Mwila Isabel Nkandu, Halil Zafer Alibaba
Department of Architecture, Eastern Mediterranean University, Mersin, Northern Cyprus
Correspondence to: Mwila Isabel Nkandu, Department of Architecture, Eastern Mediterranean University, Mersin, Northern Cyprus.
Email: |  |
Copyright © 2018 Scientific & Academic Publishing. All Rights Reserved.
This work is licensed under the Creative Commons Attribution International License (CC BY).
http://creativecommons.org/licenses/by/4.0/

Abstract
Nature has spent billions of years solving and refining many of the problems we as humans are facing today and so it is only logical for us to learn from nature’s existing solutions to solve our issues of sustainable design. Although there are many studies and approaches to designing more sustainable buildings, there is limited research on ecologically sustainable design approaches that can alleviate waste of resources by understanding the adaptation methods in natural systems. This research aims to examine biomimicry in architecture as a potential solution to sustainable building design. It analyses the basic principles and advances in biomimicry in architectural design and discusses five case studies to study how biomimicry has so far been applied in the built environment. It is expected that this research will reveal how looking to nature for inspiration can contribute to more sustainable building design by considering the structural efficiency, water efficiency, thermal environment and energy supply.
Keywords:
Ecological sustainability, Nature –inspired, Architecture, Zero- waste systems, Regenerative design
Cite this paper: Mwila Isabel Nkandu, Halil Zafer Alibaba, Biomimicry as an Alternative Approach to Sustainability, Architecture Research, Vol. 8 No. 1, 2018, pp. 1-11. doi: 10.5923/j.arch.20180801.01.
1. Introduction
After over three billion years of research, nature has already evolved and solved many of its problems. Animals, plants and other organisms have engineered themselves to survive and thrive this far without producing any waste and being very efficient with resources. Therefore, mimicking nature’s forms, systems and processes offers an opportunity to maximise resource efficiency while mitigating the negative impact of buildings on the environment (Benyus. 1997).Biomimicry is the emulation or imitation of nature in its many forms, systems and processes to solve the most pressing challenges faced by our world today. Biomimicry methods have so far proven to optimize in terms of sustainability and efficiency particularly in the fields of design and construction. However, this increasingly prominent approach has also generated development in other fields as diverse as aerodynamics, robotic navigation, medicine, clothing design and the detection of water pollution (Michael Pawlyn, 2011).Early examples of biomimicry can be seen in Leonardo Da Vinci’s sketches of a flying machine inspired by the wings of a bat. Another example comes from Fillipo Brunelleschi who studied the strength of eggshells and designed a thinner, lighter dome for the cathedral in Florence in 1436. In 1809, Naval architect Sir George Cayley designed ship hulls more streamlined by studying dolphins. A more famous example occurred in 1948 when George De Mestral, a Swiss engineer took his dog out hunting and it emerged from the bushes covered in burrs. After examining the tiny hooks of the burrs, he discovered a hook system used by the plant to spread seeds by attachment inspired by this, De Mestral created Velcro. Throughout history, architects have mainly taken inspiration from nature solely for building forms and aesthetics. Biomimicry in architecture, however, is an applied science that procures not only the aesthetics component of nature but also takes lessons from nature to solve issues in the building functionality. A multi-disciplinary approach follows a set of ethics rather than taking a stylistic approach. Sustainability is advancing to a new level that accommodates the design of buildings that are essential to the natural environment and should support nature’s work rather than work against it. It has gained a lot of popularity in the last 10 years to solve issues of sustainability while minimizing the negative impact on nature (Pedersen. 2005).There are three objectives, according to Head (2008) to reaching the so-called “Ecological Age” by the year 2050, these include; “CO2 emission reduction by 80%, ecological footprint reduction to 1.44ga/person and to increase human development index improvement.” There is a responsibility on architects to develop optimal ecological methods for design, construction and performance. This involves integrating natural ecological systems into their designs keeping in mind the human behaviour patterns.This study will take a critical look at biomimicry in architecture. It is an investigation into its key principles and concepts. It is a framework for understanding the approaches and levels of biomimicry in design. It discusses some distinct advantages and disadvantages immanent in each biomimicry level as an approach to sustainable building design. The research will look at five existing projects in which the designers looked to nature for inspiration to solve issues faced in their design process to provide insight on the level of biomimicry designers have developed. The aim of this research is to shed light on biomimicry as an approach; to showcase how it can help better the issue of sustainability and regenerative design in architecture. According to an examination conducted by M. Pedersen Zari at Victoria University in New Zealand in 2007, two distinct approaches to biomimicry as a design approach exist: Problem-Based Approach and Solution-Based Approach. These approaches each have their own advantages, disadvantages and outcomes in terms of overall sustainability. This Problem-Based approach was found to have different naming in various literatures such as “Design looking to biology” (Pedersen Zari, 2007), “Problem Driven Biologically Inspired Design” (Michael Helms, Swaroop S. Vattam and Ashok K. Goel, 2009) and “Top-down Approach” (Jean Knippers, 2009) all having the same meaning. In this approach, designers look to nature for solutions. Where a designer recognises their design problem and looks to how organisms and systems in nature have solved similar problems. One possible drawback of this design approach is that the issue of how buildings correlate with each other and the ecosystem they are part of is not investigated. Therefore, the underlying causes of non-sustainable or even degenerative built environment are not necessarily addressed. Despite this, the Problem-Based approach may be a good way to begin the transition of the built environment from inefficient to a more sustainable environment (McDonough. 