This phenomenon is often observed at terrestrial tornadoes. And, secondly, because of the difference of speeds of rotation (of an order (cs-v0)/2) between a transitive layer with abnormal high turbulent viscosity, and a spheroid, the big energy will be allocated here, causing observable powerful maser radiation of these fragments.So, the disk, with a profile of rotation (1), cannot be in a steady state. It should be compressed and eject a rotating bipolar stream of substance (fig.3) whose speed on the surfaces of the accreting disk equals | (24) |
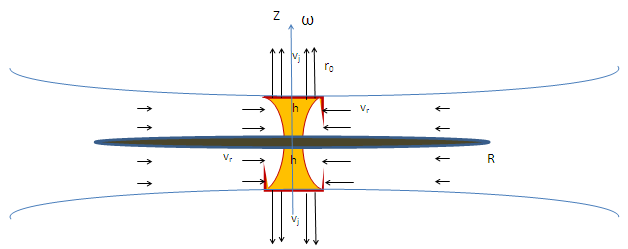 | Figure 3. Formation of bipolar outflow |
4. Discussion of Results
As we can see, the stated hydrodynamic theory of bipolar outflow formation from disk system qualitatively explains the basic observant laws of active area in Orion KL[9-13]. However, because of incompressibility of the considered model, there are some difficulties in quantitative estimations of some characteristics. For an illustration of the quantitative conformity of the developed theory with observing data we will consider, for example, the following situation.Parameters of the disc-shaped spheroid: M
1033g. As polar radius we will accept half of distance between two bright fragments of streams on exits of ejector: b
2·10-3a.u.
3·105km. Equatorial radius: a = 3·108km. Then for intensity of a gravitational field and average volume mass density of the disc-shaped spheroid we will receive: | (25) |
and for its angular velocity: Ω
2·10-6s-1.Parameters of the accreting disk: Here we will accept that the profile of rotation molecular accreting disk not precisely coincides with a profile of velocity of rotation of water "steams". More precisely, for radius r0 areas of homogeneous rotation we will accept an order 0.1а.u.: r0
107km.Semi thickness: h
3·107km, concentration of particles: nH2
1010см-3. Radius of the accreting disk: Rm
3·109km.At the moment t = 0 we will accept the angular velocity of rotation of the r ≤ r0 disk area as equal to angular velocity of the disc-shaped spheroid:  | (26) |
Then for the rotation velocity of the disk on distance r = r0 it is received  | (27) |
Velocity of a radial-converging stream vr (r0) on border r = r0 as is specified by observation, has one order smaller value: | (28) |
We will accept the sound velocity in a disk as cs ≅ 40km/s.By the formula (8) we receive the estimation for parameter α0: | (29) |
Then for the velocity of the bipolar stream on a pole of the disc-shaped spheroid we will receivevj (b)
αb
1km/s,and on an exit from accreting disk - | (30) |
For angular velocity of streams rotation at α = constant on an exit from the disk it is received
which corresponds to the period of rotation of an order of a month. Getting to more rarefied interstellar environment, a stream will extend, sharply reducing angular velocity, and on some distances forming in the central part of the outflow Rankin whirlwind, which will collimate and accelerate the stream, as it is shown in[17].Isobaric funnels reach spheroid poles in time, an order of month: | (31) |
The time of saturation of the whirlwind (21) is almost twice as big as τ. The isobaric funnel will interact with disc-shaped spheroid in a circular area of radius  | (32) |
soaking up its denser matter and throwing outside as a narrower bipolar stream. On a spheroid polar region the relative speed of a funnel makes ~20km/s. If we take into account an abnormally high turbulent viscosity of a transitive layer, it is clear that rather big energy here should be allocated. Existence of two active zones powerful maser radiations on distance 2b (~0.004 AU[12]) from each other in the disk’s central region, it is possible to interpret as a result of the interaction of an isobaric funnel of accreting disk with polar regions of denser disc-shaped spheroid.Let's estimate now the time of an active phase of the given mechanism. As energy and the angular moment in the field of a bipolar stream r ≤ r0 turn out from external area r>r0 of a disk, the maximum value of ejected energy in a period of activity is equal to | (33) |
Dividing it into a longitudinal flow of energy of the stream: | (34) |
we receive the duration of an active phase of the expiration: | (35) |
During the time of a passive phase of outflow the accreting disk filling of substance that makes | (36) |
Thus, apparently from the cited research the observant data of active area of star formation in the field of Orion KL is precisely enough described by the hydrodynamic mechanism and corresponds to an anti-centrifuge.
