Karlybaeva B. P.1, Berdimbetova G. E.1, Shmanay V. V.2, Kovalskaya S. S.2
1Karakalpak Scientific Research Institute of Natural Sciences of the Karakalpak Branch of the Academy of Sciences of the Republic of Uzbekistan, Nukus
2Institute of Physical and Organic Chemistry of the National Academy of Sciences of Belarus
Copyright © 2020 The Author(s). Published by Scientific & Academic Publishing.
This work is licensed under the Creative Commons Attribution International License (CC BY).
http://creativecommons.org/licenses/by/4.0/

Abstract
A technique for obtaining chitosan with a high degree of deacetylationhas been developed based on alkaline hydrolysis of chitin isolated from cysts Artemia Parthenogenetica crustaceans. The methods of synthesis of amphiphilic chitosan derivatives based on partial acylation or alkylation of the amino group of biopolymer have been proposed. The obtained N-acetyl- and N-oleoylchitosanscan be used in biochemical studies, in particular, for obtaining complexes with DNA.
Keywords:
Cysts, Chitin, Chitosan, Molecularweight, IR and NMR spectra, Alkylation, Acylation, Amphiphilic derivatives, N-oleoylchitosan, N-cetylchitosan
Cite this paper: Karlybaeva B. P., Berdimbetova G. E., Shmanay V. V., Kovalskaya S. S., Isolation of Chitin/Chitosan from Artemiaparthenogenetica Crustacean Cysts and Preparation of Its Hydrophobically Modified Derivatives, American Journal of Polymer Science, Vol. 10 No. 1, 2020, pp. 1-9. doi: 10.5923/j.ajps.20201001.01.
1. Introduction
Chitin is a natural polymer that forms the basis of the exoskeleton of insects and crustaceans. Processing of a class of chitin mucopolysaccharides, a number of types of their administration (heparin, chondroitin sulfate, hyaluronic acid) are common. It also forms the basis of operations in agriculture, medicine, and biopharmaceuticals. However, chitin is also used to some extent in industrial production processes.Chitin - Chitin, the second largest biopolymer after cellulose, can be obtained from many sources, including shellfish shells, insects, and exoskeletons of invertebrates [7]. These substances are used in various fields of human activity. For example, agriculture, water treatment, cosmetics, biotechnology, medicine and etc and also among the various compounds used in medicine, they are: lipids, cholesterol, proteins, metal ions, radionuclides, toxins, which are determined by their ability to form complexes. The ability of chitin to excrete toxicity makes it a very convenient tool and a convenient tool for the neutralization or removal of lipids from the intestinal tract in the treatment of patients [10]. Wider use of chitin is limited by the insolubility of large amounts of organic solvents, even in water. On the contrary, its deacetylated product - chitosan - is perfectly soluble in weak acid solutions. These substances are shaping prospects for use in medicine and biochemical research. The specific properties of chitosan include high levels of hydrophilicity, toxicity, amino grouping, and modified convenience.One of the only biological organisms currently preserved in the Aral Sea is the Artemia Parthenogenetica crustacean. In other words, it is the main source of chitin and chitosan, which are needed in various fields from rare insects. It is important to obtain natural polymers from such biological organisms [9].The aims of the work are: Optimization of chitin hydrolysis technique for obtaining chitosan with high degree of deacetylation and production of methods for the synthesis of amphiphilic chitosan derivatives.
2. Materials and Methods
2.1. Separation of Chitin from Artemia parthenogenetica Crustacean Cyst
We have been conducting our scientific research in the Aral Sea since 2017 to obtain chitin. We used a variety of methods to obtain such substances, and studied the scientific work of a number of scientists in the field and their methods. In particular, we have studied the scientific works of Lazurevskiy GV, Terenteva IV, Shamshurin AA, Shipovskaya AB, Zang K., Erselen S., Tikhonov VE, Karaeva SZ, Slita AV, Zarubaev VV, Meli I., Dyuportay G. and other scientists.As a starting material, chitin is treated with 5% hydrochloric acid and left at room temperature for 2 hours. It was then filtered and washed with distilled water to a neutral pH level. It was then treated with 5% sodium hydroxide at 100–120°C for 2 h. It is again filtered and washed in a neutral medium with distilled water. The process was repeated 3 times. In this experiment, most of the organic compounds (proteins, fats) and inorganic substances were hydrolysed to a water-soluble form. Finally, the resulting chitin was washed with ethyl alcohol and chloroform to remove water-insoluble compounds and pigments [1]. The composition of the main control components in the cysts is given in Table 1.Table 1. The main components of cysts are  |
| |
|
2.2. Mass Fraction of Moisture
The mass fraction of moisture in the raw material was determined by drying the samples to a constant mass [2] for 3 hours at 100-105°C in accordance with the requirements of GOST 23637 [3].
2.3. Mass Fraction of Protein in the Raw Material
According to the Kjeldahl method (GOST 32044.1-2012) [4,5].
