Pranita Das 1, H. L. Duorah 1, T. Bezboruah 2, Kalpana Duorah 1
1Department of Physics, Gauhati University, Guwahati, 781014, India.
2Department of Electronics, Gauhati University, Guwahati, 781014, India
Correspondence to: Pranita Das , Department of Physics, Gauhati University, Guwahati, 781014, India..
Email: |  |
Copyright © 2012 Scientific & Academic Publishing. All Rights Reserved.
Abstract
The strong constraint on the baryonic density parameter for the consistency check on the production of light elements in the early universe is the main argument for the existence of non -baryonic dark matter. The non-baryonic dark matter is considered to be made up of neutrinos and axions, where the latter decays into photons in strong magnetic fields. Using the measurements of the anisotropy of the CMB, the spatial distribution of galaxies, one finds a density of non-baryonic dark matter to be
. Using the constraint on neutrino density parameter as
for massive neutrinos
, the neutrino number density calculated from Goldreich- Julian technique is taken as the standard value for their production .The rest of the non-baryonic components, the axions, are then estimated. These axions are believed to power the magnetar gamma ray energy, which agree satisfactorily with the observed values. Magnetars possess ultra high magnetic fields are the potential sources of neutrinos and axions consistent with non baryonic dark matter production elucidated here then give rise to SGRs. For still higher magnetic field the energy production in magnetars may account for even the energy of Gamma ray burst sources (GRBs).
Keywords:
Non-Baryonic Dark Matter, Universe Mass Density, Soft Gamma Ray Emission
1. Introduction
Qualitative production of inflation idea is that the universe should be essentially flat i.e.
.To have
, there must be a form of non-luminous matter in the universe. The density of baryons needed to make
now would suppress the production of deuterium in the early universe contrary to the observation. Inflation is not compatible with a purely baryonic universe since
[1]. According to some cosmologists the most successful model to date seems to need plenty of non-baryonic dark matter. The amount of dark matter that exists determines the fate of the universe. And also several independent observations point to the existence of non-baryonic dark matter in the universe. The strong evidence for the existence of non-baryonic dark matter came in the form of massive neutrinos. Axion is also believed to be the dark matter candidate. The current status of axion as dark matter has been reviewed by Duffy et al[2]. The contribution of non-baryonic dark matter to universe mass density is found to be: | (1) |
where h is Hubble constant in units of 100km/(s.Mpc)[3].We assume here that the neutrinos and axions make up the non-baryonic dark matter that is formed in the universe. We know for sure that the neutrinos are produced abundantly in the core of the magnetars. Magnetars are the type of neutron stars having strong magnetic field much higher than quantum critical value
[4]. It is suggested that a new born magnetar combined with convection pattern in its interior, giving it a highly efficient dynamo, which build up such an enormous field[5].For most observed neutron stars, the dominant source of energy is the rotation of the stars[6]. Magnetars have long rotation period. In magnetar the magnetic energy exceeds the rotational energy by an order of magnitude[6]. Magnetar's intense magnetic field is the result of its rapid rotation[7]. The decay of magnetic field powers the emission of high-energy electromagnetic radiation, particularly X-rays and gamma rays[8]. Magnetar spins down through magnetic waves very quickly due to strong magnetic field[5]. The theory regarding magnetar was first proposed by Robert Duncan and Christopher Thompson in 1992[5]. The Anomalous X-ray Pulsars (AXPs) and the Soft Gamma Repeaters (SGRs) are considered as the two classes of magnetars[4]. Soft gamma ray repeaters (SGRs) are strange and relatively rare and exotic objects. They are believed to be stars with magnetic fields that are up to 1000 times stronger in comparison with the usual neutron stars. They spin very rapidly at 2-3 milliseconds and this rapid rotation builds up such an enormous field. Data from XMM Newton are found to suggest that photon – axion conversion may take place in a magnetic neutron star. In the magnetized plasma of neutron stars photons can convert into axions which then modify the radiation spectra and polarization signals. The first SGR was observed on 7th January 1979 and on 5th March gamma ray burst of a SGR was detected for the first time[4]. SGR emits bursts in hard X-ray/Soft gamma -ray range with the luminosity at peak of the burst