2002).The Solution-Based approach is also referred to as “Biology influencing design”, “Bottom-Up Approach” or “Solution-Driven Biologically Inspired Design”. In this approach, biological knowledge influences human design. One advantages of this approach is that the knowledge of biology may influence the design in ways other than the predetermined design problem. One disadvantage is that an in-depth biological research must be conducted then the information gathered must be determined as relevant in a design context. (Pedersen Zari, M. 2007)After examination carried out by Janine Benyus in her 1997 book, it is apparent that the approaches discussed above further divide into three levels of mimicry, these are; form (Organism), process (Behaviour) and eco-system. These levels help define the kinds of biomimicry that have evolved. They provide a framework for designers who wish to employ biomimicry methods to improve the sustainability for the built environment to identify which approach to take. This will help designers determine which aspect of “bio” to “mimic” (Pedersen Zari, M. 2007). The organism level entails the designer looking at the form of a specific organism analysing how it functions; the designer can choose to mimic a part or the organism as a whole. The behaviour level, involves the imitation of how an organism interacts with its immediate environment in order to build a structure that can fit in without resistance in its surrounding environment. The third level, involves mimicking of how an organism interacts with the environment and how many components work together; this tends to be on the urban scale or a larger project with multiple elements rather than a solitary structure (Biomimicry guild, 2007).Table 1. A Framework for the Application of Biomimicry (adapted from Pedersen Zari, 2007) 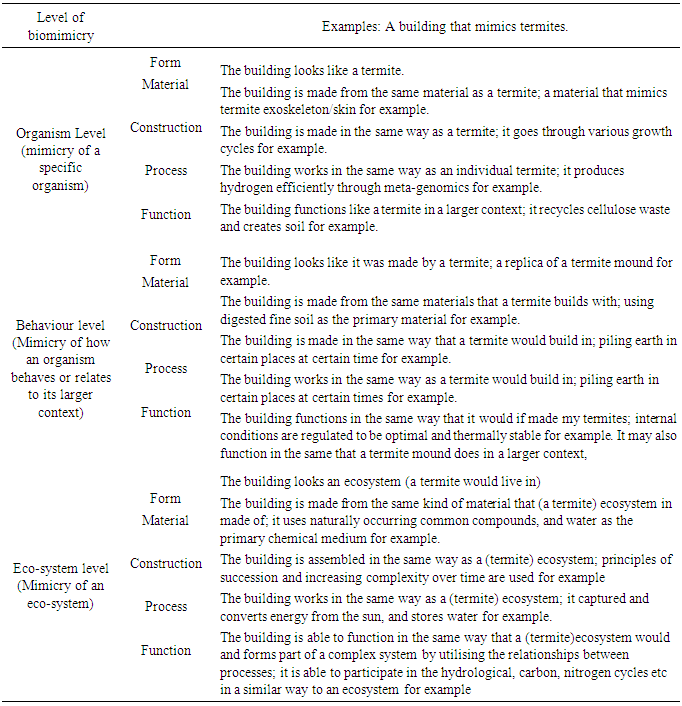 |
| |
|
2. Literature Review
The term ‘Biomimicry’ was first coined in 1962, but has just recently gained popularity. ‘Biomimicry’ comes from the Greek word bios, meaning life, and mimesis, meaning to imitate. One of the first written descriptions of Biomimicry came from Janine Benyus in 1997; she describes Biomimicry as “The conscious emulation of nature’s genius” (Benyus J.M. 1997), while for Pawlyn it is “Mimicking the functional basis of biological forms, processes and systems to produce sustainable solutions” (Pawlyn M., 2011). Many believe a biomimicry approach can not only drastically reduce the CO2 emission but save on cost. “It is possible to cut carbon emissions and save money.” says Michael Pawlyn. “The key to it is innovation.” There are characteristics of the way buildings is designed, produced and viewed and it is our job as architects to ensure the coherence of the buildings we design with its users and the environments it is in. We cannot alienate ourselves from the immediate surrounding we are building in. “The most irrevocable of these laws says that a species cannot occupy a niche that appropriates all resources- there has to be some sharing. Any species that ignores this law winds up destroying its community to support its own expansion” (Benyus J.M. 1997).In her book published in 1997 “Biomimicry: Innovation Inspired by Nature”, Janine Benyus formulated a set of questions that can be used to establish the level of biomimicry our designs (Bob & McLennan. 2004). When face with a problem in the process of designing something we must ask ourselves “Is there a precedent for this in nature?” if so then the answer to the following questions must be ‘Yes’; Does it run on sunlight? Does it use only the energy it needs? Does it fit form to function? Does it recycle everything? Does it reward cooperation? Does it rely on diversity? Does it utilize local expertise? Does it curb excess from within? Does it tap the power of limits? Is it Beautiful? McDonough and Braungart believe that biomimicry as a design approaches presents a solution for a more ecological, sustainable and regenerative built environment.“From my designer’s perspective, I ask: Why can’t I design a building like a tree? A building that makes oxygen, fixes nitrogen, sequesters carbon, distils water, builds soil, accrues solar energy as fuel, makes complex sugars and food, creates microclimates, changes colours with the seasons and self-replicates. This is using nature as a model and a mentor, not as an inconvenience. It’s a delightful prospect…” (McDonough and Braungart, 1998).According to Baumeister (2014), it is only when all three levels of biomimicry (Organism, Behaviour and eco-system) have been taken into account will the design ‘behave like a well-adapted organism’ and ‘create conditions conducive to life.
3. Methodology
The type of research used in this study comprises of examination of the latest researches relating to Biomimicry in architecture. The study investigates the how and why various designers applied Biomimicry methods into their projects. It will look at the three levels of Biomimicry and conduct case studies under each level. Of the five projects that were analysed, one represents the Organism level, one represents the Behavioural level and three represent the Eco-system level. The projects are as listed below: Organism level: 30 St Mary Axe A.K.A Gherkin Tower, United Kingdom Behavioural level: East gate mall, Zimbabwe. Eco-system Level: Cardboard to caviar project, United Kingdom, Sahara forest project, Qatar, Jordan and Tunisia and the Eden project, United KingdomOverall, these five case studies have been carefully chosen and thoroughly analysed in order to understand the way in which biomimicry is integrated effectively into the design project. They were selected because they successfully represent the way in which 21st century architects and designers are taking inspiration from nature and applying in into their design producing design that are much more efficient and ecologically sustainable than most design projects today. Close attention has been paid to the eco system level because it holds the most potential to produce buildings that are not only highly sustainable but also regenerative. Doing so will show how biomimicry is and has been applied to architect and provide an insight on the possible ways to expand and delve deeper into the design approach.Given the fact that Biomimicry in architecture is still an emerging discipline, many designers are still trying to understand and find ways to apply this approach in their design and so there are very few examples that implemented it at a grand scale. Therefore, these projects have been mindfully chosen as they represent the current general way designers have integrated of biomimicry in architecture theoretically and practically to show the potential impact biomimicry can have as an ecological sustainable design practice in a wider context.
