Rania E. Morsi1, Maher Z. Elsabee2
1Department of Analysis and Evaluation, Egyptian Petroleum Research Institute, Cairo, Egypt
2Department of Chemistry, Cairo University, Cairo, Egypt
Correspondence to: Maher Z. Elsabee, Department of Chemistry, Cairo University, Cairo, Egypt.
Email: |  |
Copyright © 2015 Scientific & Academic Publishing. All Rights Reserved.
Abstract
This work deals with the preparation of polyaniline nanotubes using template free oxidation polymerization. Aniline, aniline hydrochloride and aniline sulphate were polymerized without the use of any acids or templates. Nano tubes structure was confirmed by the transmittance electron microscope imaging. The prepared polymers were characterized using FT-IR and XRD. Mercury uptake using polyaniline nanotubes was investigated at different time intervals, different adsorbent dosage and different initial concentrations. It was found that the adsorption process reached its equilibrium after 60 minutes and the process follows the pseudo second order kinetics. The uptake process was found also to work well giving complete removal at low ppb concentrations, which suggests polyaniline nanotubes to be an efficient finishing step in Hg (II) contaminated water treatment. Different chemical structure and oxidation state of PANI affect their Hg uptake capacity and was in the following order: Polyaniline hydrochloride < polyaniline sulphate < alkali-treated-polyaniline. The uptake capacity was studied for several toxic metal ions in their individual forms and as a mixture. From the experimental data of uptake capacity, the competition was in the following order, Pb (II) > Hg (II) > Cd (II) > Cu (II) > Zn (II), which was slightly in order of the electronegativity, atomic weight and atomic radius.
Keywords:
Polyaniline nanotubes, Oxidation polymerization, Template free polymerization, Heavy metal removal, Competitive uptake, Mercury, Lead, Cadmium, Copper, Zinc
Cite this paper: Rania E. Morsi, Maher Z. Elsabee, Polyaniline Nanotubes: Mercury and Competative Heavy Metals Uptake, American Journal of Polymer Science, Vol. 5 No. 1, 2015, pp. 10-17. doi: 10.5923/j.ajps.20150501.02.
1. Introduction
Polyaniline (PANI) is a promising polymer with a great potential due to its low cost, ease of preparation, good thermal and electrical properties, its environmental stabilities, and versatile applications. It possesses exceptional structural properties due to the inception of nitrogen heteroatom between the phenyl rings along the polymer backbone. Polyaniline can exist in three different forms namely Leucoemaraldine, Permigraniline and Emaraldine according to its oxidation state which is characterized by the ratio of imine to amine nitrogen. Polyaniline can be synthesized for specific applications like rechargeable batteries, biosensors, corrosion protection materials, organic electronic materials. The characteristics can surface the applicability of the synthesized PANI. It could be used in many applications such as hydrogen storages, sensors, solar cells, antistatic coatings, diodes and anticorrosive coatings. Additionally, polyaniline (PANI) possesses a large amount of amine and imine functional groups, which can interact with metal ions. Moreover,nano-scale adsorbents with large specific surface areas have higher efficient removal than the regular scale ones.Heavy metal ions in water have considered as a major preoccupation for many years because of their toxicity toward aquatic-life, plants, animals, human beings and the environment in general. As they do not degrade biologically like organic pollutants, their presence in water is a public health problem due to their absorption and therefore possible accumulation in the living organisms. Water contaminated by heavy metal ions became much more serious with the rapid development of industries and competitive use of the fresh water. Therefore, heavy metal ions removal from water is an important subject today. It is necessary to remove these ions from the waste waters before releasing into the environment. Beside other toxic heavy metals as lead, copper, zinc and cadmium, mercury poses a serious risk to the environment and endangers public health as well as the environment. Mercury (Hg) is well known to be one of the most toxic metals known in natural ecosystems. It is known that Hg may be absorbed through the gastro-intestinal tract and through the skin and lungs. Soluble compounds of mercury are particularly toxic because their adsorption is rapid. Nevertheless, Hg is still being used worldwide in applications such as barometers, thermometers, pumps, and lamps, to name a few. An improved understanding of the toxic health effects of mercury and its bio-accumulative properties has led to greater regulatory control. The World Health Organization (WHO) also recommends a maximum uptake of 0.3 mg per week and 1 µg/L as the maximum acceptable concentration in drinking water. Thus, accurate and precise qualitative and quantitative detection of mercury in aqueous media is highly recommended. Several processes have been used and developed over the years to remove the metal ions. Adsorption is regarded as one of the most promising and widely used methods. In this work, uniform PANI nanofibers will be conducted via an effective one-pot synthesis method. The polymerization of aniline hydrochloride and aniline sulphate will be also performed using the same procedure. The prepared polyaniline will be investigated as an uptaking material useable for mercury contaminated water. The effect of adsorbent dosage, contact time, initial metal ions concentration in addition to the effect of the chemical structure of the produced polymers on metal uptake capacity will be studied. The selectivity of polyaniline hydrochloride will also be focused regarding to different cations; copper, zinc, cadmium, lead and mercury.
