Shadi Abu-Baker 1, Annette Holstein 1, Makayla Lancaster 1, Amber Abram 1, Shahrokh Ghaffari 1, Philip Garber 2, Tanya Whitmer 3, Indra Sahu 4
1Department of Chemistry and Biochemistry, Ohio University, Zanesville, Ohio, USA
2Genesis Hospital, Zanesville, Ohio, USA
3Department of Chemistry and Biochemistry, Ohio State University, Columbus, Ohio, USA
4Department of Chemistry and Biochemistry, Miami University, Oxford, Ohio, USA
Correspondence to: Shadi Abu-Baker , Department of Chemistry and Biochemistry, Ohio University, Zanesville, Ohio, USA.
Email: |  |
Copyright © 2017 Scientific & Academic Publishing. All Rights Reserved.
This work is licensed under the Creative Commons Attribution International License (CC BY).
http://creativecommons.org/licenses/by/4.0/

Abstract
2,4-Dinitrophenol’s (2,4-DNP) effect on synthetic membrane proteins was investigated to test if it is selective to cancer-mimic synthetic cell membranes, which are more bountiful in phosphatidylserine (PS) lipids compared to normal cells that have much more phosphatidylcholine (PC) lipids. Three undergraduate students prepared the untreated synthetic membranes and incorporated 2,4-DNP into a second set of treated samples. Students analysed the samples via solid-state 31P NMR. The results indicate that 2,4-DNP breaks down 1-palmitoyl-2-oleoyl-sn-glycero-3-[phospho-L-serine] (POPS) synthetic membranes, but not 1-palmitoyl-2-oleoyl -sn-glycero-3-phosphocholine (POPC) membranes that mimic the normal cell membrane composition. The 31P NMR spectrum of the head groups of the membrane suggests that the 2,4-DNP is disturbing the multilamellar vesicles (MLVs) and forming small micelles only in the POPS synthetic membranes.
Keywords:
Undergrads Research Experiment, Synthetic Membranes, Solid-State NMR
Cite this paper: Shadi Abu-Baker , Annette Holstein , Makayla Lancaster , Amber Abram , Shahrokh Ghaffari , Philip Garber , Tanya Whitmer , Indra Sahu , Investigating the Effect of 2,4-Dinitrophenol on Synthetic Membranes: An Undergraduate Research Experiment, American Journal of Organic Chemistry, Vol. 7 No. 2, 2017, pp. 19-21. doi: 10.5923/j.ajoc.20170702.01.
1. Introduction
Some membranes of cancer cells, such as pancreatic cancer, have been found to contain more phosphatidylserine (PS) lipids while normal cells contain more phosphatidylcholine (PC) lipids [1]. The chemical structures of the lipids used in this study are shown in Figure 1. The 2,4-DNP (Figure 1) has never been tested against synthetic membranes or multilamellar vesicles (MLVs) that resemble the cancer cells (POPS-lipid predominant) or the normal cells (POPC-lipid predominant). This is a cost-effective experiment and the results are promising. One of our undergraduate students is considering going to graduate school to further investigate 2,4-DNP as a potential moiety that can be added to a pro-drug that selectively targets the cancer cell. A similar approach was used to test SapC-DOPS Nanovesicles in real pancreatic cancer cell lines as a selective potential treatment of the pancreatic cancer patients [1]. 