2.4. Determination of Ash Content of Aral Sea Artemia Cysts
The mass fraction of minerals was determined after burning organic matter in a muffle furnace at a temperature of 500–700°C for a continuous mass for 5–6 h [6]. The chitin content was controlled by elemental analysis.The patent of the Russian Federation was used as a basis for the development of a method for obtaining chitosan from the chrysanthemum of the Aral Sea artemia [7]. Because we needed chitosan, which has the highest level of deacetylation, hydrolysis was performed under more severe conditions. The components were mixed in an amount of 10 ml of 50% aqueous KOH solution for 1 g of chitin. The mixture was boiled for 4 hours, cooled to room temperature and poured into 10 times the amount of distilled water. The chitosan precipitate was filtered, washed once with distilled water, and once with acetone. Dried overnight in a vacuum desiccator (oil pump). The yield of the product was 73% (for chitin, samples containing it were calculated by the amount of nitrogen).
2.5. Determination of the Molecular Mass of Chitosan by Viscometric Method
Ubbelod viscometer with a capillary diameter of 3 mm, chitosan solutions were prepared in 4% acetic acid (50 mg chitosan in 20 ml of water) to determine viscosity. The shelf life of the solution was measured, then it was diluted in half and the shelf life was measured again [8]. Industrial chitosan (China) was used as a comparison example. The data are presented in Table 2.Table 2. Determination of the molecular mass of chitosan by viscometry 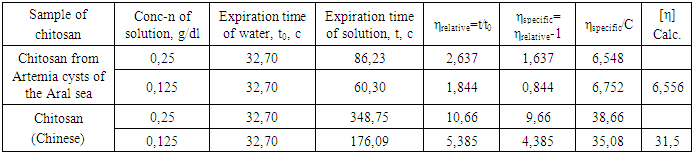 |
| |
|
The average viscosity mass Мη of chitosan samples was calculated using the Mark-Kuhn-Hauvink equation:[η]= 1,38.10-4.Мη0,85
2.6. Determination of the Critical Micelle Concentration for Chitosan Samples on the Malvern Zetasizer
The critical concentration of micelle formation for chitosan samples from the “Aral Sea Artemia” and a sample produced in China was determined using Malvern Zetasizer.For the experiment, chitosan solutions with a concentration of ~ 25 mg / ml in 4% acetic acid were prepared and the size of the micelles was measured. The solutions were then diluted with water: in 200 ll steps for 1000 ul of natural solution. The critical concentration of micelle formation (CCM) was estimated to be less than 1 nm [10].
2.7. Thecontent of Chitosan and Its Derivatives was Determined Based on the Data of IR, NMR-1H and 13C Spectroscopy
The IR spectra of the studied materials were recordedon the spectrophotometer IR Fourier spectrometer NicoletiS50 FT-IR in the area of 4000-400 cm-1. To record the spectra, tablets with KBrwere used.NMR spectra were recorded for solutions in D2O with the addition of a drop of CF3COOH.
2.8. Synthesis of N-oleoylchitosan
The compound was obtained by the method of mixed anhydrides. To perform this reaction, we experimentally selected a solvent mixture containing a minimum amount of water and at the same time well dissolving chitosan. Its content is methylene chloride - acetic acid - water in a ratio of 10:20:3. Thiscontent of mixture is well suited for dissolving mixed anhydride obtained from isobutyl chloroformate and oleic acid. Molar ratio substrate (per 1 link) - mixed anhydride ~5:4.
2.9. Dissolution of Chitosan
To a mixture of 10 ml of methylene chloride and 20 ml of glacial acetic acid was added 1.5 g of chitosan. When 3 ml of water was added, the chitosan was completely dissolved by stirring.
2.10. Obtaining Mixed Anhydride
0.192 g of oleic acid was dissolved in 20 ml of methylene chloride, 0.98 ml of triethylamine was added and cooled to -20°C. Gradually 0.78 mL of isobutyl chloride was added to maintain the low temperature. The reaction mixture was held for another 15 min, then the triethylamine hydrochloride precipitate was filtered. Rapid filtration is important because when the reaction mixture is heated, the solubility of the hydrochloride in methylene chloride increases and it becomes impossible to separate it completely [11].
2.11. Acylation of Chitosan
The mixed anhydride solution was gradually added to the chitosan solution with active stirring, and the mixture was left at room temperature for one day. The reaction mixture was then neutralized; the sediment product was filtered, washed with water, and dried in a vacuum extractor. 2.83 g of N-oleoylchitosan were obtained.
2.12. Synthesis of N-cetylchitosane
2.5 g (15.5 mmol) of chitosan was dissolved in 20 ml of 1% acetic acid; and 2 ml (8 mmol) of С16Н33 cetyl chloride was dissolved in 20 ml of chloroform. Both solutions were placed in a flask and in an interphase transfer catalyst of 43 mg (1 mol. %) Tetrabutylammonium chloride. The mixture was kept for one day with intensive stirring, the water phase was separated, and the reaction product was cultured with the addition of isopropanol. The product was washed with water and dried in vacuum desiccators.