2. Experimental Part
2.1. Materials and Reagents
Aniline (Merck) was purified by vacuum distillation before polymerization. Aniline salts; aniline sulphate and aniline hydrochloride, are of analytical grad and were used without further purification. Copper, Zinc, Cadmium, Lead and Mercury Merck standard stock solutions (1000 mg/L) were used in the preparation of different metal ions concentrations. All other reagent are of analytical grad and used without further purification.
2.2. Methods and Techniques
2.2.1. Chemical Synthesis of Polyaniline (PANI), Polyaniline Hydrochloride and Polyaniline Sulphate
Polyaniline powder was synthesized through oxidation polymerization. Briefly, 5.0 g (0.054 mole) freshly distilled aniline, aniline hydrochloride or aniline sulphate was mixed with 80 ml deionized water. The mixture was stirred for 10 minutes before cooling below 5°C for 60 minutes. 0.054 mole potassium persulphate dissolved in deionized water was added in one portion to the monomer solution under low temperature vigorous stirring. After addition of the oxidant, the mixture was further stirred for a period of 60 minutes and left unstirred overnight at low temperature (0-5℃). The precipitated polymer (dark powder) was filtered and extensively washed with deionized water until the washing liquid was colorless. In case of polyaniline, the separated powder was further treated with alkali solution in order to ensure getting the un-dopped form. Briefly, polyaniline was dispersed in 0.1 mole ammonia solution for 3 hours under stirring. The polymer was then separated by filtration, washed in deionized water to remove the excess alkali. The prepared polymers were then dried at 50-60℃ in a vacuum oven, powdered in a mortar and stored.
2.2.2. Characterization of the Prepared Polymers
FT-IR spectra were recorded on a Nicolet Is-10 FT-IR Thermo-fisher scientific spectrometer. The samples were characterized by powder X-ray diffraction (XRD) using a Philips X’Pertpro Pan-analytical instrument. Data was taken for the 2θ range of 10 to 100 degrees with a step of 0.02 degree. High Resolution Transmission Electron Microscopy (HR-TEM) imaging was performed using a Jeol-JEM Japan 2100 operating at 200 kV, the samples for TEM were prepared by making sonication for samples in ethyl alcohol and depositing onto a copper coated carbon grid and then let the solvent to evaporate.
2.2.3. Metal Uptake
Assessment of metal ions adsorption by the prepared polymers was carried out by batch adsorption experiments. Batch experiments were performed in sealed glass flasks at room temperature. Pre-weighted amounts of the investigated polymers were added to glass flasks contain a defined volume of the desired concentration of aqueous metal solution. The flasks were shacked for the desired time, filtered and then subjected to concentration measurements. The effects of the polymer dosage (0.01, 0.05 and 0.1 g/ 10 ml of metal ion solution), contact time (10–120 min) and the initial concentration (10 ppb to 10 ppm for Hg and 10 ppm for Cu, Zn, Cd and Pb) were investigated as individual metal ion solutions as well as a mixture of the metal ions used. The amount of metal ions removed was determined by the difference of the initial concentration (Ci) and the equilibrium concentration (Ce). The percentage of the metal ions removed from the solution was calculated as follows: | (1) |
The uptake capacity (qe) was calculated as following: | (2) |
where, V = volume of the Hg solution (L), M = weight of adsorbent (g).The concentration of the studied metals was measured before and after the treatment using atomic absorption spectrometer ZEE-nit 700P analytic-jena, Germany. Copper, zinc, cadmium and lead ions concentrations were determined by flame atomic absorption spectrometer while mercury concentration was determined using hydride system-cold vapor atomic absorption spectrometer (CV-AAS). In this technique, mercury solution as well as acid and reductant were aspirated by a multi-channel peristaltic pump. Acidified sample and reductant get into contact in the reactor for sample reduction where the atomic Hg vapor and hydrogen gas liberated in this process. The gaseous reaction products are carried by the argon stream to a gas-liquid separator. There, the gas phase (Hg vapor, argon and hydrogen) and the liquid phase are separated where the residual liquid is pumped off. Gas path selector leads the separated gases to a gold collector for Hg enrichment. The enriched Hg then liberated in baking out the gold collector and carried out to the quartz cell by an argon stream where the free Hg atoms were subjected to atomic absorption measurements.