and earlier it is also considered as a sub-class of gamma ray burst[9]. And sometimes it emits X-ray in quiescence with luminosity
. Till now four SGRs (1806-20, 1900+14, 0525-66 and 1627-41) are discovered and studied in detail[10]. Anomalous X-ray Pulsars emit in the soft X-ray range with luminosity
[9] . Massive neutrinos, component of dark matter are emitted from these magnetars[11]. The bremsstrahlung emission of neutrino pairs or axions arises from nucleon spin fluctuations in collision. There are several reactions that produce neutrino in magnetar. Table1. Processes of neutrino emission[12] 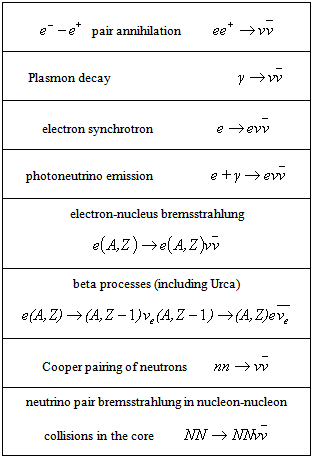 |
| |
|
Another possible dark matter component, axion is also produced in these objects. Deog Ki Hong studied emission of axions in strongly magnetized star[13]. He found that if
in strongly magnetized stars, the axion emission rate is enhanced by several orders of magnitude.The strong magnetic field of these objects affect the emission of axion and axion decays into two photons[14]. Therefore in magnetar, axion plays a great role in photon emission.
Under the condition of the core of the hot neutron star just after their formation, the dominant axion emission process is nucleon-nucleon, axion bremsstrahlung[15]:
So, this bremsstrahlung process can also be used as source of axion in highly magnetised neutron star. The density of the electron component in the region of closed field lines of magnetar exceeds Goldreich–Julian density by several orders of magnitude. Under this condition Mikheev et al. studied the resonant production of axions[16]. The search for observational appearance of dark matter axions using radio observations of neutron stars was suggested by Pshirkov et al[17]. Again in 1998 Mikheev et al have studied the photon decay with interaction of pseudo scalar particle, axion with two photons induced by an external electromagnetic field[18]. And they analysed axion emission by photon as a possible source of energy losses by astrophysical objects. Axion emission affects the evolution of stars particularly hot neutron stars[19]. Axion plays an important role in cooling of these nascent neutron stars. Axion emission cools the inner core rapidly[19]. Axions are pseudoscalar particles that arise in the Peccei- Quinn solution of the strong CP problem. They are light neutral spin zero bosons and they have the characteristic property of being converted into photon. Axion behaves as a cold dark matter candidate because it satisfies the conditions required for cold dark matter :(1)a non-relativistic population of axions could be present in our universe in sufficient quantities to provide the required dark matter energy density and (2) they are effectively collisionless[20].Axions can be detected through their weak interaction with matter. In the early universe axions are produced due to both thermal and non-thermal processes[19]. At high temperature the dominant axion production process is photo-production.Allowed range of axion mass is[17] | (2) |
The other non-baryonic matter neutrino known as hot dark matter candidate is a weakly interacting and electrically neutral particle with spin half. Neutrinos are produced in the early universe. They are fermions and relativistic particle. It has three flavours:
,
and
. Using data from the largest ever survey of galaxies, researchers found the mass of a neutrino at no greater than 0.28 eV. The neutrino density parameter is predicted to be[14]: | (3) |
where
is the mass of neutrino. Here an attempt is made to study the gamma ray emission in magnetar as SGR with dark matter components neutrino and axion in proportion to their contribution to the universe mass density. A comparison is also made with axion emission rate due to nucleon-nucleon, axion bremsstrahlung.