3. Results and Discussion
The chitin we obtained was a lightly dusty mass of yellowish color. The composition of the chitin obtained is given in Table 3.Table 3. Elemental analysis of chitin  |
| |
|
From the data presented, it can be seen that the chitin obtained still contains large amounts of organic compounds. No inorganic contaminants.Chitin is insoluble in any solvent, which severely limits its practical use. On average, with a sufficiently high degree of chitin deacetylation, the resulting product - chitosan - is soluble in slightly acidic aqueous solutions, but the latter, unlike chitin, is no longer broken down by specific enzymes. The loss of biological degradation is offset by the appearance of polyelectrolyte properties in chitosan. However, there is a compositional heterogeneity of chitosan due to incomplete deacetylation of chitin: i.e., chitosan is a glucosamine polymer where the bonds vary with free (NH2) and acetylated (NHCOCH3) amino groups. It is believed that in the hydrolysis of chitin, chitosan is usually formed, with a deacetylation rate of about 85%. Using the method we proposed, we obtained chitosan with a high degree of deacetylation, which was confirmed by spectral data.The initial chitin spectrum contains bands associated with oscillations of the acetamide group at frequencies of 1658 cm-1 (amide I) and 1557 cm-1 (amide II). There are also bands of vibrations of C-O and C-O-C bonds (1260, 1029-1158 cm-1), as well as a strong broadened band in the area of 3600-3100 cm-1, corresponding to the hydrogen bonds of OH and NH groups. In the chitosan spectrum obtained by hydrolysis of chitin hydroxide, residual signals of the vibration bands of the amido groups are observed, and a strong oscillation range of the free amino group appears (1578 cm-1). The remaining bands in the spectrum vary slightly [9].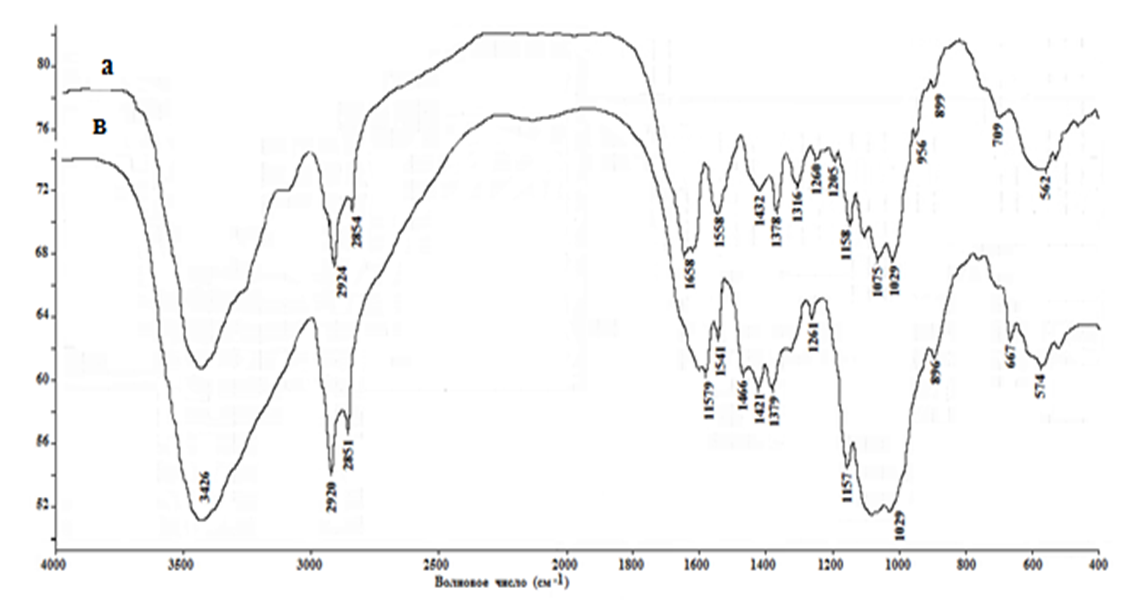 | Figure 1. IR spectrum of chitin (a) and chitosan (b) from Artemia cysts of the Aral sea |
The degree of deacetylation was quantified based on spectroscopy data NMR 1H. Thespectra were recorded for solutions in D2O with the addition of a drop of CF3COOH (chitosan is not soluble in a neutral medium).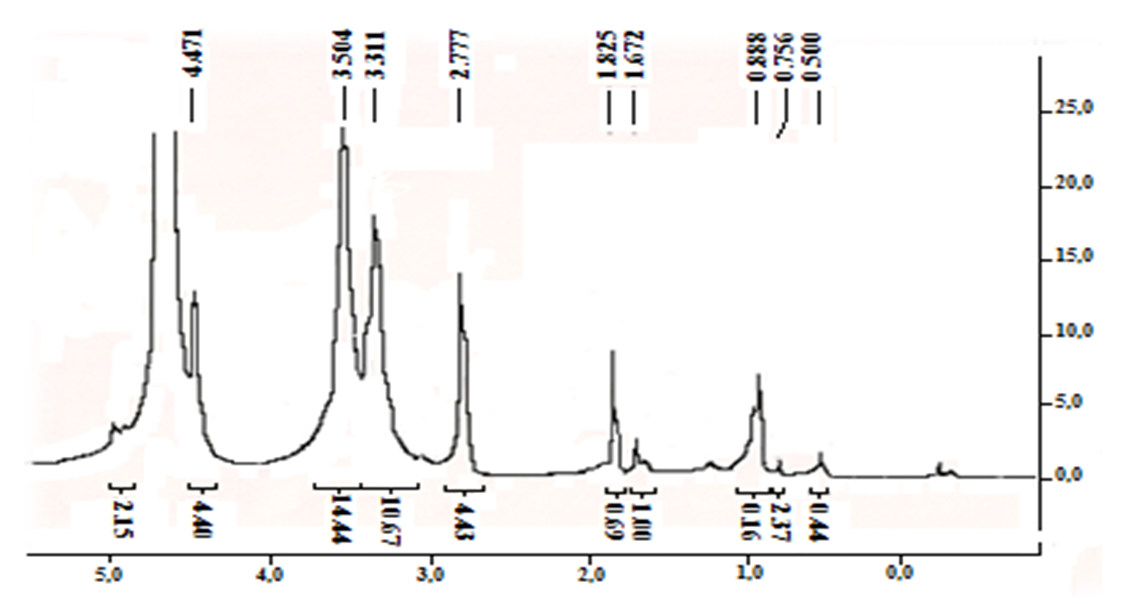 | Figure |
