Richard Oyekunle Afolabi
Department of Petroleum Engineering, Covenant University, Ota, Nigeria
Correspondence to: Richard Oyekunle Afolabi, Department of Petroleum Engineering, Covenant University, Ota, Nigeria.
Email: |  |
Copyright © 2015 Scientific & Academic Publishing. All Rights Reserved.
Abstract
Associating polyacrylamide polymers have found profound use in the oil industry for Enhanced Oil Recovery (EOR) due to their improved rheological properties compared with ordinary non-associating polymers. Polymer rheology is important as it controls the mobility ratio of the displacing fluid to the displaced fluid and this can be severely affected by the high temperature and salinity present in most oil reservoirs resulting in loss of viscosity and viscous fingering of the displaced fluid. With associating polyacrylamide polymers, the presence of hydrophobic blocks on the polymer backbone allows for stronger intermolecular interaction between the polymer chains thereby improving its rheological behavior under harsh conditions obtainable in most oil reservoirs. In this project, polyacrylamide containing N-dodecylacrylamide was synthesized via micellar copolymerization in order to investigate the effect of hydrophobe variation and surfactant variation on the rheology of the prepared copolymers. Rheological measurements were carried out with the use of a Bohlin Rheometer. Improved viscous characteristics were observed with increasing hydrophobe content especially when polymer concentration exceeds the Critical Aggregation Concentration (CAC). This is due to larger hydrophobic blocks on the polymer backbone. Improved polymer viscosity was also observed when surfactant was added below its Critical Micelle Concentration (CMC.) This is as a result of the creation of mixed micelles of surfactant and hydrophobic blocks. This allows for an optimum polymer and surfactant concentration to be determined for poly (acrylamide-co-N-dodecylacrylamide) for potential application in Alkaline Surfactant Polymer (ASP) flooding involved in Enhanced Oil Recovery (EOR) projects.
Keywords:
Associating Polymers, Enhanced Oil Recovery, Critical Aggregation Concentration
Cite this paper: Richard Oyekunle Afolabi, Effect of Surfactant and Hydrophobe Content on the Rheology of Poly(acrylamide-co-N-dodecylacrylamide) for Potential Enhanced Oil Recovery Application, American Journal of Polymer Science, Vol. 5 No. 2, 2015, pp. 41-46. doi: 10.5923/j.ajps.20150502.02.
1. Introduction
The global consumption of energy is estimated to rise by 50% according to figures obtained from the United States Department of Energy (DOE) [1]. This incremental demand in energy is not expected to be met by other forms of renewable energy sources and this leaves a stout demand for oil as the principal energy source [2, 3]. Estimates from the Department of Energy (DOE) shows that nearly 67% of the United States oil remains in place after primary and secondary oil recovery techniques must have been applied and this scenario apply to other global oil reserves. This tend to leave a considerable amount of oil in reservoirs which in most circumstances is open to Enhanced Oil Recovery (EOR) methods such as polymer flooding [4]. Polymer flooding requires the use of water soluble polymers as mobility control agents. Mobility control is as a result of the high molecular weight of the polymers which increases the viscosity of the dilute solution leading to residual oil being recovered in geologic formations. The sweep efficiency of such polymers is due to reduced fingering effect which stems out of the viscous nature of the dilute solution thereby limiting the possibility of early water breakthrough [5-7]. Water soluble polymers include the partially Hydrolyzed Polyacrylamide (HPAM). Solubility of HPAMs in water is due to the polar amide group (-CONH2) and the ionic carboxylate group (-CO2-M+). The hydrodynamic volume of HPAMs is increased in water as a result of the electrostatic repulsion between the carboxylate groups (COO-) thereby elongating the polymer chains and hence its viscosity. HPAM viscosity also increases when the molecular weight of HPAM increases. Some oil reservoirs hold connate water with high salt concentration and are under extreme temperatures. Presence of salt ions upsets the repulsion amongst the carboxylate groups (by shielding of ionic groups) which brings about polymer chains coiling up and results in a decrease in the fluid hydrodynamic volume thereby causing a reduction in the fluid viscosity. Additionally, thermal breakdown of HPAM takes place at high reservoir temperature (usually above 60oC) in which complete loss of fluid viscosity occurs. Furthermore, HPAMs with high molecular weight are degraded with increasing shear rates [8, 9]. High shear rates are obtainable around the near wellbore region of the injection well in a reservoir. Shear degradation is an irreversible process whereby the polymer backbone is severed bringing about a reduction in fluid viscosity [10, 11]. These drawbacks and problems related with partially hydrolyzed polyacrylamide affects its usage in improved oil recovery operations.This project looked at the synthesis and characterization of a hydrophobically associating copolymer, poly (acrylamide-co-N-dodecylacrylamide), made up of the acrylamide monomer and N-dodecylacrylamide co-monomer. The hydrophobe of concern is the N-dodecylacrylamide co-monomer. The synthesis of the copolymer was carried out via micellar copolymerization. Micellar copolymerization was used to overcome the insolubility of the hydrophobic co-monomer by the addition of a surfactant. The characterization of poly (acrylamide-co-N-dodecylacrylamide) was carried out to define quantitative parameters such as the molecular weight and also to explore the effect of variation in parameters such as hydrophobic content and surfactant concentration and its subsequent impact on the rheology of the copolymer for potential enhanced oil recovery application.