4. Findings
4.1. Organism Level
The organism level approach may help to understand the negative environmental impact that human activities have on the worlds and its many of its ecosystems. For billions of years organisms have withstood and adapted to many changes over time. Humans therefore, have a wide variety of examples to study and draw solutions for problems that have already been addressed, particularly in the use of energy and materials effectively. As Baumeister (2007) points out, “The research has already been done”. (Alberti et al. 2003)A disadvantage, however of mimicking an organism is that without mimicking how it interacts and contributes to the ecosystem at larger context, it has the potential to produce designs that are below average in terms of the impact it will have on the environment. (Reap et al. 2005)The case study being conducted under the organism level is the 30 St Mary Axe.
4.1.1. 30 St Mary Axe A.K.A Gherkin Tower (2003)
This building takes inspiration from the Venus Flower Basket Sponge (see Figure 1 below). This sponge sits in an underwater environment with strong water currents and its lattice-like exoskeleton and round shape help disperse those stresses in various directions and its round shape reduce forces due to strong water currents.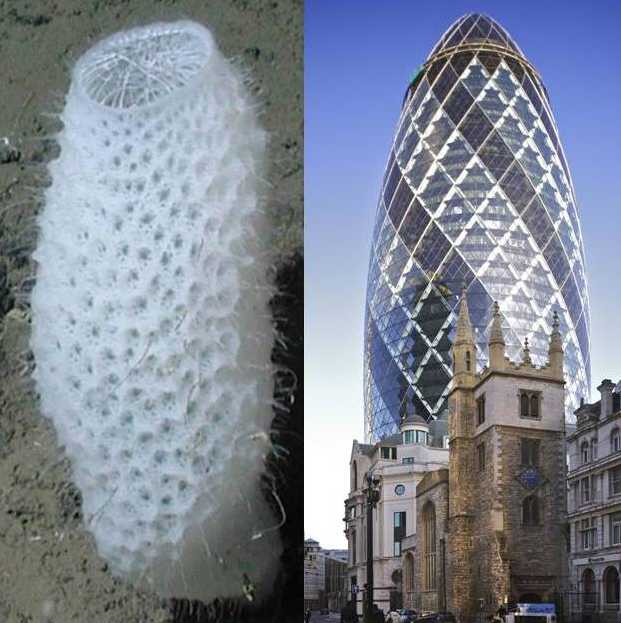 | Figure 1. Venus Basket sponge (left) Gherkin tower (right) |
The curved sides allow winds to pass easily around the building, rather than deflecting down to street level to blast pedestrians. Because more air can flow around the side of a cylinder than the corner of a rectangle, its speed increases, causing a higher negative air potential at the back of the building. Architects Norman Foster and Sons used this to drive a natural ventilation system.Inspired by the hexagonal skin of the Venus flower sponge, the main structure of the building is aluminium coated steel diagrid structure. Yoram Eilon, vice president of engineering firm WSP Cantor Seinuk, describes the Diagrid structure as “a series of triangles that combine gravity and lateral support into one, making the building to be stiff, efficient and lighter than a traditional high-rise”. The Diagrid divides the tower height into a series of modules this is referred to as a vertical cantilever. This freestanding structure has no columns, which allows for uninterrupted interior office space with revolving triangular atriums (see Figure 2 below). (Vikram bengani, 2015)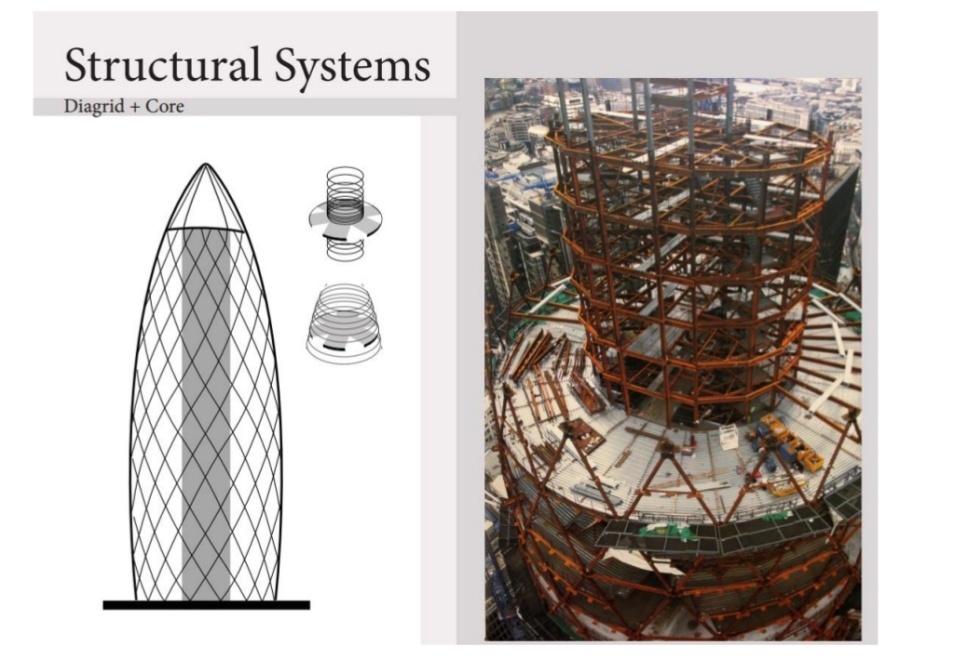 | Figure 2. Diagrid structure with core in the centre (left) and Construction of core and Diagrid structure (right) |
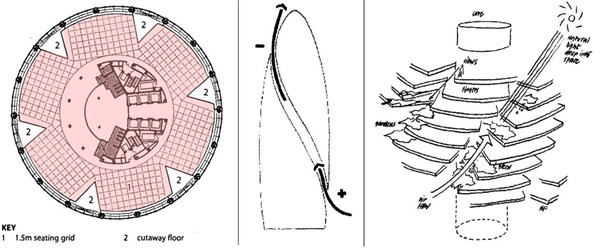 | Figure 3. Floor plan showing lightwells (left), Sketches showing movement of natural light and air through lightwells (right) |