So, in a fully acetylated product, the ratio of the intensity of the proton signal of the glucosamine fragment, for example, a well-differentiated signal with a chemical shift of 2,78 m.d., and the signal of the acetyl group at 1,83 m. d., would be 1:3. According to this spectrum, it is 4,43:1, or 1:0.225, which corresponds to the proportion of acetylated amino groups of 7.5% (0.225: 3 x 100%). Thus, our optimized method allows us to achieve a very good degree of chitin deacetylation. In this case, the spectrum identifies the presence of a certain amount of impurities that could not be separated during the isolation and purification of chitosan. However, given that the nitrogen content of the original chitin (4,55%) indicates the content of the main substance no more than 71%, we can say that the lion's share of impurities was separated by us.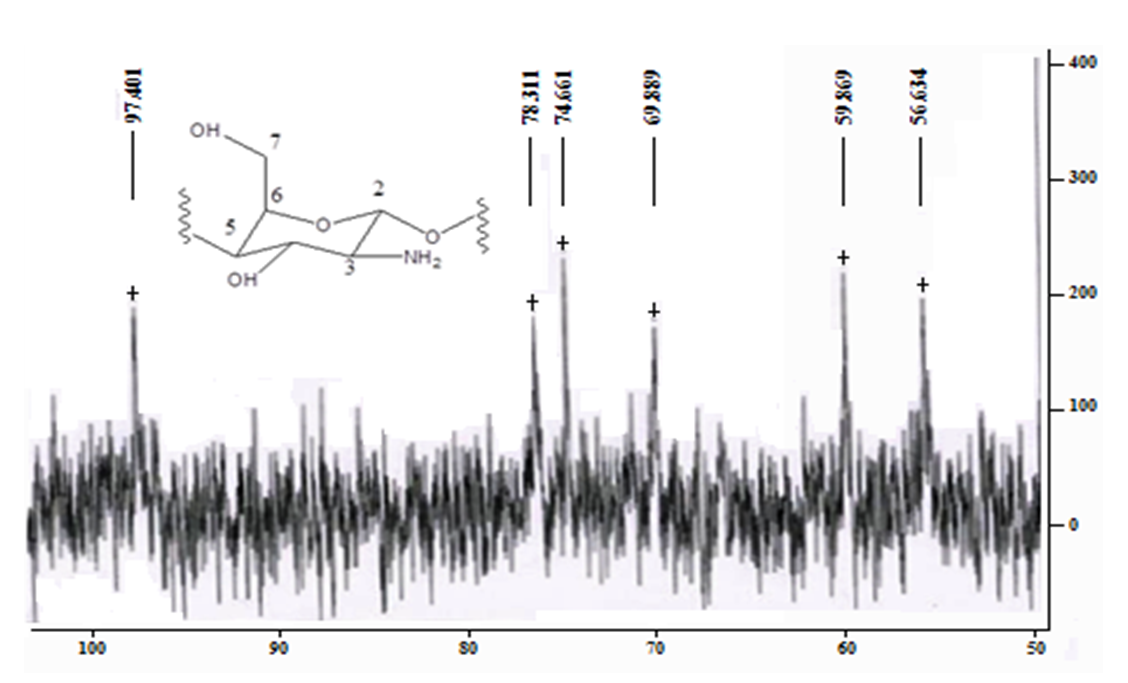 | Figure 2. NMR1H spectrum (after Fourier transform) (a) and 13C (recorded in continuous scan mode) (b) of chitosan from Artemia cysts of the Aral sea |
Figure 2 shows the 13C NMR spectrum of the same product. The chemical shifts of the observed signals are well consistent with the structure of the glucosamine fraction of the biopolymer. Thus, the weakest complete signal (97.4 m. D.) is converted to a C-2 atom bonded to two oxygen atoms, and the strongest (55.6 m. D.) to a carbon of the methylene group (C-7). The With a chemical shift of 59.9, the signal refers to the nitrogen-bound C-3 atom. The chemical displacements of the remaining atoms are also well consistent with the structure of the glucosamine bond.From the point of view of practical application of chitosan, not only its degree of deacetylation, but also its molecular weight is important, because the viscosity of these swollen polymer solutions is highly dependent on it.The molecular weight (MW) of chitosan obtained was determined by viscometry.The average viscosity masses of chitosans calculated according to the Mark-Cannes-Hovink formula are given in Table 4.Table 4. The average viscous masses of chitosans and the number of glucosamines are calculated according to the Mark-Cannes-Hovink formula  |
| |
|