3. Results and Discussion
3.1. Chemical Oxidation Polymerization of Polyaniline and Its Salts
Among different methods of Polyaniline synthesize, the chemical oxidation is the most widely used one. The chemical oxidation polymerization is practically viable due to feasibility of synthesis mechanism and low cost for producing Polyaniline. Powder nanotubes of polyaniline, polyaniline hydrochloride and polyaniline sulphate were prepared using oxidation polymerization method without using any templates or acids. Polyaniline was further alkali-treated to get the free un-dopped emeraldine base form. Polyaniline hydrochloride and polyaniline sulphate were used as prepared without further treatment.
3.1.1. FT-IR Spectroscopy
The FT-IR peaks of the backbone structures of PANI, PANI-hydrochloride and PANI- sulphate nanotubes are similar to each other. Figure 1 shows the FT-IR spectrum of the prepared materials. The main typical bands are assigned as follow; the bands at 829 and 1145 cm-1 are due to the aromatic C-H in-plane bending and the out-of-plane deformation of C-H in the 1, 4-disubstituted benzene ring. The C-H out-of-plane bending vibrations band from a para-substitution pattern at 829 cm-1 and the N Q N absorption peak at 1145 cm-1 (with Q representing the quinoid ring) indicate the head-to-tail coupling of aniline monomers. The main chain characteristic bands at 1507 and 1588 cm-1 was attributed to the C=N and C=C stretching modes for the quinoid and benzenoid rings, respectively. Bands at 1300 and 690 cm-1 can be assigned to C-N stretching of the secondary aromatic amine and aromatic C-H out-of plane bending vibrations, respectively. The broad bands in the range of 3000–3500 cm-1 correspond to secondary amine stretching (N-H) vibrations. For PANI-sulphate nanotubes, beside the characteristic peaks, such as the C=C stretching vibration of quinoid (1583 cm-1) and benzenoid rings (1496 cm-1), the C–N stretching of secondary aromatic amines (1301 cm-1), and the aromatic C–H bending (1142 cm-1), the bands at 1033, 693 and 617 cm-1 can be assigned to the O=S=O stretching vibration, S–O stretching vibration, and C–S stretching vibration, respectively. For PANI-hydrochloride, the C-Cl stretching peak arises in the region 590 − 700 cm-1.