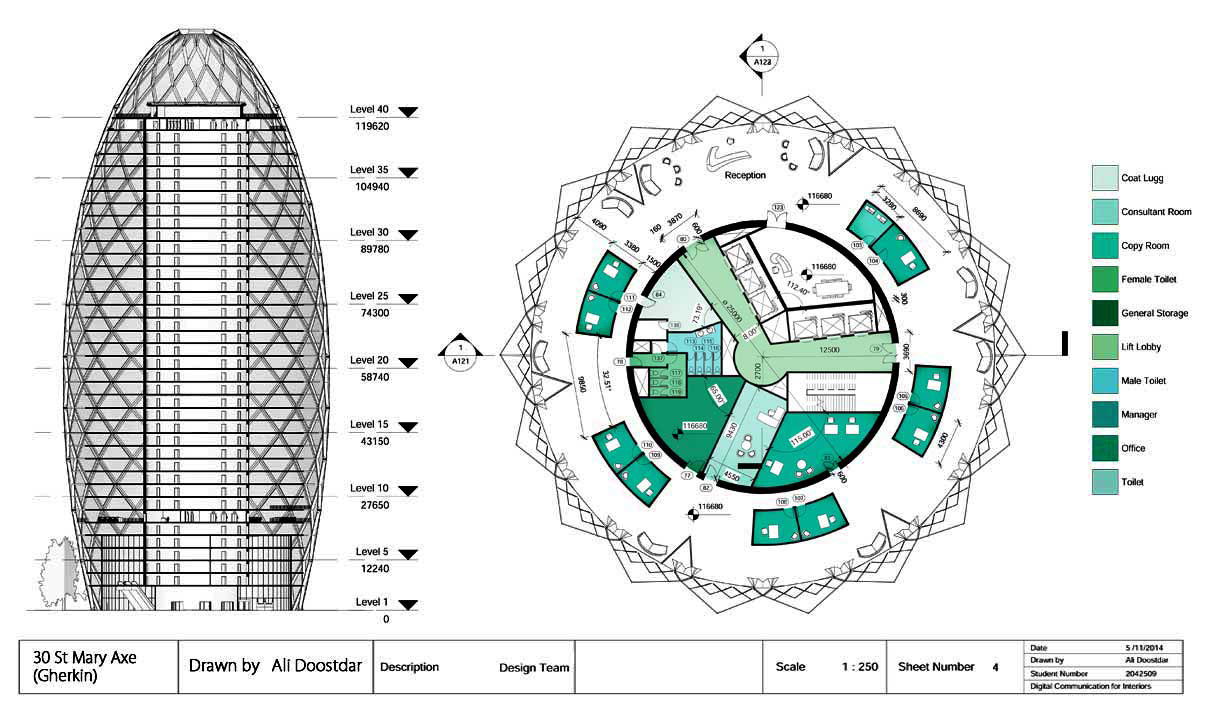 | Figure 4. Section of the building (Left) and typical floor layout (Right) |
The building has a 5-degree rotation between each floor plate to incorporate wedge shaped lightwells. These lightwells allow light and air to reach each floor of the building. (See Figure 3 above)A double skin façade was selected in order to maximize sun exposure. The outer skin of the building is composed of mullions and triangular shaped windows, while the inner wall is made of sliding glass doors made accessible only to maintenance. In between the two walls is a space with a row of horizontal shading devices. These double walls also contain venting flaps to allow hot air to travel up and out of the building (Sahil Virmani, 2014). The design of this tower presents some advantages that make it very effective. Firstly, the building has minimal windblast of pedestrians at street level, due to the cylindrical shape of the tower, which allows wind to pass smoothly around it. Another advantage of the is the efficacious implementation of passive cooling , heating, ventilating and lighting techniques through the use of double skin façade. In addition, the use of rotated floor slabs produce lightwells, which provide each floor with natural day lighting and ventilation. (Vikram bengani. 2015)However, this design does pose some disadvantages and threats. A massive building such as the Gherkin Tower made of glass gives rise to a few problems that presents a danger to its users, as was the case when one of the numerous glass panels fell off in 2005 when a window fell from the 28th floor to the plaza below. Another drawback in the design of glass skyscrapers is the sun glare from the glass. This creates discomfort for the pedestrians and drivers below and can result in accidents as the strong glare may disrupt your vision. Despite this, the gherkin tower does prove more efficient than most buildings its size, credit can be given to the biomimicry design methods used by the designers. (Martin Wainwright, 2005).
4.2. Behavioural Level
The behavioural level is based on the fact that a vast number of organisms have learnt to operate within the capacity of specific environmental conditions and within the limits of energy and material availability, they encounter the same environmental conditions that humans do and therefore need to solve similar issues that humans face. These limits as well as pressures that create ecological niche adaptations in ecosystems mean not only well-adapted organisms continue to evolve, but all well-adapted organism behaviours and relationship patterns between organisms or species (Reap et al. 2005).
