S. Kalita, K. Duorah, H. L. Duorah
Department of Physics, Gauhati University, Guwahati, 781014, Assam
Correspondence to: S. Kalita, Department of Physics, Gauhati University, Guwahati, 781014, Assam.
Email: |  |
Copyright © 2012 Scientific & Academic Publishing. All Rights Reserved.
Abstract
The “cosmological constant” being an observationally satisfactory candidate for dark energy is treated as a constant energy density of vacuum. The possible variations of both “gravitational constant” and the “cosmological constant” during the early Universe have been constrained by Big Bang Nucleosynthesis (BBN). The BBN is highly sensitive to the early Universe expansion rate which is in fact governed by the “gravitational constant” as well as the “cosmological constant”. It manifests in the variation of primordial elemental abundances for helium (
and lithium (
, through elevation of the neutron-proton freeze-out temperature. Such elevation is equivalent to diminution of neutron-proton mass difference (Q). An effective “gravitational constant” is formed by expressing it in terms of the “cosmological constant” and the background energy density. The permitted range of the “cosmological constant” leads to that of primordial helium which has been then utilized to constrain the variation of the “gravitational constant”.
Keywords:
Gravitational Constant, Cosmological Constant, Freeze-Out, Vacuum Energy
1. Introduction
The idea that fundamental constants may vary with time, dates back to Dirac[1]. Although there is no robust evidence yet for such variation, many extensions of standard physical theories like superstring theory, scalar –tensor gravity predict that “fundamental constants” may have variation in time. In absence of any underlying physical principle in recent time, for predicting these fundamental constants, one can at least put some constraints on their variations which do not affect the evolution of the universe we see today. In cosmological setting, the impact of the fundamental constants can be best explored during the era of Big Bang Nucleosynthesis (BBN) as it is the earliest event in the history of the universe for which we can obtain solid and well testable predictions. The variations of these “fundamental constants” can be checked against the response of the cosmological evolution during BBN when light elements like deuterium, helium and lithium were being synthesized. As the large scale evolution of the universe is governed by two parameters namely “cosmological constant” (
and the “gravitational constant” (
appearing in the theory of gravity, we will consider them in our work. The “cosmological constant” acting as a successful candidate of dark energy[3,4] is reminiscent of gravitational repulsion. It is the energy density hidden in empty space and models the vacuum energy. It is responsible for expansion of space right after the Big Bang that has made our universe so large. On the other hand, the “gravitational constant” together with the “cosmological constant” determines the formation of the large scale structures like galaxies and clusters of galaxies. Therefore their permissible variations can be useful tool for examining the behavior of the cosmological evolution.In an earlier work[2], Kalita et al. have shown how the early expansion rate can be enhanced by a “cosmological constant” and hence resulting in elevation of the freeze-out temperature[2] which in turn elevates the abundances of both deuterium and helium. Response of the primordial abundances due to general modification of gravitational constant, G was studied by Bambi et al.[5].This work attempts to put constraint on variation of “gravitational constant” permitted by presence of a “cosmological constant”. The “gravitational constant” is found to be related to presence of vacuum energy which manifests as the “cosmological constant” and also by background energy distribution. An “effective gravitational constant” results.The basic idea in our work is that elevation of freeze-out in the BBN era cannot distinguish between an “effective gravitational constant” and a “cosmological constant”. The shift in the “gravitational constant” in our work is found to be mild compared to that found by Bambi et al. The variation of primordial helium abundance due to that of “cosmological constant” is used to find variation of the “gravitational constant” for maximal shift of neutron-mass mass difference which is equivalent to elevated freeze-out[2]. A shift in the primordial lithium is also found by such variation. The paper is organized as follows. Section 2 includes the effect of
and
on early expansion rate and hence on freeze-out. It also introduces the “effective gravitational constant”. Section 3 displays the results of variation of the “cosmological constant” on primordial helium and deuterium abundance as found by Kalita et al.[2]. It also includes the variation of the “gravitational constant” and also primordial lithium. Section 4 concludes.
2. Effect of
and
on Early Expansion Rate
The generalized expansion rate is governed by the Friedmann equation in relativistic cosmology, with the scale factor
and energy (mass) density
: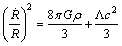 | (1) |
Equation (1) defines an “effective gravitational constant” which is related to “cosmological constant” and also the background energy density of the universe.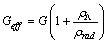 | (2) |
This may point out that fundamental constants may be determined by properties of vacuum itself which manifest in the form of “cosmological constant” at least in our observable universe. Here
is the energy density in the early universe when it was radiation dominated. The enhanced expansion rate due to “cosmological constant” yields an elevated freeze-out of neutron-proton ratio. An enhanced expansion rate means earlier freeze-out and hence more number of neutrons which in turn means more amount of helium. On the other hand enhanced expansion rate cools the universe faster leaving more unburnt deuterium[2]. The elevated freeze-out (in the unit of
is given by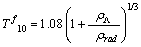 | (3) |
The enhanced neutron fraction is given by[2],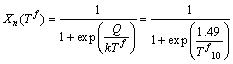 | (4) |
On the other hand the freeze-out elevation due to shift in “gravitational constant” during BBN, is given by Bambi et al.[5]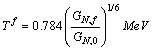 | (5) |
where,
and
are “gravitational constant” during freeze-out and at present time respectively. On the other hand the “effective gravitational constant” as argued by us gives the freeze-out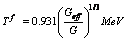 | (6) |
As the elevation of freeze-out cannot distinguish between “cosmological constant” and an “effective gravitational constant” we can parameterize the “gravitational constant” by the neutron-proton mass difference (Q) as 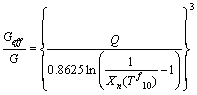 | (7) |
The elevated freeze-out is equivalent to the decrease in Q value from 1.293 MeV to 1.279 MeV as resulting from equation (4)[2]. We can now find out the variation of the “gravitational constant” for enhanced neutron fractions
parameterized by the Q value. As enhanced neutron fraction is related to enhanced fraction of helium and deuterium[2], we can conclude that the variation is permissible from BBN consideration.