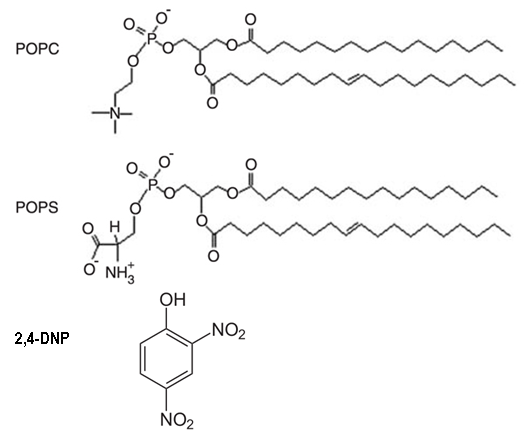 | Figure 1. The Phosphatidylcholine (POPC) chemical structure compared to Phosphatidylserine (POPS) lipids is shown in the first two panels. The chemical structure of 2,4-Dintrophenol (2,4-DNP) is shown in the last panel |
2. Experimental
2.1. Instrumentation and Materials
All synthetic phospholipids such as 1-palmitoyl-2-oleoyl -sn-glycero-3-phosphocholine (POPC);1-palmitoyl-2-oleoyl -sn-glycero-3-[phospho-L-serine] (POPS) were purchased from Avati Polar Lipids (Alabaster, AL). The phospholipids were dissolved in chloroform and stored at −20°C; N-[2- hyd roxyethyl] piperazine-N′-2-ethane sulfonic acid (HEPES), 2,4-Dinitrophenol and EDTA were obtained from Sigma -Aldrich (St. Louis, MO). 31P NMR spectra were recorded on a Bruker Avance 600-MHz WB solid-state NMR spectrometer (Bruker, Billerica, MA). The 31P NMR spectra were recorded with 1H decoupling using a solid-state NMP probe. For the 31P NMR spectra, 512 scans were taken and the free induction delay was processed using 100 Hz of line broadening. The spectral width was set to 300 ppm. All 31P NMR spectra were referenced by assigning the 85% H3PO4 31P peak to 0 ppm. The data analysis was done using Bruker’s Topspin® software.
2.2. Sample Preparation
To make multilamellar vesicles (MLVs), 40mg of the POPC or POPS lipid from Avanti was added to pear-shaped flasks. Then, 10 μL of the 2,4-DNP solution (20mg/ml) was added to a set of treated samples to form 2 mol% of 2,4-DNP in lipids. The untreated set of samples were left without adding any additional chemical and it has 0 mol% 2,4-DNP in lipids. All of the samples were dried using N2 gas. After the drying, the samples were left in a desiccator overnight. The next day, 85μL of HEPES buffer was added to each sample. The samples were then heated in a water bath with slight agitation to avoid frothing of suspension. The samples were transferred to NMR tubes in preparation for the static 31P NMR spectroscopy using the parameters reported previously [2, 3]. The multilamellar vesicles (MLVs) are made of bilayers that are relatively big and when disturbed by a toxin or a chemical that interfere with the MLV structure, it will breakdown to form a small size micelles. 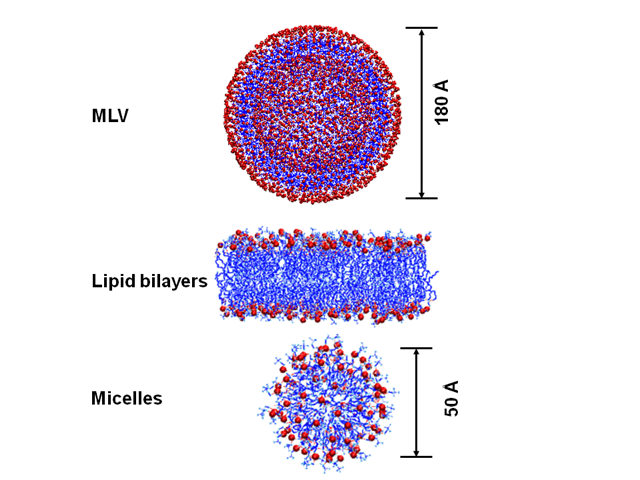 | Figure 2. The multilamellar vesicles (MLV), lipid bilayer and Micelles structures |