According to viscometry, the MW of chitosan was 317 kDa, the total number of glucosamine units in it was 1974. The amount of Chinese chitosan is more than 2013 kDa, the number of glucosamine units in it is 12090. Due to its high molecular weight, the weight and viscosity of chitosan make it difficult to conduct scientific research. Thus, chitosan MW obtained from Artemia cysts of the Aral Sea is suitable for many scientific studies.A convenient tool for studying colloidal systems is the dynamic light scattering method, which is based on the ability of colloidal solutions to scatter some of the transmitted light. The method allows you to determine the size of colloidal particles, such as micelles.The CCM of chitosan from Artemia was 4.8 mg / ml and -1.9 mg / ml for the reference sample. Undoubtedly, the low molecular weight chitosan obtained from Aral Sea Artemia provides a real solution at high polymer concentrations, making it a valuable object for biochemical research.In recent years, the natural polymer chitosan has been widely used in biochemistry, in particular in the production of complexes with DNA. Its usefulness as an integral part of such complexes depends on several factors. Thus, the presence of an aminogroup in the polymer structure allows it to successfully bind DNA to phosphate residues, thereby hiding its negative charge and protecting nucleic acid from nuclease cleavage. And the high hydrophilicity of the polymer ensures that the complex is “invisible” to the body’s immune system.However, the high hydrophilicity of the polymer also prevents the complex from penetrating through hydrophobic cell membranes. This poses the problem of obtaining amphiphilic chitosan derivatives that can overcome this barrier.The transfer of the amphiphilic properties of the chitosan chain is accomplished by partial acylation or alkylation of the amino groups in its composition using reagents containing a long ("fat") hydrocarbon fragment.However, both acylation and alkylation reactions of chitosan are hampered by the insolubility of the biopolymer in any organic solvents, which requires special approaches to its modification.To obtain the noble anhydrides, we chose the mixed anhydride method because the mixed anhydrides, unlike anhydrides and chlorohydrides, are slowly hydrolyzed in water and mainly react with the amino group to form the corresponding N-acid derivative.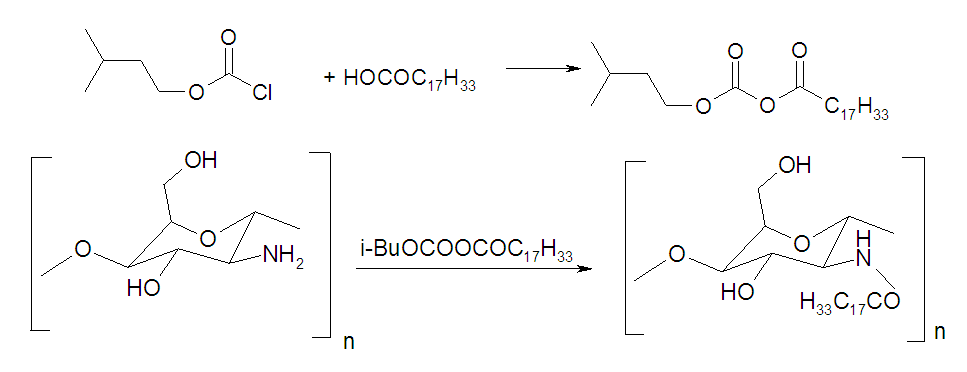 | Figure 3. Obtaining the N-oleoylchitosan reaction |