4.2.1. The East gate Centre, Harare, Zimbabwe
Indigenous Zimbabwean masonry and the self-cooling mounds of African termites inspired the East gate centre design; it stays regulated year round with dramatically less energy consumption using design methods. Zimbabwe has a temperate climate because of its altitude with temperatures ranging from 7-27 Degrees Celsius this makes passive heating and cooling a possible alternative to mechanical methods. This mid-rise building designed by architect Mick Pearce in collaboration with Arup engineers, has no conventional air-conditioning or heating. It uses less than 10% of the energy of a conventional building its size through passive cooling and heating techniques (Michael Pawlyn, 2011).Termites constantly open and close a series of heating and cooling vents in the mounds throughout the course of the day; this keeps the building temperature regulated all day (See Figure 5 below). The East gate building operates in the similar way; outside air is drawn in through vertical ducts on the first floor and is either warmed or cooled by the building mass depending on which is hotter the building concrete or the air. It is then pushed into the building’s floors through the central spine of the two buildings before exiting via chimneys at the top. (Abigail Doan.2012) (See Figure. 6 below). 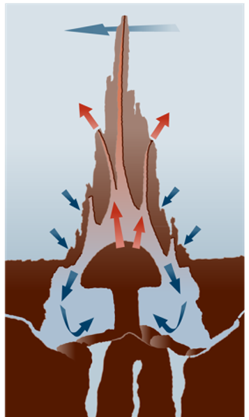 | Figure 5. Showing air circulation in a typical termite mound |
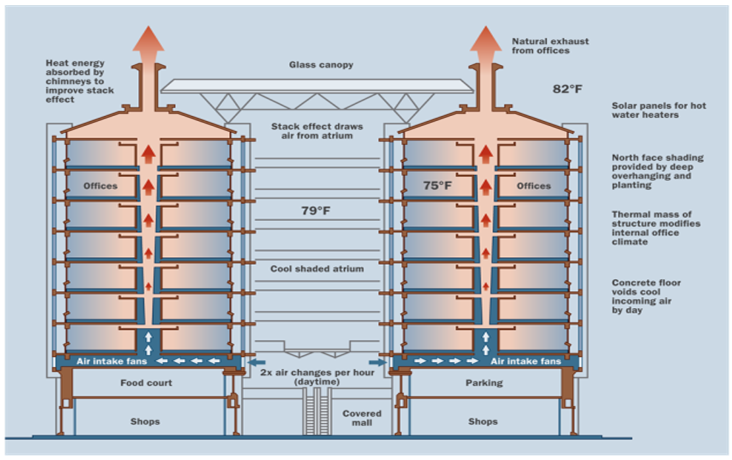 | Figure 6. Air circulation in East gate buildings |
There are two buildings are joined together by steel bridges this makes the building open to the local breezes. The bridges are suspended on cables from steel lattice beams, these bridges span over the atrium below. On top of the bridges lies a glass roof. Both buildings have protruding stone elements to protect the windows from the surface area of the building façade to improve heat loss by night and minimize heat gain by day. Below is a study conducted in 1998 of the way the East gate building successfully regulates the temperatures of the internal area of the building, the outside air and of the concrete slab (Figure 7) (Michael Pawlyn, 2011).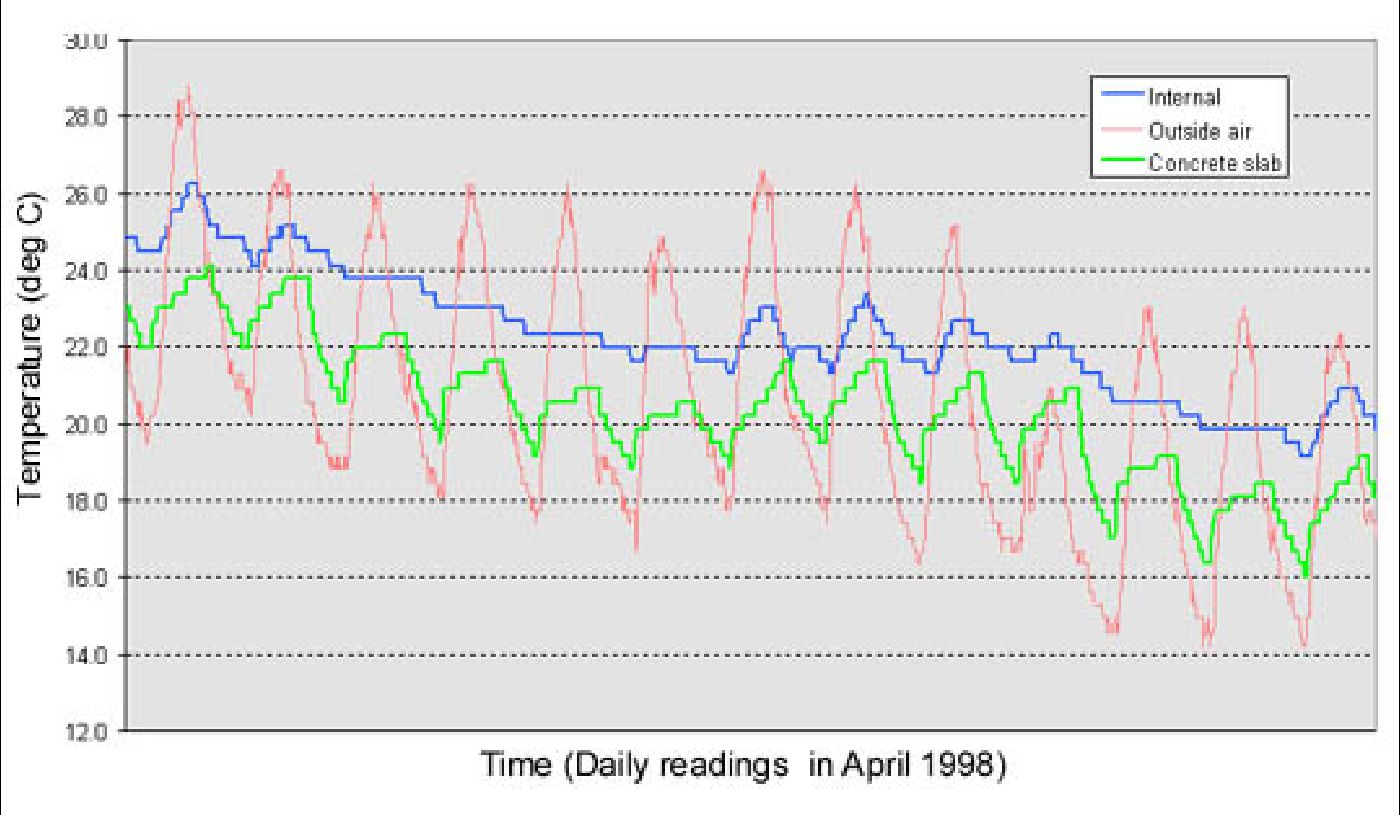 | Figure 7. Thermal regulation of the concrete slab. In East gate mall |
After a detailed simulation, the engineering company (Arup engineers) gave Pearce a set of rules to follow in his design: The external walls on the North façade must not have any direct sunlight falling on them. The window to wall ratio are must not exceed 25%. Windows must be light filter, controlling glare, noise and security. Because of noise pollution and unpredictable wind pressures and temperatures, windows must be sealed with ventilation relying solely on the duct system. (Shiva Shankar. 2016)
4.3. Eco-System Level
Benyus (1997) and Vincent (2007) describe the mimicking of ecosystems as an integral part of biomimicry. An advantage of mimicking at this level of biomimicry is that it can be used in conjunction with other levels of biomimicry (organism and behaviour). The most important advantage of such an approach to biomimetic design however may be the potential positive effects on overall environmental performance.