2. Experimental Procedure
2.1. Synthesis of Poly (acrylamide-co-N-dodecylacrylamide)
The experimental conditions for the synthesis of poly (acrylamide-co-N-dodecylacrylamide) are as follows: the amount of acrylamide monomer in water was 3 wt. %, the fraction of N-dodecylacrylamide that makes up the monomer feed ranged from 1 - 3 mol. %, the surfactant (sodium dodecyl sulphate) concentration was varied from 1 - 5 wt. %. The amount of potassium persulphate, K2S2O8 was 0.3 wt. % of the monomer feed. Potassium persulphate was used as initiator. The synthesis process took place in a 700ml reactor with a mechanical stirrer attached to it and the reaction progressed at 50℃ using a thermostatic bath for 7hrs under nitrogen. The monomer conversion to poly (acrylamide-co-N-dodecylacrylamide) was about 91%. The poly (acrylamide-co-N-dodecylacrylamide) was recovered through precipitation in cold methanol and dried in oven at 50℃ for 7hrs.
2.2. Characterization of Poly (acrylamide-co-N-dodecylacrylamide)
The molecular weight of the synthesized poly (acrylamide-co-N-dodecylacrylamide) was determined for different hydrophobe content using an Ubbelohde Viscometer to carry out measurements for the reduced viscosity, relative viscosity and specific viscosity of polymer solutions based on their flow times. The reduced viscosity,
is related to the polymer concentration, C, by the Huggins’ equation: 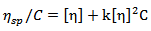 | (1) |
The measurements of viscosity were carried out at 25℃ and this was maintained using a circulating thermostatic water bath and flow times measured with a stop watch. The plot of reduced viscosity of the polymer solution against polymer concentration which is called the Huggins’ plot (Figure 1) was obtained and extrapolating the Huggins’ plot to infinite dilution in order to obtain the intercept gave the intrinsic viscosity. This intrinsic viscosity,
is related to the polymer molecular weight,
, by the Mark-Houwink-Sakurada equation below | (2) |
For this measurement in which polymer solution was prepared by dissolving the polymer in water and temperature kept at 25℃, the Mark-Houwink-Sakurada equation becomes | (3) |
Equation (3) was used to calculate the molecular weight of poly (acrylamide-co-N-dodecylacrylamide). 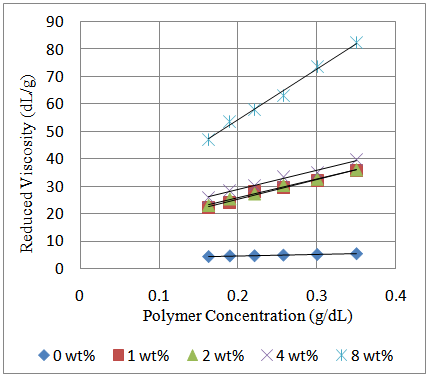 | Figure 1. Huggins’ plot of reduced viscosity against polymer concentration for polymers containing 0wt% - 8wt% N-dodecylacrylamide |
2.3. Rheological Measurements of Poly (acrylamide-co-N-dodecylacrylamide)
Rheological measurements were carried out using a Bohlin Rheometer. The shear rates were varied from 0.1/s to 100/s.