2. Calculation
Nucleosynthesis data say that baryonic matter makes up 0.04 of the critical cosmological density[21], assuming
. It is believed that the universe is dominated by non baryonic dark matter in the form of particles like neutrinos and axions. The temperature fluctuation of CMB radiation can be explained with non baryonic drak matter such as massive neutrinos[21]. The non-baryonic nature of dark matter for both axion and neutrino has played a crucial role in determining the structure from galaxies to the large scale arrangement of superclusters of galaxies. Studying the baryonic matter in the intergalactic medium (IGM) between quasar and our galaxy, the mean number density of baryons
in the IGM as a function of redshift is[22] found to be proportional to
. Therefore it is assumed that number density of neutrino
is also proportional to
. Here we have used neutrino and axion as non-baryonic dark matter for understanding
- ray emission in magnetar.It is known that neutron star, GRB are the sources of neutrino. Bing Zhang et al[11] have considered the production of neutrinos via photomeson interactions in the magnetosphere of magnetar. The amount of neutrino emitted from these magnetars per unit time is estimated as[11] 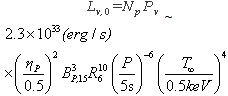 | (4) |
where
is the neutrino emission power , | (5) |
and
be the total number of protons,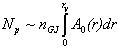 | (6) |
where
is Goldreich-Julian number density.Finally neutrino luminosity is[11]: | (7) |
where
is the correction factor for cooling or reacceleration.Considering
and
eq(7) can be rewritten as: | (8) |
where surface magnetic field at the pole in units of
,
and the neutron star radius
in
cm unit are given.
and
are spin period and the observed blackbody temperature for SGR/AXP quiescent emission[11] respectively. The observed black-body temperature for SGR/AXP quiescent emission is KT~0.4-0.6keV[11]. Since we have assumed
is in proportion to its contribution to the mass density of the universe therefore this estimated neutrino luminosity must correspond to an energy density in the universe equivalent to
for
. For axion dark matter produced in the same environment, the contribution to the total energy density can be calculated from this neutrino energy density. We assume that
in magnetar and therefore axion luminosity is. 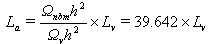 | (9) |
Thus the luminosity due to axion is estimated by standardizing the contribution of neutrino to the mass density of the universe with the non-baryonic dark matter density. The calculated axion luminosity is shown in Table2 for various values of
determined from eq(8) for different magnetic field strengths.Table 2. Luminosity due to non-baryonic dark matter neutrino and axion along with magnetic field 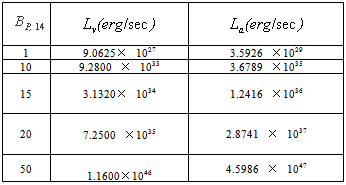 |
| |
|
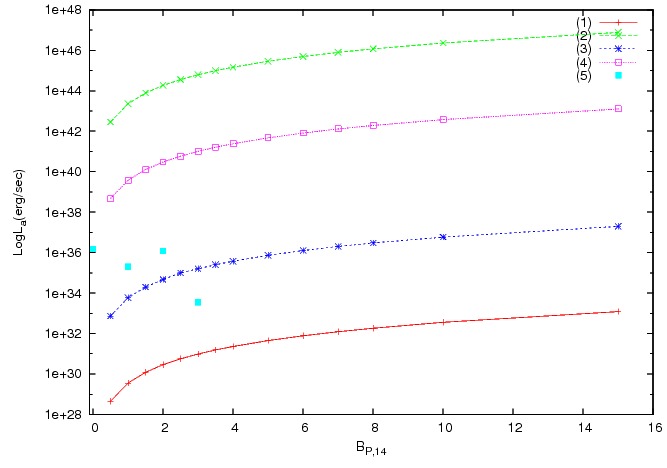 | Figure 1. Luminosity as a function of Magnetic field. (1), (2), (3) and (4) indicate curves for different values of R6, and P. Some of the observed values of energy emission from SGRs are shown by (5) |
It is seen that the luminosity due to axion always exceeds luminosity due to neutrino. The calculated axion luminosity for different values of radius, temperature, spin period and polar cap magnetic field is shown in Fig1. The observed -ray emission rate of some known SGRs(1806-20, 1900+14, 0525-66 and 1627-41) are also shown in Fig1 for comparison.
3. Nucleon-Nucleon, Axion Bremsstrahlung Process(NNAB)
In neutron star one of the important processes of axion emission is the nucleon-nucleon bremsstrahlung process. Three bremsstrahlung processes are important. | (10a) |
 | (10b) |
 | (10c) |
In terms of matrix element squared,
, the axion emission rate is given by[19]
 | (11) |
where
, the labels
indicate the incoming(1,2) and outgoing (3,4) nucleons (neutrons and proton), ‘a’ denotes the axion.
is the symmetry factor for identical particles in the initial and final states,
is summed over initial and final spins. The nucleons are in semi-degenerate state in the core[19].The axion emission rate is estimated as [15,19] | (12) |
Axion luminosity is therefore, | (13) |
Here it is assumed that number of neutron=number of proton .For
and
, the axion luminosity is found to be
. It amounts to the same energy as estimated in our work.