It was found that in the selected solvent system, the hydrolysis of the mixed anhydride almost occurred, resulting in a standard treatment of the reaction mixture, we obtained a product with a moderate degree of acylation, which is also soluble in aqueous solutions of acids. organic solvents.The IR spectra of the chitosan noble product are recorded in two modes - transmission and reflection for more information, as the amphiphilicity of the product makes it difficult to record the spectrum. The product spectrum includes strong bands of 2922 and 2854 cm-1 alkyl C-H vibrations. Vibrations of the hydroxyl group occur in the area of 3427 cm-1. Amide bands are recorded at 1636 and 1532 cm-1 (conduction mode), and oscillations of the non-acidic amino group are recorded at 1410 cm-1. The weak oscillation range of the two-way bond is covered by the C = C amide I band.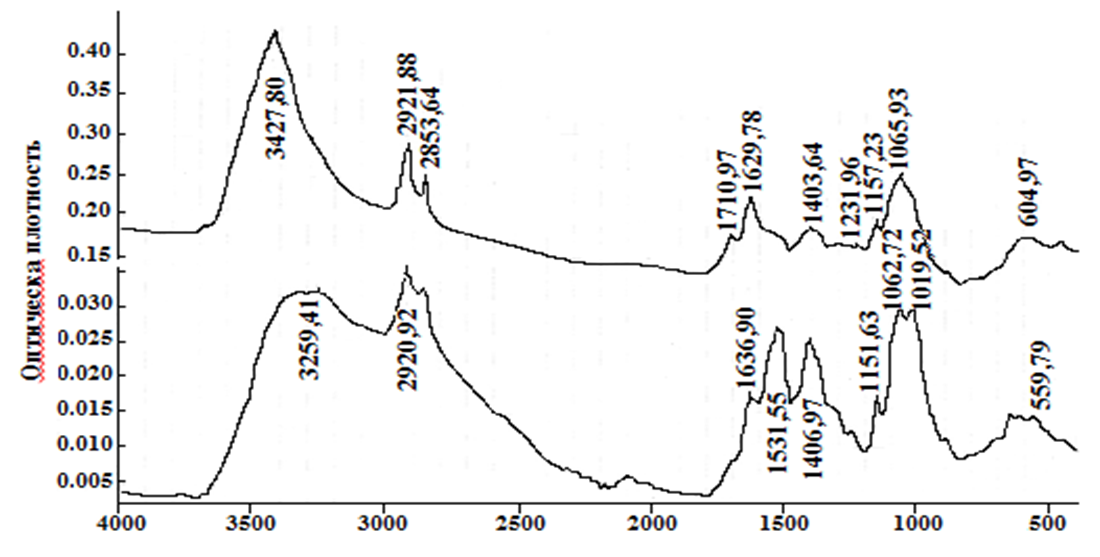 | Figure 4. IR spectrum of N-oleoylchitosan from Artemia cysts of the Aral sea |
The degree of acylation is established based on the 1H NMR spectrum of the product.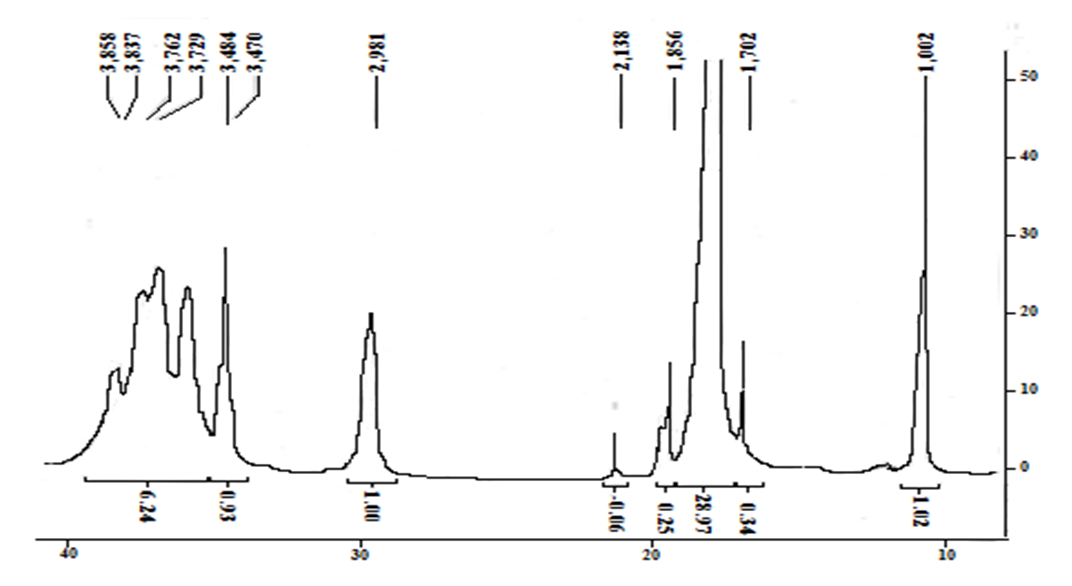 | Figure |
A comparison of the integral intensities of signal of the terminal methyl group of the oleoilic fragment (triplet at 1,09 ppm, 3H) and one of the signals of the glucasamine link, which is well differentiated from other signals (δ 2,98 ppm, 1H), is 1:1 and indicates that approximately 1 of the 3 amino groups underwent acetylation. This degree of modification is convenient for obtaining complexes with DNA, i.e., on the one hand, it contains a sufficient number of free amino groups necessary for interaction with DNA phosphates; on the other hand, the lipophilicity of the complex provided by oleoilic residues allows us to count on a good interaction with the lipid membrane of the cell.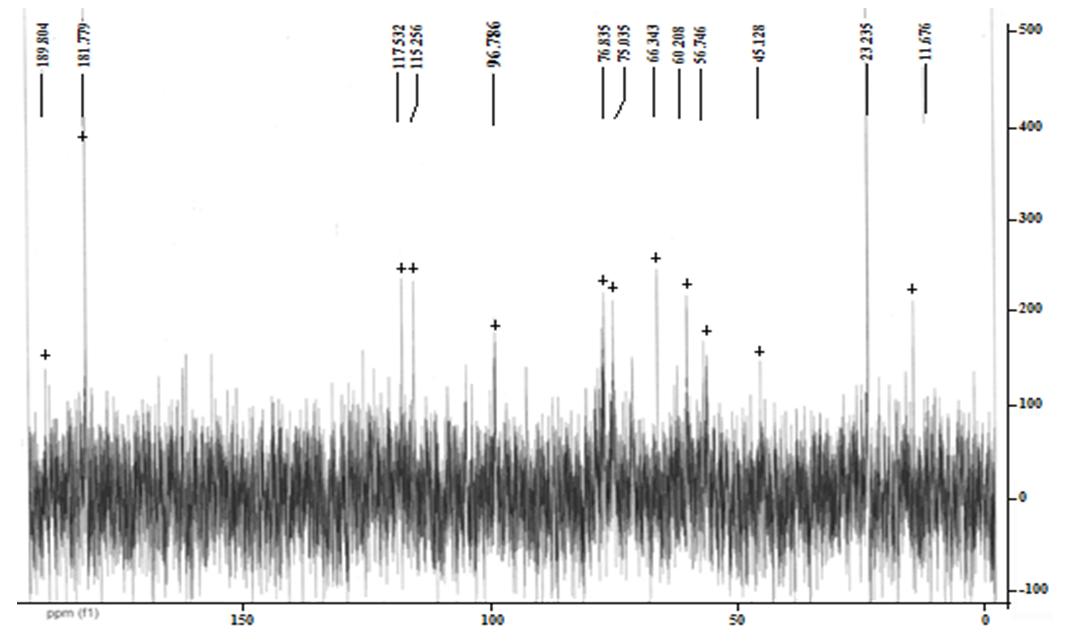 | Figure 5. NMR spectrum of 1H (a) and 13C (b) N-oleoylchitosan |