3.1.2. Transmission Electron Microscope
Polyaniline nanotubes were investigated by high-resolution transmission electron microscope (HR-TEM), as shown in Figure 2. The morphology of PANI was well-defined tubular-like structure. The average diameter for different samples was about 80 to100 nm outer diameters and 20 to 30 nm inner diameters. 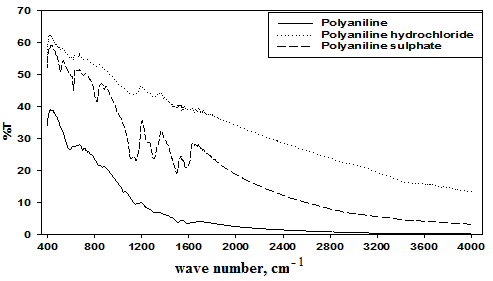 | Figure 1. FT-IR spectra of the prepared polymers |
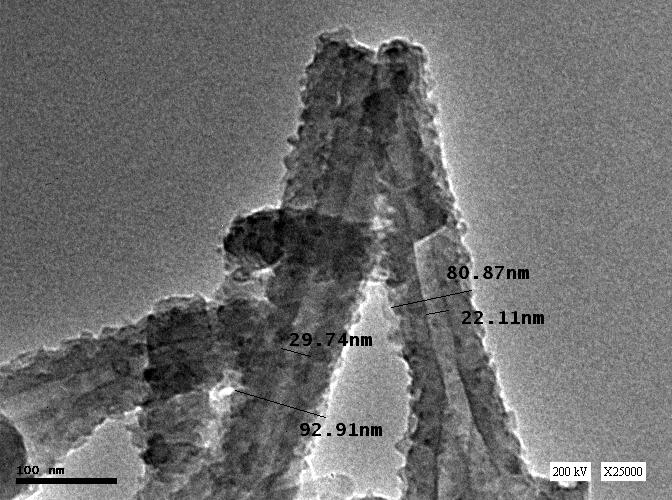 | Figure 2. HR-TEM of detailed PANI nanotubes structure |
Tubular nanofiber structures have attracted interest because of their novel properties and wide potential in the nano-scale applications. PANI nanotubes were found to be formed simply using template-free and surfactant-free polymerization process. The morphology of PANI; granular or tubular, depends on the acidity conditions during the reaction rather than on the chemical structure of the acid. Sedenkova et al. found that in solutions of strong acid, a granular PANI is produced while in solutions of weak acids or without any acid, PANI nanotubes are obtained. It is strongly suggested that the nanotubes formation mechanism of PANI is through the transformation from flake-like structure objects to self-curling nanotubes through oxidation polymerization either in weak acid or in acid-free solution. Figure 3 shows different stages of the PANI nanotubes formation as imaged by TEM. The polymerization mechanism described as follows: (1) aniline monomers form phenazine-like aniline units in the early stages of polymerization and (2) continuing the reaction produces the head-to-tail coupling of aniline molecules. The resulting PANI has a head of phenazine-like units and a tail of para-linked aniline units.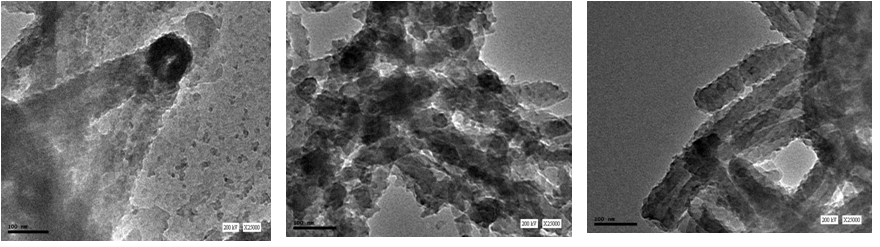 | Figure 3. TEM images of different stages of the PANI nanotubes formation |
3.1.3. XRD
Figure 4 shows the X-ray diffraction of alkali treated PANI and as prepared PANI-sulphate and PANI-hydrochloride. The diffraction patterns consisted of broad crystalline peaks and the prominent peak about 2θ= 18.55° can be attributed to the periodicity parallel to the polymer chain, whereas the peak around 2θ= 25.8° is attributed to the periodicity perpendicular to the direction of polymer chain indicating the predominant amorphous structure of the polymer chain. The resulting nanofibers show amorphous behavior. In particular, the unusual peak around 2θ= 10.83° may be assigned to the scattering along the orientation parallel to the polymer chain.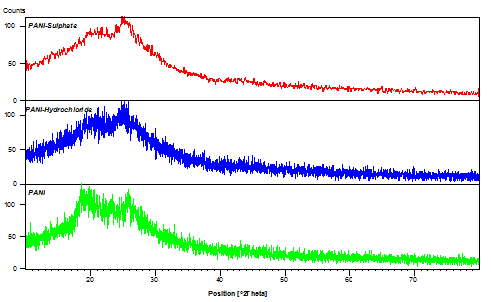 | Figure 4. X-ray diffraction (XRD) patterns of the prepared polymers |
3.2. Heavy Metals Removing
Adsorption is usually attributed to the affinity of interaction between the adsorbent active sites and the adsorbate. The adsorption capacity is affected by the adsorbent properties such as its structure, particles size, and chemistry of the surface. Polyaniline (PANI) is being considered for the removal of heavy metal ions because it possesses chelating properties attributed to the electron donating groups (amine and secondary amino groups) on the polymer chains. The driving forces of the adsorption of metal ions on PANI nanotubes are the electrostatic interaction between the basic sites of PANI (amine and imine nitrogen along the polymer backbone) and the metal cation in addition to the uptake inside the tubular structure of the polymer.