4.3.1. The Cardboard to Caviar Project in Wakefield UK
The cardboard to Caviar project (also known as the “ABLE project”) is an example of a closed loop system in which no waste is produced and a much greater productivity is yielded. Graham Wiles initiated this project, at every stage of the project; he applied the biomimetic principle of using waste as a resource to feed another process. The project begins with collecting empty cardboard boxes from restaurants and using them as horse bedding in the stables. The soiled cardboard is then collected and used to establish a wormery composting system. With the abundance of bait, Wiles decided to establish a small-scale Siberian sturgeon fishery. The sturgeon then produces caviar, which is sold back to the restaurants. This demonstrating the possibility to turn what would be considered a waste material into a high value product while also generating numerous social, economic and environmental benefits (Michael Pawlyn, 2011).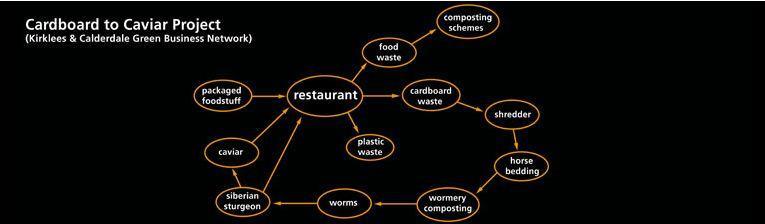 | Figure 8. Cardboard to Caviar project |
4.3.2. Sahara Forest Project Qatar, Tunisia and Jordan
A greenhouse project that took inspiration from the Namibian fog-basking beetle, which has found its own way to evolve its own fresh water in a desert and to regulate its body temperature by accumulating heat by day and collecting the water droplets formed on its wings by the fog. The greenhouse design emulates this beetle to combat climate change in an arid climate. (Michael Pawlyn 2011).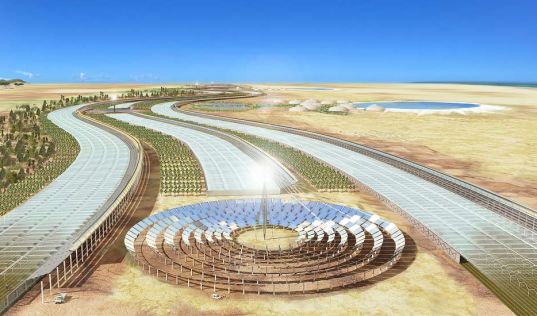 | Figure 9. Sahara Forest project Tunisia |
The Exploration Architecture design team, strived to design an eco-system that was not only sustainable but also regenerative. Three core components drove the design of the greenhouse; the major one being a creating a saltwater cooled greenhouse, concentrated solar power (CSP) and technologies for desert vegetation. (An Oasis of Green Building. n.d)Saltwater cooled greenhouses present suitable conditions for year round cultivation. To create a saltwater cooled greenhouse Charlie Paton created system to provide evaporative cooling and humidification in the greenhouse structure using saltwater. The evaporated air condenses to fresh water allowing the greenhouse to remain heated at night. This system produces more water than the interior plants need so the excess is spewed out for the surrounding plants to grow.The salt extracted from the evaporation process are crystallised at different stages to extract various elements. The first things to crystallise out the evaporated saltwater is calcium carbonate followed by sodium chlorite; these elements can both be compressed into building blocks. Many other elements present in seawater are reused in other ways to minimize the waste (Michael Pawlyn 2011).Concentrated solar power involves concentrating the heat from the sun with solar tracking mirrors to create steam that drives conventional turbines; this produces zero carbon electricity, which is twice as effective as photovoltaics (Biomimicry and the Sahara Forest Project. n.d).This project offers a solution to restoring forests and creating a solution for vegetation of deserts as a source of food, water and energy in an otherwise resource –constrained area of the world.“…the Sahara Forest project is a model for how we can create zero carbon food, abundant renewable energy in some of the most water stressed parts of the of the planet as well as reversing desertification in certain areas” (Michael Pawlyn 2011).