The 13C NMR spectrum also agrees well with the product structure. The spectrum contains signals of the amide carbonyl group (181,8 m.d.), double bond carbons (117,5 and 115,3 m. d.), as well as all other signals of both the glucosamine link and the acyl substituent carbons.An alternative method for obtaining amphiphilic chitosan derivatives is the reaction of alkylation on the amino group by long-chain alkyl halides. The advantage of this method is that it does not reduce the number of amino groups and, as a result, the basicity of the biopolymer. However, the implementation of this reaction is complicated by the fact that the substrate – chitosan – is soluble only in slightly acidic aqueous solutions and does not dissolve in organic solvents. In contrast, alkyl halides are only soluble in organic solvents.Therefore, to implement the reaction, we used the method of interphase catalysis.The fact that the chitosan alkylation reaction under conditions of interphase catalysis leads to the formation of the target product is confirmed by a decrease in the intensity of the free amino group vibration band (1578 cm-1) and an increase in the intensity of the C-H bond vibration bands (2920 and 2852 cm-1).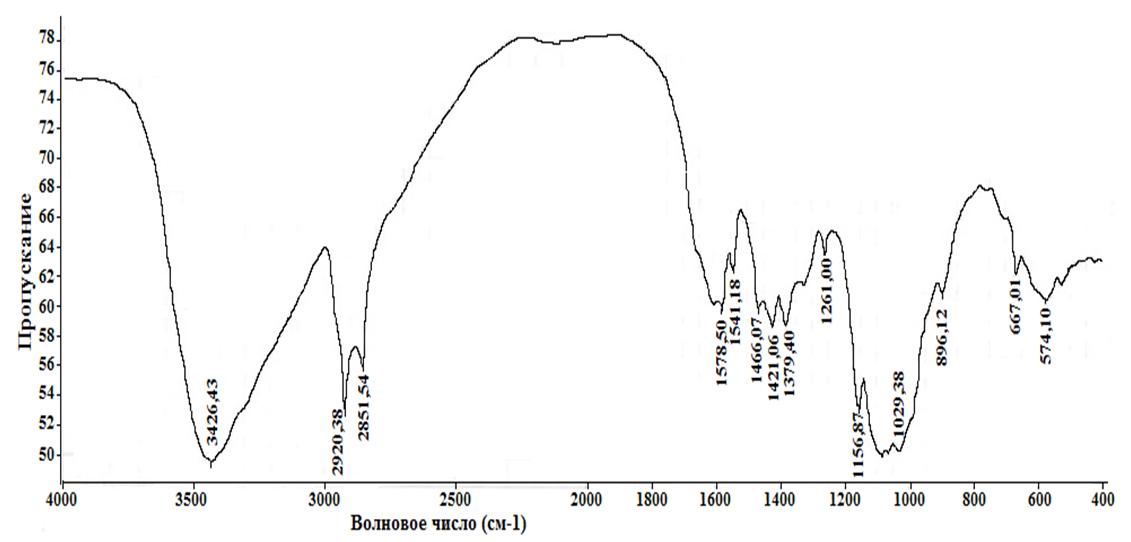 | Figure 6. IR spectrum of N-cetyl chitosan |
However, according to the data of spectroscopy 1H NMR, the degree of alkylation achieved under these reaction conditions is small. This is indicated by the small integral intensity of the signals of protons belonging to the cetyl radical: the terminal methyl group (δ 0,81 m. d.), the CH2 group directly bound to the nitrogen atom (δ 3,37 m. d., triplet). The intensity of the last signal is overestimated by the overlap with the signals of the glucosamine link. The exact degree of alkylation is difficult to determine due to the large error in integrating signals with low intensity. It is approximately 10%.Probably, to achieve a higher degree of chitosan alkylation, it is necessary to increase the amount of interphase transfer catalyst and/or the reaction time.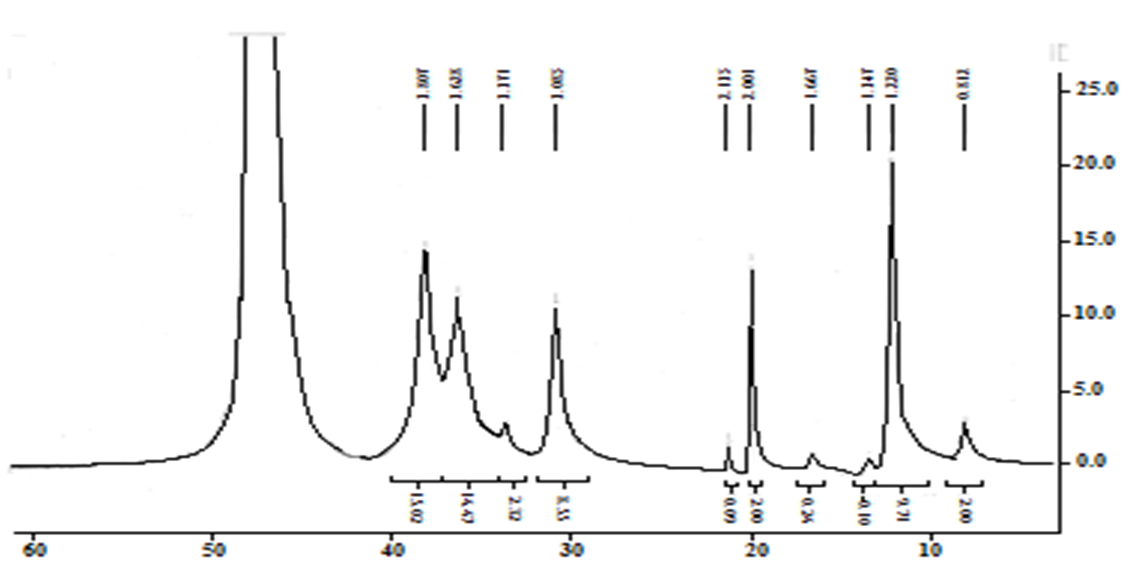 | Figure 7. 1H NMR spectrum of N-cetylchitosan |
4. Conclusions
At the result, research has shown that Artemia Parthenogenetica crustacean cysts are a good source of low-molecular-weight chitosan. The proposed methods of chemical modification of the biopolymer make it possible to obtain amphiphilic derivatives that are promising for use in biochemical research.