3.2.1. Effect of Adsorbent Dosage
Different adsorbent doses were used; 0.01, 0.05 and 0.1g per 10 ml of the metal solution (which are corresponding to 1, 5 and 10 g/L), keeping all other factors constant. It was found that, as the adsorbent amount increases, the uptake efficiency increases due to the availability of more active sites for the metal cation.
3.2.2. Effect of Time and the Adsorption Kinetics
Fig. 6 shows the effect of contact time on the mercury uptake efficiency using different doses of the adsorbent; PANI. As shown in the Fig., the adsorption efficiency reached the equilibrium within 60 minutes after which the uptake reached a constant value. At this point, the amount of metal ions being adsorbed on the polymer is in a state of dynamic equilibrium with the amount of metal ions desorbed.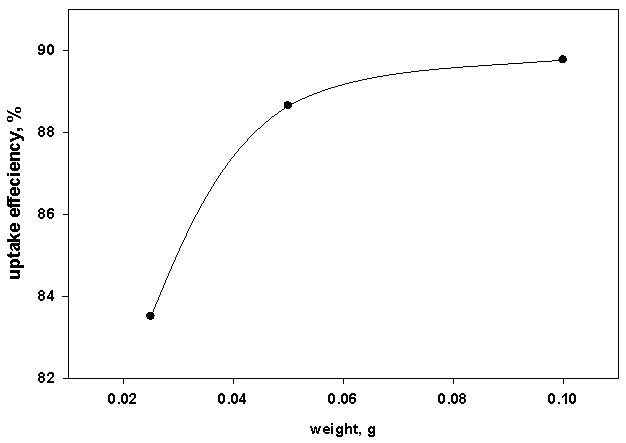 | Figure 5. Effect of PANI dosage on Hg (II) uptake efficiency |
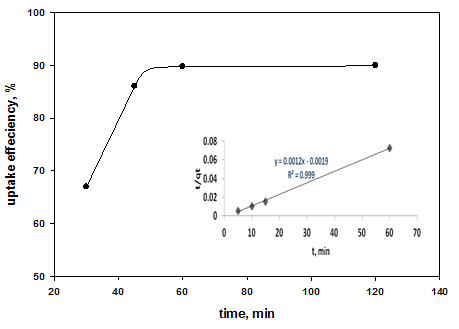 | Figure 6. (a) Effect of time on Hg (II) uptake efficiency and the insertion is the fitting of Pseudo 2nd Order kinetic model |
For further analysis of the results, four common equations were tested to find the best-fitted model for the experimental data obtained. The pseudo-first order kinetic model was suggested by Lagergren for the adsorption of solid/liquid systems and its linear form can be formulated as: | (3) |
The 1st order kinetic model linear form can be formulated as: | (4) |
Ho and McKay’s pseudo-second order kinetic model can be expressed as: | (5) |
The 2nd order kinetic model linear form can be formulated as: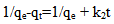 | (6) |
where qe and qt are the amount of Hg (II) adsorbed at equilibrium (mg g-1) and time t, respectively. k1 is the equilibrium rate constant of the pseudo-first order adsorption (min-1). k1p is the equilibrium rate constant of the first order adsorption (min-1). k2 is the equilibrium rate constant of the pseudo-second order adsorption (g mg-1 min-1).k2p is the equilibrium rate constant of the second order adsorption (g-1 mg-1 min-1).The experimental data have been fitted by the mentioned kinetics models. Based on the R2 value of the linear form for various kinetics models, the pseudo-second order model was more appropriate to describe the adsorption kinetics behaviors for Hg (II) ions. Accordingly, the chemisorptions were the rate controlling mechanism. The fitting results of the pseudo-second order model was shown in the insertion of Figure 6.