5. Conclusions
Our studies of the active region of the H2O maser emission in Orion KL shown:→The H2O maser emission is accompanying the star formation.→The region structure consists of rigid body rotated disk and high collimated bipolar outflow.→The gas-dynamics show that surrounding matter is accreted to the disc, followed along arms to central part, and ejected bipolar outflow.→The interaction with surrounding medium is collimated and accelerated outflows.→The hydrodynamics instabilities of the outflows are determined its helix structure with increased step.→The extra ordinary conditions in the central part of the disk are determinate formation of the massive body – star.→The active region of the star formation is similar cosmic tornado – anti-centrifuge.One of the authors (MGA) expresses gratitude to the State Committee of Science of Armenia for support of this work within the limits of the grant 11-1С107 and (LIM) - for support of the given research under the program of Presidium of the Russian Academy of Sciences «The Origin, a structure and evolution of objects of the Universe» and the hospitality shown by the Yerevan State University.
References
[1] | V.A.Ambartsumyan. Works of 2nd meeting on cosmogony. M. AS of the USSR, 1953. p.9. |
[2] | V.A.Ambartsumyan, Ann. Rev. Astron. Astroph., 18, 1-13, 1980. |
[3] | B.F.Berk L.D.Dzhonston, V.A.Efanov, et al., Radiofizika, 5, 799-802, 1973. |
[4] | L.I.Matveenko, L.R.Kogan, V.I.Kostenko, Pis’ma Astron. J., 8,505-508, 1980. |
[5] | R.Genzel, D.Downes, J.M.Moran, et al., Astron. Astroph., 66, 13-29, 1978. |
[6] | L.I.Matveenko, Pis’ma Astron. J., 12, 100-105,1981. |
[7] | L.I.Matveenko, F.D.Diamond, D.A.Grem, Astronomicheskij J., 77, 9, 669, 2000. |
[8] | L.I.Matveyenko. «Low mass stars-formation and bipolar outflows», JENAM-2007, Yerevan 2007, № 2, p.7. |
[9] | L.I.Matveenko, K.M.Zaharin, F.D.Dajmond, D.A.Grem, Pis’ma Astron. J., 29, 723-726, 2003. |
[10] | V.A.Demichev, L.I.Matveenko, Astronomicheskij J., 81, 12, 1074, 2004. |
[11] | L.I.Matveenko, V.A.Demichev, S.S.Sivakon, F.D.Diamond, D.A.Grem, Pis’ma Astron. J., 31.12. 913, 2005. |
[12] | L.I.Matveenko, S.S.Sivakon, Pis’ma Astron. J., 34. 12. 908, 2008. |
[13] | L.I.Matveenko, Astronomicheskij J., 81, 8, 726, 2004. |
[14] | W.J.M. Rankine. Phil. Mag. Ser. - 4, 39, 211, 1870. |
[15] | M.G.Abrahamyan. Astrophysics. 51, 201, 2008. |
[16] | M.G.Abrahamyan. Astrophysics. 51, 431, 2008. |
[17] | M.G.Abrahamyan. Astrophysics. 52, 136, 2009. |
[18] | L.D.Landau, E.M.Lifshits. Hydrodynamics. Nauka, M. 1986. 736с. |