3. Results and Discussion
3.1. Effect of N-dodecylacrylamide on Molecular Weight
The synthesized poly(acrylamide-co-N-dodecylacrylamide) having 1wt%, 2wt%, 4wt% and 8wt% N-dodecylacrylamide at a concentration of 0.35g/dL exhibited increasing values for intrinsic viscosity (Table 1 and Figure 2) and hence, molecular weight. The large molecular weight can be ascribed to larger hydrophobic blocks on the polymer backbone which arises from the micelles in aqueous solution during the synthesis process containing more N-dodecylacrylamide molecules in it (Figure 4). The more N-dodecylacrylamide molecules enclosed in each micelle, the larger the hydrophobic block on the polymer backbone which brings about an increase in the molecular weight (Figure 3).Table 1. Intrinsic Viscosity and Polymer Molecular Weight 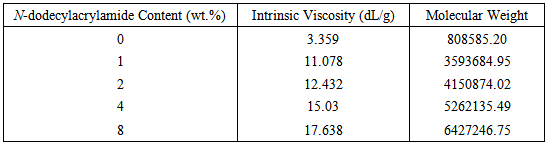 |
| |
|
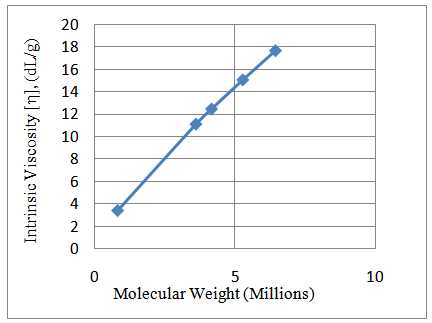 | Figure 2. Intrinsic viscosity and polymer molecular weight for hydrophobe content 0 - 8wt% at concentration of 0.35g/dL and temperature of 25°C |
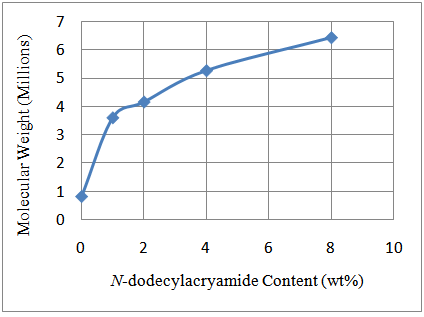 | Figure 3. Influence of N-dodecylacrylamide on molecular weight of polymer |
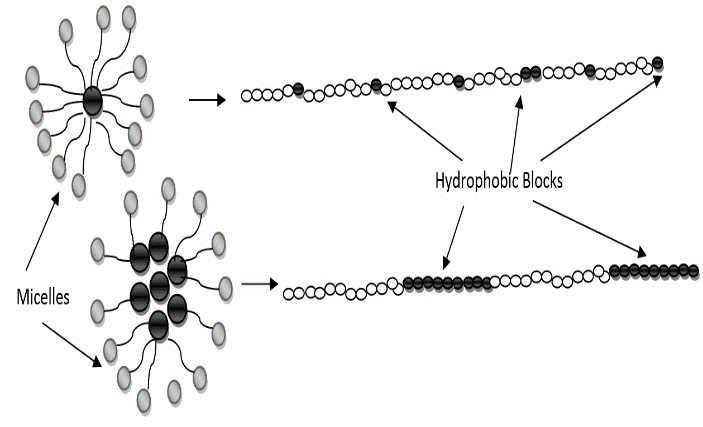 | Figure 4. Influence micelle containing N-dodecylacrylamide on hydrophobic block on polymer backbone and its molecular weight |
The hydrophobic blocks of N-dodecylacrylamide on the polymer chain impact on the polymer rheology through its contact with other polymer chains. Larger hydrophobic blocks on the polymer backbone would bring about stronger communications between polymer chains and hence an enhanced viscous property.Figure 5 shows the trend observed with increasing amount of hydrophobe content and hence molecular weight with polymer viscosity. Intermolecular association between the chains of the polymer molecules arising from increasingly larger hydrophobic interactions allow for this trend observed with the polymer viscosity.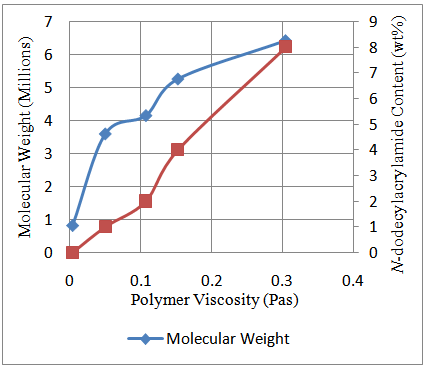 | Figure 5. Effect of molecular weight and N-dodecylacrylamide content on viscosity of poly (acrylamide-co-N-dodecylacrylamide) at a shear rate of 10.83/s and temperature 25°C |
3.2. Effect of N-dodecylacrylamide on Polymer Viscosity