3.2.3. Effect of Metal Ions Concentration
Due to its dangerous effects, mercury removal process is required to be effective at very low as well as high concentrations. From figure 8, the Hg uptake of PANI was found to increase linearly with the increase of the initial concentrations of Hg. Complete removing of mercury ions in the lower range of concentration is achieved as shown in the insert of Fig. 7. At low initial concentrations of Hg (II) ions, the adsorption sites on the adsorbents were sufficient and the Hg (II) uptakes relied on the amount of Hg (II) ions transported from the bulk solution to the surfaces of the adsorbents. However, at higher initial concentrations of Hg (II) ions, the adsorption sites on the surfaces of the adsorbents reach the saturation and the adsorption of Hg (II) ions achieves equilibrium giving incomplete Hg adsorption. The expected adsorption sites are the amine and imine groups on the polyaniline backbone beside the inside of the tubular structure. The ability of polyaniline nanotubes to work well both at part per million (ppm) and parts per billion (ppb) concentration ranges gives the material an advantage of being used as Hg up taker in high concentrations and as a finishing step in the low concentrations water contamination to reach the accepted limit of Hg in the treated water.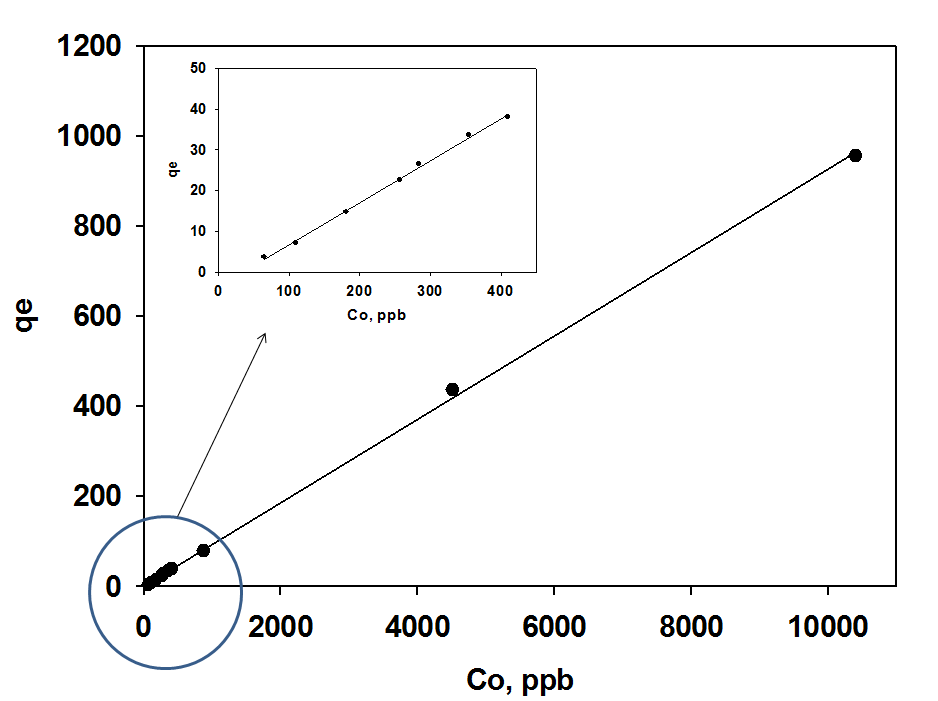 | Figure 7. Effect of concentration on Hg (II) uptake efficiency at different concentration ranges |
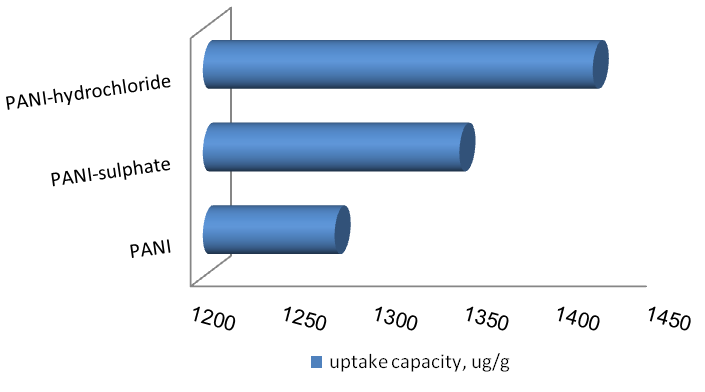 | Figure 8. Effect of chemical structure of polyaniline on the uptake capacity |
3.2.4. Effect of Chemical Structure and Oxidation State of PANI on Hg Uptake Capacity
The improvement of adsorption behavior of PANI-Hydrochloride and PANI-Sulphate in comparison with free-PANI is shown in figure 8. The improvement in the removal of mercury ions was assigned to synergetic effects of different removing mechanisms; chelation, electrostatic attraction and ionic exchange on the sulphate and chloride groups.