4. Conclusions
The neutrinos are presently considered as massive and so they can make a significant contribution to the total energy density of the universe. They arise from the constraint on the observable baryonic matter consistent with production of light element. And it is found that in the presence of strong magnetic field, the axion dark matter could reasonably decaying into two photons.Here we have studied
--ray emission in magnetar as Soft Gamma Repeaters with dark matter components axion and neutrino. All the observed energy emissions from SGRs are found to conform within the estimated axion luminosity. Comparison with NNAB process makes the whole matter self consistent and it can be proposed that magnetars are powered by axion. It is found that the magnetic field of known SGRs are within
which agrees with the observation. If magnetic field is increased to
, the energy rate can be found in the Gamma Ray Burst range
. Axion luminosity is found to increase with magnetic field as shown in Fig1. This appears to be a suitable way to explain the enigmatic energy generation in magnetar.
ACKNOWLEDGEMENTS
The authors acknowledge the support received from DST, project No:S2/SR/HEP-02/2007.
References
[1] | J.V. Narlikar and T. Padmanabhan , Annu. Rev. Astron. Astrophys. 1991. 29: 325-362. |
[2] | Leanne D. Duffy and Karl van Bibber, New J.Phys.11:105008,2009 |
[3] | Ofer Lahav and Andrew R. Liddle, arXiv: 1002.3488v1[astro-ph.co]. |
[4] | Chryssa Kouveliotou,Proc.Natl. Acad. Sci. USA Vol.96 , pp. 53515352,May 1999. |
[5] | Duncan & Thompson, Ap.J. 392, L9-L13, 1992. |
[6] | J.S. Heyl, arXiv:astro-ph/0504077v1. |
[7] | Richard N. Boyd, “An Introduction to Nuclear Astrophysics” , University Of Chicago Press, 2008. |
[8] | Duncan & Thompson, Ap.J. 473 : 322-342, 1996, Goldreich, P., & Reisenegger, A., Ap.J., 395,250, 1992. |
[9] | Sandro Mereghetti , arXiv:0804.0250v1[astro-ph]. |
[10] | L. Zhang and K.S. Cheng, The Astrophysical Journal,579:716-724,2002. |
[11] | Bing Zhang, Z. G. Dai, P. Meszaros, E. Waxman and A. K. Harding, 2003, ApJ. ,V595. |
[12] | D.G. Yakovlev, A.D. Kaminker, O.Y. Gnedin and P. Haensel, arXiv:astro-ph/0012122v1. |
[13] | Deog Ki Hong, Phys.Lett. B445 (1998) 36-42. |
[14] | David Spergel, arXiv:astro-ph/9603026v1. |
[15] | Ralf Peter Brinkmann and Michael S. Turner, Physical Review D, Vol.38, No.8, 1988. |
[16] | N. V. Mikheev, D. A. Rumyantsev, and Yu. E. Shkol’nikova, JETP Letters, 2009, Vol. 90, No. 9, pp. 604–607, 2009. |
[17] | M. S. Pshirkov and S. B. Popov,Journal of Experimental and Theoretical Physics, 2009, Vol.108, No. 3, pp. 384-388. |
[18] | N.V. Mikheev, A.Ya. Parkhomenko, L.A. Vassilevskaya, Mod.Phys.Lett. A13 (1998) 1899-1905. |
[19] | Edward W. Kolb and Michael S. Turner, "The Early Universe", Westview Press, 1994. |
[20] | Leanne D. Duffy and Karl van Bibber, arXiv:0904.3346v1[hep-ph]. |
[21] | Jaan Einasto, Astronomy and Astrophysics 2010[Eds. Oddbjorn Engvold, Rolf Stabell, Bozena Czerny, John Lattanzio], in Encyclopedia of Life Support Systems (EOLSS), Developed under the Auspices of the UNESCO, Eolss Publishers, Oxford ,UK. |
[22] | Peter Coles and Francesco Lucchin, "Cosmology The Origin and Evolution of Cosmic Structure",2002, Second Edition,John Wiley & Sons, Ltd. |