The viscosity of polyacrylamide indicates a linear behavior with concentration and that of the poly (acrylamide-co-N-dodecylacrylamide) shows superior viscous behavior at concentration beyond 0.105g/dL for increasing amount of hydrophobe content. This concentration is known as the Critical Aggregation Concentration (CAC). The critical aggregation concentration splits the behavior of the polymer into two regions (Figure 6) with diverse associative features. Region 1 is characterized by polymer concentration less than CAC and exhibit low polymer viscosity. In this region, intramolecular attractions within polymer chains dictate the rheological performance of the polymer. In region 2, polymer concentration is greater than the CAC and there is a dramatic increase in polymer viscosity. With increasing N-dodecylacrylamide content, the viscosity of the polymer increases (Figure 5). This behavioral trend in region 1 and 2 can be explained using the Hydrophobic Effect. In the first region, lesser amount of polymer molecules are available in water and the presence of the polymer molecules brings about a disorder in the hydrogen bonding that exist between water molecules due to the existence of hydrophobic blocks on the polymer backbone. The hydrogen bonds are recreated when molecules of water form a Solvation Shell or Water Cage round the hydrophobic blocks. The polar nature of water molecules brings about the attraction of the “Caged” hydrophobic blocks present in solution. For the first region, there are less molecules of polymer in solution which indicates that there are fewer hydrophobic communications between molecules of polymer when water molecules move due to polar attraction permitting for intramolecular association within the molecules of polymer to control the polymer rheology.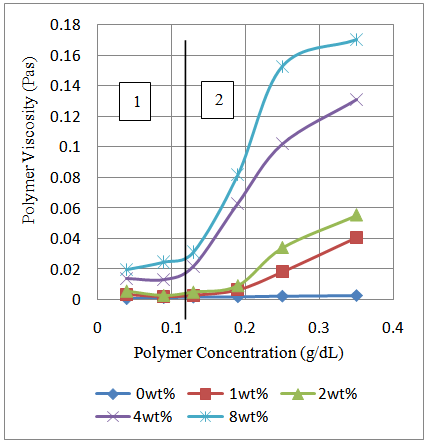 | Figure 6. Polymer viscosity as a function of polymer concentration for different content of N-dodecylacrylamide and at a shear rate of 10.83/s and temperature of 25°C |
The second region corresponds to polymer concentrations greater than the Critical Aggregation Concentration (CAC) in which association of polymer chains arises due to hydrophobic interactions among hydrophobic blocks on the backbone of the polymer chains. For this region, more molecules of polymer are in solution which indicates that additional hydrophobic blocks and additional Solvation Shells around these blocks. The translation of water molecules due to polar attraction would bring these shells into contact due to their large number in solution. As a result, intermolecular associations between polymer chains control the polymer rheology in the second region and this effect result in the large increase in the viscosity of the polymer.