4.3.3. The Eden Project (2001) in Cornwall, England
Grimshaw architects designed this project, they are a company in which Michael Pawlyn practiced and was a core part of the architectural team that designed the Eden project in the year 2000. Grimshaw Architects looked to nature to build an effective spherical shape. It has two huge artificial enclosures; each enclosure emulates a natural biome. Soap bubbles inspired the forms of the biomes and cellular structures inspired the hexagonal frames. A biome is a natural occurring community of flora occupying a major habitat. The artificial biomes in the Eden project feature a humid tropic rainforest and Mediterranean biome. (Michael Pawlyn, 2011)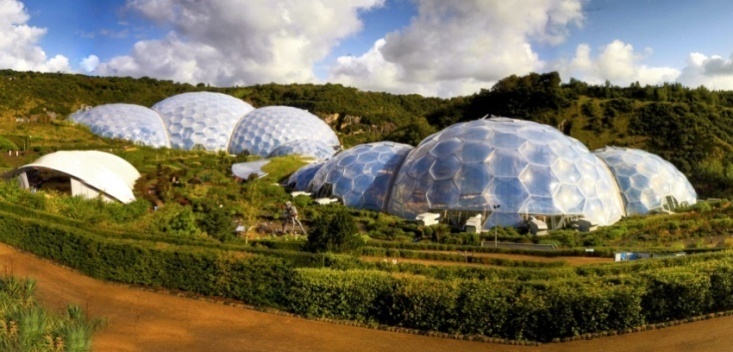 | Figure 10. Eden Project |
The Humid Tropics Biome recreates the natural environment of a tropical rainforest. The warm, humid enclosure is home to hundreds of trees and other plants from rainforests in South America, Africa, Asia and Australia such as fruiting banana plants, coffee, rubber and giant bamboo; it is kept at a tropical temperature and moisture level. The dome is 240 metres long, 55 metres high and measures 110 metres across at its widest point. (Michael Pawlyn, 2015)The Mediterranean biome has varied plant life from temperate rainforests in Southern Africa, the Mediterranean and California. The Mediterranean biome covers (1.6 acres) and measures 35 metres high, 65 metres wide, and 135 metres long and houses familiar warm temperate and arid plants such as olives and grape vines and various sculptures (Michael Pawlyn, 2015).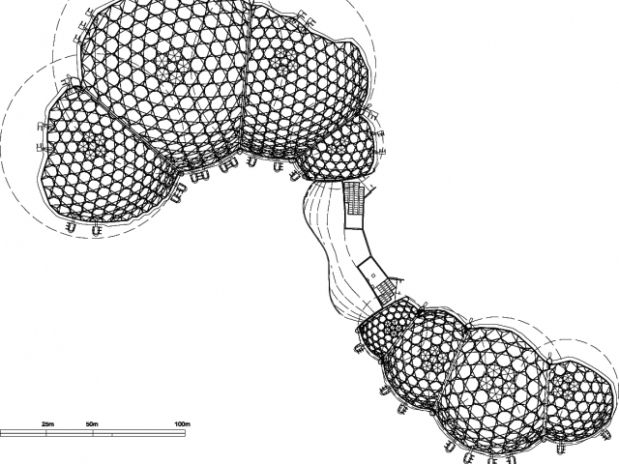 | Figure 11. The Eden project |
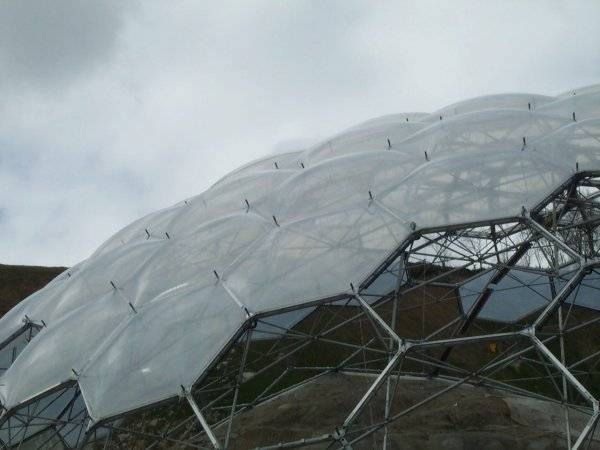 | Figure 12. ETFE pillows |
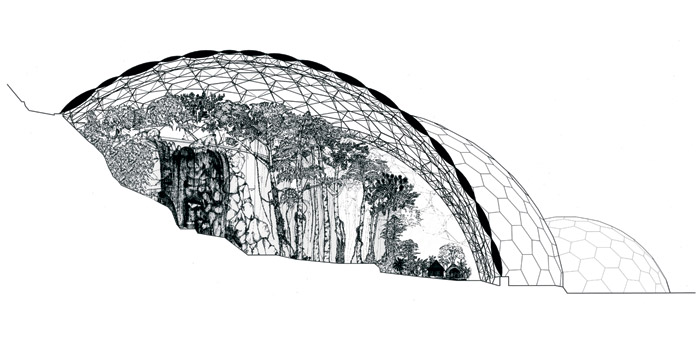 | Figure 13. Cross section of Eden project |
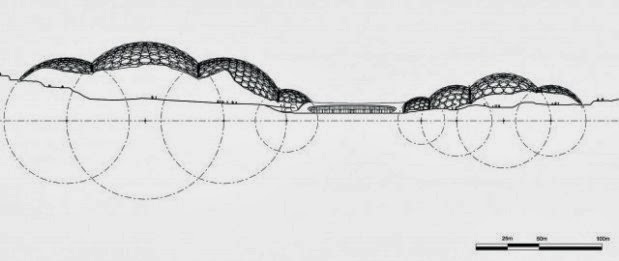 | Figure 14. Schematic site section |
Each of the biomes consists of several geodesic domes joined together. These domes are made of hexagonal pillows, made from three layers of a material called Ethylene Tetrafluoroethylene (ETFE) welded together along the sides and on top of one another. These layers have air pumped into them this increases the level of insulation without affecting the amount of sunlight shining through the material. The amount of air in between the layers is adjustable, during the winter, the pillows are pumped with more air to increase the amount of insulation and they are partially deflated during the summer to allow more cooling in the space. The pillows are built to detach easily from the steel frame, so they can be replaced should a more efficient material come along. One advantage of the geodesic dome shape is that it adapts easily to most ground surfaces. (Murray-White, 2010)The use of inflated Ethylene Tetrafluoroethylene (ETFE), a material that is both light and strong, (1% of the weight of double glazing) provides other benefits such as a lighter steel frame, letting in more sunlight and adding solar gain also and it costs 1/3 less than the traditional glass solution. The final superstructure weighs less than the air it contains. (Michael Pawlyn, 2015.