3.2.5. Competitive Adsorption and Selectivity of Pb(II), Hg(II), Cd(II), Cu(II) & Zn(II)
Competitive adsorption is important in water and wastewater treatment because most metal ions to be adsorbed exist with other metal ions in solution. The uptake capacity was studied for several toxic metal ions, Pb(II), Hg(II), Cd(II), Cu(II) & Zn(II) in their individual forms and as a mixture. From the experimental data of qe (µg/g) shown in figure 9, the competition was in the following order, Pb(II)> Hg(II) > Cd (II) > Cu(II) > Zn(II). All those metals are bivalent, although the differences in the capacity and thus interactions with the adsorption centers are obvious.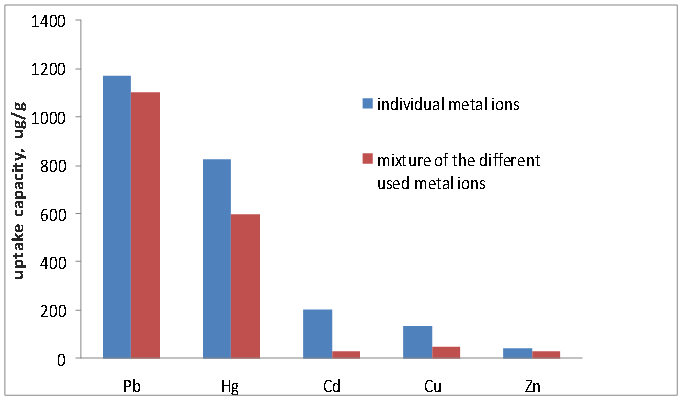 | Figure 9. Competitive heavy metal uptake in comparison with the individual metal ions |
There is no consensus regarding the competitive adsorption of metal ions. It is well known that surface physical adsorption (related to the surface area), ion exchange (related to functional groups) and redox properties (related to functional groups) can be involved into heavy metal adsorption in solution. It is interesting that the order of the amounts adsorbed agrees more or less with electronegativity and the atomic number and weight values as shown in Table 1. No straightforward dependence on other mentioned parameters.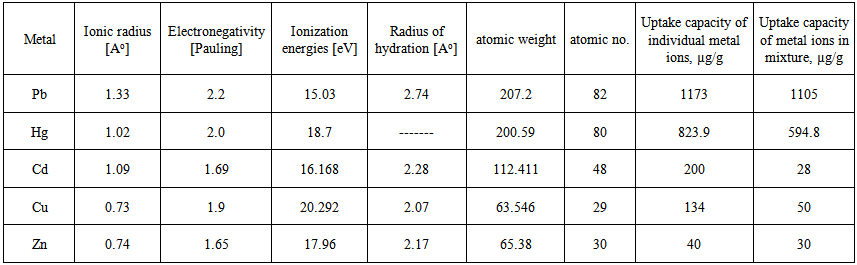 | Table 1. Electrochemical parameters and uptake capacity of Pb(II), Hg(II), Cd(II), Cu(II) and Zn(II) |
4. Conclusions
Polyaniline nanotubes were prepared without the aid of any acid or templates and then further alkali treated to get the free base structure. On the other hand, polyaniline hydrochloride and polyaniline sulphate was prepared without alkali treatment after polymerization. The driving force of the adsorption of metal ions on the free PANI is the electrostatic interaction between the basic sites of PANI (amine and imine nitrogen) and the metal cation. PANI-Hydrochloride and PANI-Sulphate nanomaterial were compared with the free-PANI for their mercury uptake capacity which was found to be in the following order: Polyaniline hydrochloride < polyaniline sulphate < free polyaniline. PANI nanotubes, in their different forms, play an important role in the selective uptake of heavy metal cations. It is suggested that it performs ion exchange in addition to the chelation by nitrogen atom and uptake inside the nano-tubes.