3.3. Effect of Surfactant on Polymer Viscosity
The impact of surfactant (Sodium Dodecyl Sulphate) on the rheology of the prepared poly (acrylamide-co-N-dodecylacrylamide) was investigated. This took into account the effect of surfactant on polymer viscosity and also how salinity impacts on the Critical Micelle Concentration (CMC) of the surfactant together with its subsequent effect on the polymer viscosity. The concentration of the surfactant at which the viscosity of the polymer is at a maximum for the different N-dodecylacrylamide content is known as the critical micelle concentration. This corresponds to a value of about 2wt% from Figure 7. The region where surfactant concentration is less than the CMC is described by increasing viscosity of polymer with surfactant concentration up to the CMC. This viscosity improvement rises with increasing N-dodecylacrylamide content which is an outcome of the growing hydrophobic interactions among polymer chains. At low concentrations of surfactants, the hydrophobic sections existent in solution are great in number with respect to the surfactant. 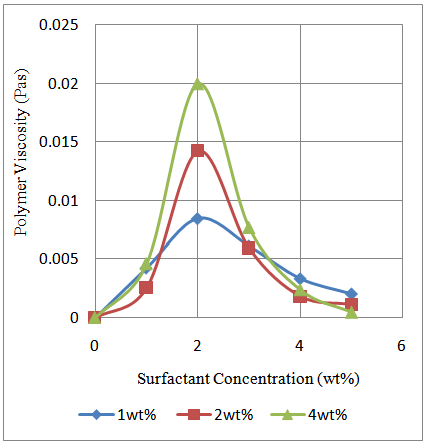 | Figure 7. Polymer viscosity and surfactant relationship for different N-dodecylacrylamide content ranging from 1wt% - 4wt%; Polymer concentration: 0.35g/dL; Shear rate: 79.43/s |
Due to this, there is no adequate number of surfactant in solution to solubilize the numerous hydrophobic regions on the polymer chains. The outcome is the formation of “Mixed Micelles” in which one micelle could solubilize 2 to 3 hydrophobic regions. The solubilization of two to three hydrophobic regions guarantees extra hydrophobic interactions which bring about viscosity improvement up to the CMC. In this particular region, not every added surfactant was involved in the formation of micelles as there are free molecules of surfactant in the solution. At CMC, any additional surfactant goes into the creation of micelles. This result in the start of a drop in polymer viscosity as there are beginning to be more micelles to enclose the several hydrophobic regions present in solution. Region 2 is characterized by a reduction in viscosity. This result from the fact that with the growing amount of micelles in solution, less hydrophobic regions in solution would be solubilize compared to the large number of micelles in solution. With this growing number of micelles, the amount of hydrophobic regions solubilized by any one micelle begins to decrease and also the hydrophobic interactions. As a result, the viscosity of the polymer begins to decrease. This continues up until one hydrophobic section is solubilized in one micelle. At this particular point the viscosity of the polymer is at its lowest value (Figure 7) as there are no hydrophobic interactions between polymer chains in solution.
3.4. Effect of Salinity and Surfactant on Polymer Viscosity
The presence of salts brings about a different rheological behaviour for the prepared poly (acrylamide-co-N-dodecylacrylamide). It was observed that there was a decline in the maximum polymer viscosity after Sodium Chloride was introduced into the system (Figure 8). This is as a result of the screening effect of metallic sodium ions existing in solution. The sodium ions in solution give the polymer chains a coiled structure thereby decreasing the hydrodynamic volume of the polymer and hence its viscosity. The molecules of surfactant in solution would solubilize the hydrophobic regions in the chains while the metallic sodium ions in solution causes the amide groups on the polymer backbone to be attracted to it due to charge screening. The metallic sodium ions are electron deficient which ensures that electrical neutrality is not sustained. As a result, the positively charged nucleus tends to attract the amide groups to it resulting in the coiled arrangement (Figure 9).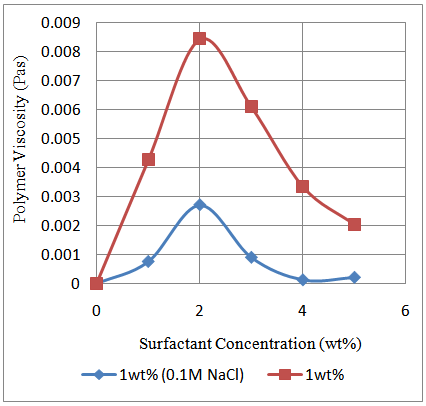 | Figure 8. Relationship between polymer viscosity and surfactant concentration in the presence and absence of NaCl. Polymer concentration: 0.35g/dL; Shear rate: 79.43/s |