5. Discussions
This study looked at how biomimicry fits into sustainable design practices in order to provide efficient ecological designs with moderate use of materials and energy at a macro scale. It also looked at how biomimicry is currently applied in theory and in practice specifically in the field of architecture. It discusses the two different approaches to biomimicry in architecture and presents some advantages and disadvantages of each approach. Biomimicry as an architectural design method presents a suitable solution to the current sustainability predicament without affecting the environment negatively. The five case studies conducted in this research, reveal that biomimicry aids in resource efficiency. It has great potential to pave the way in the production of ecological sustainable designs, zero waste systems and an overall regenerative built environment thereby not only positively affecting human life as a whole but also working with nature instead of against it.An effective biomimetic approach to architectural design requires the development of design methods that take into consideration the modelling of behaviour, the constraints of materials and the influence of environmental factors. This requires an in-depth understanding of form, material and structure not separately but rather as having complex interrelations.Some critics argue that biomimicry is an indefinite and broad way to approach sustainability, stating that, to apply it effectively requires an extensive study with the help of multiple disciplines. While others criticise that the principle of biomimicry separates man from nature without acknowledging his eminent role in the eco system. The concept of biomimicry is still in the developing phase and is not largely applied as a design method. To execute it successfully at a grand scale requires the co-operation of multiple disciplines such as biologists, ecologists and designers who can establish the relationships between the organism and systems in nature and the needs of humans in order for them to make ethical decisions for a more sustainable built environment.
6. Conclusions
The built environment accounts for a majority of the world’s global environmental and social problems with vast proportions of waste, material, energy use and greenhouse gas emissions (Mazria, 2003, Doughty and Hammond, 2004). There is a rapidly growing demand for an effective ecological sustainable design approach without compromising the needs of the society. Although there are currently numerous approaches to sustainable design in architecture, very few have proven effective at a macro scale. Biomimicry offers a relatively new solution to our issues of sustainability. It demands the integration of multiple disciplines working together to produce buildings and systems that are not only more beneficial to its users but also give back to nature. Once implemented well, imitating could prove advantageous in the field of architecture and human life as a whole. The greatest limitation of this study is that although many architects are interested in taking inspiration from nature, a widespread application of biomimicry as a design method remains largely unrealised because of this there are a very small number of existing projects that have truly integrated biomimicry at a grand scale in theory and in practice. This is because biomimicry as a sustainability approach is very much still an emerging discipline in the development phase. Nevertheless, there is an obvious positive attitude towards biomimicry and this shows a potential for the increased integration of this discipline in architectural design. As there is a growing need for buildings that work with nature to create a regenerative built environment, architects can no longer ignore the relevance of bio-inspired theories and approaches to achieve a more sustainable future. By using the framework in this paper, a clearer distinction is seen between the different levels of biomimicry and that they all present different potentials.
References
[1] | Abigail Doan. 2012. Biomimetic architecture: Green Building in Zimbabwe Modelled after Termite Mounds. Retrieved from: https://inhabitat.com/building-modelled-on-termites-eastgate-centre-in-zimbabwe/. |
[2] | Alberti, M., Marzluff, J. M., Shulenberger, E., Bradley, G., Ryan, C., & Zumbrunnen, C. (2003). Integrating Humans into Ecology. Bioscience, 53, 1169-1179. |
[3] | An Oasis of Green Building. Retrieved from https://www.saharaforestproject.com/qatar/. |
[4] | Baumeister, D. (2007). Evolution of the Life's Principles Butterfly Diagram. In M. P. Zari, Biomimetic Approaches to Architectural Design for Increased Sustainability. [Personal Communication]. |
[5] | Baumeister, D. (2014). Biomimicry Resource Handbook - A seedbank of best practice. |
[6] | Benyus J.M. (1997). Biomimicry Innovation inspired by nature. New York. Marrow. |
[7] | Berkebile, B McLennan, J. (2004). The Living Building; Biomimicry in Architecture, and Integrating Technology with Nature. |
[8] | Biomimicry Inspired by Nature Guild (2007). Innovation Work Book, Biomimicry Guild. |
[9] | Biomimicry and the Sahara Forest Project. (n.d). Retrieved from https://www.saharaforestproject.com/biomimicry/qatar. |
[10] | Eastgate. (n.d). Retrieved from: http://www.mickpearce.com/Eastgate.html. |
[11] | Gamage, A. (2015). Exploring a Biomimicry Approach to Enhance Ecological Sustainability in Architecture. |
[12] | Helms, M., Swaroop, S. V., & Goel, A. K. (2009). Biologically inspired design: process and products. Elsevier, 606-622. |
[13] | Knippers, J. (2009). Building & Construction as a Potential Field for the Application of Modern Biomimetic Principles. International Biona Symposium. Stuttgart: [Personal communication]. |
[14] | Martin wainwright, 2005. Gherkin skyscraper sheds a window from 28th storey. |
[15] | Gherkin skyscraper sheds a window from 28th storey. Retrieved from: https://www.theguardian.com/society/2005/apr/26/urbandesign.arts. |
[16] | McDonough, W., & Braungart, M. (2002). Cradle to Cradle-Remaking the Way We Make Things. New York: North Point Press. |
[17] | Green Architect, Designer of the Eden Project: A Case Study. (2010). Retrieve from http://www.sustainablebuild.co.uk/designer-eden-project-case-study.html. |
[18] | Pawlyn. M. (2011). Biomimicry in Architecture. London. UK: RIBA Publishing. |
[19] | Pawlyn. M. (2015). Using nature's genius in architecture. Retrieved from: https://www.ted.com/playlists/28/sustainability_by_design. |
[20] | Sahara Desert Project to grow 10 hectares of food in Tunisian desert (n.d). Retrieved from https://inhabitat.com/sahara-desert-project-to-grow-10-hectares-of-food-in-tunisian-desert/. |
[21] | Salma Ashraf El Ahmar, “Biomimicry as a Tool for Sustainable Architectural Design: Towards Morphogenetic Architecture” (master’s thesis, Alexandria University, 2011), 22. |
[22] | Sahil Virmani. (2014). Biomimicry. Retrieved from: https://issuu.com/sahilvirmani07/docs/bio. |
[23] | Vikram bengani. (2015). the gherkin: Case study .Retrieved from: https://www.slideshare.net/VikramBengani/the-gherkin-case-study. |
[24] | What is biomimicry? (n.d). Retrieved from https://biomimicry.org/. |
[25] | Zari, M. P. (2007). An ecosystem based biomimetic theory for a regenerative built environment. Sustainable Building Conference. Lisbon: [Personal communication]. |