3.
and Response of Primordial Element Abundances
The enhanced neutron fraction can influence on the total baryonic density parameter (
and also the total matter density parameter (
which are displayed along with the range of values of the “cosmological constant” in the form of the vacuum energy density parameter (
in table 1.Table 1. The constraint on “cosmological constant” permitted by BBN. (h is reduced Hubble parameter, ) 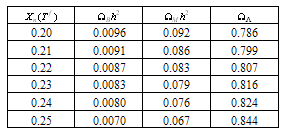 |
| |
|
It is seen that the highest neutron fraction corresponds to the highest value of the “cosmological constant” which in turn points towards a smaller value of neutron-proton mass difference (Q). As argued by Kalita et al. in[2] the neutron-proton mass difference can go down from 1.293 MeV to 1.279 MeV. The variation of the “gravitational constant” permitted by that of helium fraction () resulting from the enhanced neutron fraction is shown in table 2 and table 3. The baryon-to-photon ratio ( which plays an important role during BBN era is also calculated by using Bambi’s relation[5]Table 2. Shift in the “gravitational constant” for Q=1.293 MeV 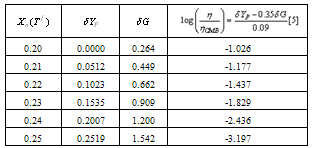 |
| |
|
Table 3. Shift in the “gravitational constant” for Q=1.279 MeV 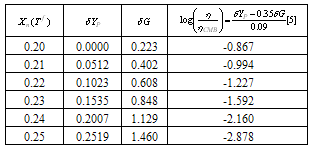 |
| |
|
It is observed that a decreasing value for neutron-proton mass difference (Q) i.e. a higher value of “cosmological constant” or vacuum energy density favors a mild variation of “gravitational constant”. It is essential to have a mild variation for G rather than an abrupt change in the early universe so as to have a more habitable universe for gravitating structures.Apart from helium and deuterium (the later being not shown in this work) the most important element that was synthesized after the Big Bang is lithium (). It is the most crucial element which can be used as consistency check for BBN[8 ]. The response of the primordial lithium to change in the “gravitational constant” is given by the relation[5] | (8) |
where
[5] is some response parameter. For the standard value for Q, the shift in the lithium abundance (
) is shown below.The variations show that a variation of the “gravitational constant” in presence of the “cosmological constant” during BBN can explain low lithium abundance compared to the standard scenario. This may help us understand the cosmological lithium discrepancy that the predicted lithium abundance in standard BBN calculations is higher than the value measured in the atmosphere of old stars[9].Table 4. Variation of lithium abundance with Q=1.293MeV 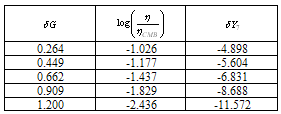 |
| |
|
4. Conclusions
The vacuum energy density or a “cosmological constant” with permissible range may be an essential ingredient in determining the observed structures in the universe. The fundamental constants, like neutron-proton mass difference and even “gravitational constant” is found to be related to the “cosmological constant”. The addition of a “cosmological constant” in relativistic cosmology gives more mild variation of the “gravitational constant”,
compared to that found by Bambi et al.[5],
. The effect of variation of the fundamental constants on BBN is yet to be explored in full vigor. Through this work we want to suggest the possibility of non-standard Big Bang Nucleosynthesis.
References
[1] | P.A.M.Dirac; Nature, 139, 323 (1937). |
[2] | S.Kalita, H.L.Duorah and K.Duorah; Int.J.Mod.Phys.A, 26, 331-339, (2011). |
[3] | P.J.E.Peebles and B.Ratra; astro-ph/0207347, (2002). |
[4] | S.Perlmutter; Physics Today, (2003). |
[5] | C.Bambi, M.Giannotti and F.L.Villante; Phys.Rev.D., 71, 123524, (2005). |
[6] | Suyu et al.; Astrophy.J., 711, 201, (2010) |
[7] | Suyu et al. ;Astrophys.J., 277, 277, (2009) |
[8] | J.Melendez and I. Ramirez; Astrophys.J., 615, L33-L66, (2004) |
[9] | A.J.Korn et al.; Nature, 442, 657, (2006) |