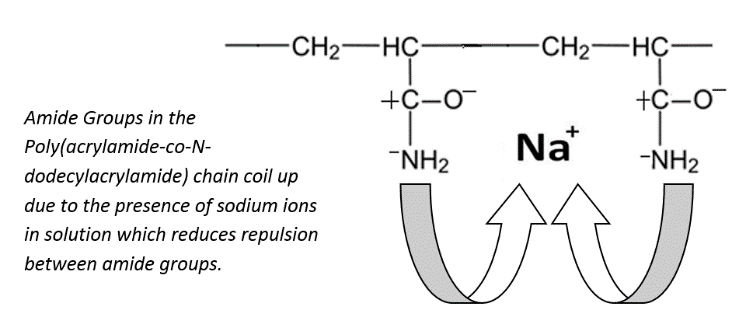 | Figure 9. Interaction between amide groups in the polyacrylamide backbone and sodium ions in solution which causes the polyacrylamide chain to coil up |
4. Conclusions
The aim of this project on polyacrylamide based associating polymers was to emphasize the impact of the hydrophobe content (N-dodecylacrylamide) and surfactant (Sodium Dodecyl Sulphate) on the rheological behavior and properties of poly (acrylamide-co-N-dodecylacrylamide) for potential application in enhanced oil recovery operations. The improved polymeric properties is evident when the hydrophobe content rises which brings about larger hydrophobic blocks on the backbone of the polymer thereby increasing its molecular weight and hence its viscosity. At polymer concentrations beyond the Critical Aggregation Concentration and at surfactant concentrations below the Critical Micelle Concentration, the viscosity of the polymer increases. This allows for an optimum polymer and surfactant concentration to be determined for potential application in Alkaline Surfactant Polymer (ASP) flooding involved in Enhanced Oil Recovery (EOR) projects.
References
[1] | H. Shamsi Jazeyi, C. Miller, M. Wong, J. Tour, and R. Verduzco, “Polymer Coated Nanoparticles for Enhanced Oil Recovery”, Journal of Applied Polymer Science. 131(15), pp. 1 – 13, 2014. |
[2] | N. Ogolo, O. Olafuyi, and M. Onyekonwu, “Enhanced Oil Recovery Using Nanoparticles”. Paper presented at the SPE Saudi Arabia Section Technical Symposium and Exhibition, Al – Khobar, Saudi Arabia, 2012. |
[3] | A. Roustaei, S. Saffarzadeh, and M. Mohammadi, “An Evaluation of Modified Silica Nanoparticles’ Efficiency in Enhancing Oil Recovery of Light and Intermediate Oil Reservoirs”, Egyptian Journal of Petroleum. 22(3), pp. 427 – 433, 2013. |
[4] | J. Ball and M. Pitts, “Effects of Varying Polyacrylamide Molecular Weight on Tertiary Oil Recovery From Porous Media of Varying Permeability”, Paper presented at the SPE/DOE Fourth Symposium on Enhanced Oil Recovery, Tulsa, OK, 1984 |
[5] | R. El-Ghazawy, and A. Atta, “High Molecular Weight Thermally Stable Poly (Sodium Methacrylate)/Magnetites Nanocomposites via Emulsion Polymerization”, International Journal of Engineering Research and Applications. 4(4), pp. 83 – 90, 2014. |
[6] | N. Yabin, O. Jian, Z. Zhu, G. Wang, G. Sun, and I. Shi, “Research on Hydrophobically Associating Water-Soluble Polymer Used for EOR”. Paper presented at the 2001 SPE International Symposium on Oilfield Chemistry, Houston, TX, USA, February 13-16, 2001. |
[7] | M. Zeynali, A. Rabi, and H. Baharvand, “Synthesis of Partially Hydrolyzed Polyacrylamide and Investigation of Solution Properties (Viscosity Behavior)”, Iranian Polymer Journal. 13 (6), pp. 479 – 484, 2004. |
[8] | J. Maerker, “Shear Degradation of Partially Hydrolyzed Polyacrylamide Solutions”. Paper presented at the SPE-AIME 49th Annual Fall Meeting, Houston, USA, October 6 – 9, 1974. |
[9] | F. Candau, S. Biggs, A. Hill, and J. Selb, “Synthesis, Structure and Properties of Hydrophobically Associating Polymers”, Progress in Organic Coatings. 33 (1994), pp. 11 – 19. |
[10] | K. Taylor, “Rheology of Hydrophobically Associating Polymers for Oil Field Applications”, Annual Transactions of the Nordic Rheology Society. 11(2003), pp. 13-18. |
[11] | K. Taylor and H. Nasr-El-Din, “Water Soluble Hydrophobically Associating Polymers for Improved Oil Recovery: A Literature Review”, Journal of Petroleum Science and Engineering. 19 (2008), pp. 265-280. |