References
[1] | Lazurevskiy G.V., Terenteva I.V., Shamshurin A.A. «Prakticheskierabotypoximiiprirodnyxsoedineniy ("Practical works on the chemistry of natural compounds) », izd-vo «Vysshayashkola», M., 1966. |
[2] | Balabaev V.S. and dr. “Technologicnostalternativnyxsyrevyxistochnikovdlyapolucheniyapishchevogokhitozana” sovremennыe problem nauki I obrazovaniya (Technology of alternative raw sources for food chitosan" modern problems of science and education). 2005. №-1.1. |
[3] | GOST 23637. Metodyopredeleniyasoderjaniyavlagi (Methods of determining the content of moisture). |
[4] | GOST 32044.1-2012 (ISO 5983-1: 2005). Opredeleniemassovoydoliazotavavichisleniemassovoydolisirogoproteyna. (Determination of mass fraction of nitrogen and calculation of mass fraction of protein). |
[5] | GOST 13496.4-93. Metodyopredeleniyasoderjaniyaazota I sirogoproteyna (Methods for determining the nitrogen content and protein content). |
[6] | GOST 26226-95. Metodyopredeleniyasyroyzony (Methods of determining the gray area). |
[7] | Patent RF № 2246880 «Sposobpolucheniyaxitozanaizxitinarakkobraznyx» (patentobobladelVserossiyskiy NII rybnogoxozyaystva I okeanografii). ("The ability to obtain chitosan from chitorakoobraznyx" (patent holder Vserossiyskiy NII fish farm and oceanography)). |
[8] | Shipovskaya A. B. Metodyvydeleniya I fyzyko-ximicheskiesvoystvapirodnyxpolisaxaridov. (Methods of production and physical-chemical properties of natural polysaccharides). Manual. - Saratov: SGU, 2015-64.: il. |
[9] | Karlybaeva B.P., Berdimbetova G.E., Ernazarov U.K. “Ik-SpektralnyyanalizxitinavaxitozanaiztsistArtemiiAralskogomorya” ("IK-Spectral analysis of chitin and chitosan from the cyst of Artemis of the Aral Sea"). Vestnik, KKNIIEN KKO ANRUz, с.N-2018. № 4. |
[10] | Zang K., Erselen S., Tixonov V. E., Karaeva S. Z., Slita A. V., Zarubaev V. V., Meli I., Dyuportay G., Babak V. G. “Nizkomolekulyarnyealkilirovannyexitozanykaknevyrusnyevektorytransfetsiidlyagennoyterapii (“Low molecular weight alkylated chitosans as non-viral transfection vectors for gene therapy”)". Ross. xim. j. (J. Ross. Xim. Ob-vaim. D.I. Mendeleeva), 2007, t. LI, № 6. S-81-88. |
[11] | Гершкович А.А., Кибирев В.К. Химический синтез пептидов. Киев., 1992. С. 28-39. |