References
[1] | Ivana Sˇ edeˇnkova´, Miroslava Trchova, Jaroslav Stejskal, Thermal degradation of polyaniline films prepared in solutions of strong and weak acids and in water – FTIR and Raman spectroscopic studies, Polymer Degradation and Stability 93 (2008) 2147–2157 |
[2] | Materials 2012, 5, 1487-1494, Synthesis of Uniform Polyaniline Nanofibers through Interfacial Polymerization, Ahmad Abdolahi, Esah Hamzah, Zaharah Ibrahim and Shahrir Hashim |
[3] | Niemann, M.U.; Srinivasan, S.S.; Phani, A.R.; Kumar, A.; Goswami, D.Y.; Stefanakos, E.K. Room temperature hydrogen storage in polyaniline nanofibers. J. Nanosci. Nanotechnol. 2009, 9,4561–4565. |
[4] | Polyaniline nanofibers assembled on alginate microsphere for Cu2+ and Pb2+uptake,Nina Jiang, Yiting Xu, Yuqiong Dai, Weiang Luo, Lizong Dai, Journal of Hazardous Materials 215– 216 (2012) 17– 24 |
[5] | Chien-Hsin Yang, Yi-Kai Chih, Hsyi-En Cheng, Cheng-Ho Chen, Nanofibers of self-doped polyaniline, Polymer 46 (2005) 10688–10698 |
[6] | Y.F. Huang, C.W. Lin, Exploration of the formation mechanisms of polyaniline nanotubes and nanofibers through a template-free method, Synthetic Metals 159 (2009) 1824–1830. |
[7] | Mohamad Ayad, Gad El-Hefnawy, Sawsan Zaghlol, Facile synthesis of polyaniline nanoparticles; its adsorption behavior, Chemical Engineering Journal 217 (2013) 460–465. |
[8] | Chuanqin Zhang, Guicun Li, Hongrui Peng, Large-scale synthesis of self-doped polyaniline nanofibers, Materials Letters 63 (2009) 592–594. |
[9] | Atassi, Y., Tally, M., Ismail, M., 2008. Synthesis and Characterization of Chloride Doped Polyaniline by Bulk Oxidative Chemical Polymerization Doping Effects on Electrical Conductivity. Higher Institute for Applied Sciences and Technol- ogy, HIAST, P.O. Box 31983, Damascus, Syria. Transactions of the IRE Profes- sional Group. |
[10] | Ivana Sˇ edeˇnkova´, Miroslava Trchova, Jaroslav Stejskal, Thermal degradation of polyaniline films prepared in solutions of strong and weak acids and in water – FTIR and Raman spectroscopic studies, Polymer Degradation and Stability 93 (2008) 2147–2157. |
[11] | Y.F. Huang, C.W. Lin, Exploration of the formation mechanisms of polyaniline nanotubes and nanofibers through a template-free method, Synthetic Metals 159 (2009) 1824–1830. |
[12] | Polymer 50 (2009) 775. |
[13] | Y.F. Huang, C.W. Lin, Exploration of the formation mechanisms of polyaniline nanotubes and nanofibers through a template-free method, Synthetic Metals 159 (2009) 1824–1830. |
[14] | Chemical engineering journal 217 (2013) 460-465. |
[15] | Chien-Hsin Yang, Yi-Kai Chih, Hsyi-En Cheng, Cheng-Ho Chen, Nanofibers of self-doped polyaniline, Polymer 46 (2005) 10688–10698. |
[16] | Hossein Eisazadeh, Removal of Chromium from Waste Water Using Polyaniline, Journal of Applied Polymer Science, Volume 104, Issue 3, pages 1964–1967, 5 May 2007. |
[17] | Chemical engineering journal 217 (2013) 460-465. |
[18] | D. Tiwari, S.M. Lee, Biomass-derived materials in the remediation of heavy metal contaminated water: removal of cadmium (II) and copper(II) from aqueous solutions, Water Environ. Res. 83 (2011) 874–881. |
[19] | F.J. Cerino-Cordova, A.M. Garcia-Leon, E. Soto-Regalado, M.N. Sanchez- Gonzalez, T. Lozano-Ramirez, B.C. Garcia-Avalos, J.A. Loredo-Medrano, Experimental design for the optimization of copper biosorption from aqueous solution by Aspergillus terreus, J. Environ. Manag. 95 (2012) 77–82. |
[20] | Reza Ansari, Application of Polyaniline and its Composites for Adsorption/Recovery of Chromium (VI) from Aqueous Solutions Acta Chim. Slov. 2006, 53, 88–94. |
[21] | Chemical engineering journal 217 (2013) 460-465. |