3. Results and Discussion
The line-shapes of the static 31P NMR spectra have been used to investigate the perturbation effect induced by proteins or drugs on phospholipids [1-13]. In this report, the static 31P NMR spectra of both POPC and POPS lipid bilayers prepared in the absence and presence of 2 mol % 2,4-DNP at 25°C are shown in Fig. 3. At room temperature, the motionally averaged powder pattern spectra are characteristic of MLVs in the liquid crystalline phase (Lα) and are expected for POPC and POPS bilayers at a temperature well above the different chain melting point transition temperatures (Tm). The Tm values are −2 and 14°C for POPC and POPS, respectively [13]. As we reported previously, POPS and POPC control samples (Fig. 3, A and C), the phospholipid molecules in the MLVs can be characterized by an axially symmetric motion with fast reorientation about the long molecular axis (gives the sharp peak at the σ-perpendicular (σ⊥) edge) and a slower reorientation rate perpendicular to that same axis [13] Conversely, the POPS MLVs containing 2 mol% 2,4-DNP (Fig. 3 D) has a sharp 31P isotropic line-shape component compared with the pure POPS MLVs (Fig. 3 C). This indicates that the axially symmetric motion of the phospholipids has been altered upon 2,4-DNP incorporation [13]. The 31P line-shape indicate that the phospholipid head groups are only perturbed by the incorporation of 2,4-DNP into POPS bilayers (Figure 3 D) and not into the POPC lipids (Figure 3 B). Additionally, the presence of the 31P NMR isotropic peak in Figure 1D suggests that the 2,4-DNP is disturbing the POPS membranes (similar to those in cancer cells) and forming smaller components such as micelles that has faster motions compared to MLVs [13]. The 2,4-DNP seems to not affect the POPC synthetic membranes (similar to those in normal cells). 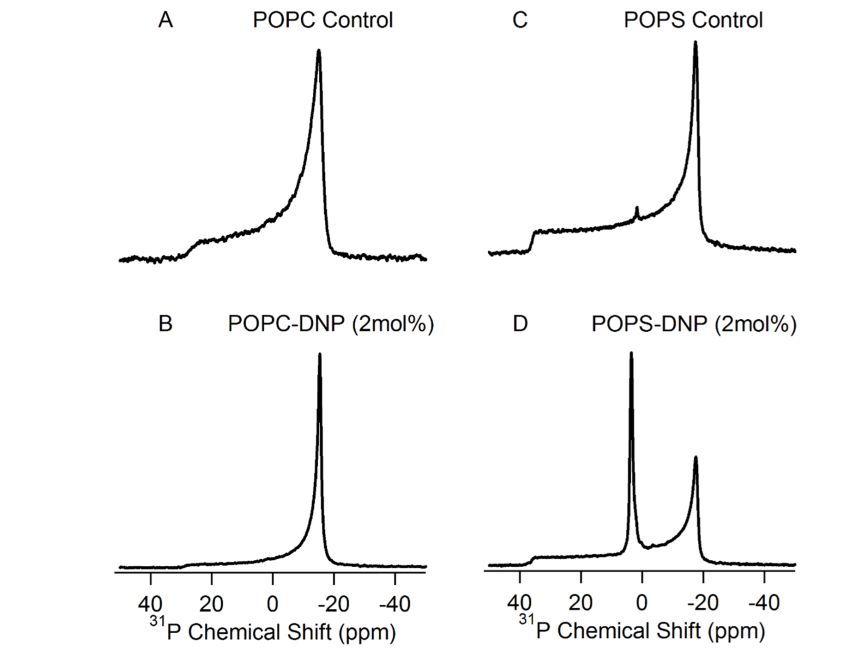 | Figure 3. 31P Solid-state NMR spectra of the MLVs of the synthetic membranes with and without 2,4-DNP treatment. The 31P chemical shift in panels (A and C) is not shown as it is in a similar scale to panels (B & D) |
A Plausible mechanism by which 2,4-DNP effects the POPS MLVs is shown in Figure 4. The 2,4-DNP disturbs the larger MLV and, as a result, small structures such as micelles may leave the MLV.
4. Conclusions
The proposed effect on 2,4-DNP on POPS MLVs is shown in figure 4. Our data suggests that the 2,4-DNP is a promising toxin and it could be connected to a sugar component to form a prodrug that could have a potential anticancer activity. We plan to synthesize the prodrug on a scale large enough to repeat the NMR experiments using the prodrug instead of the isolated 2,4-DNP in our future studies. 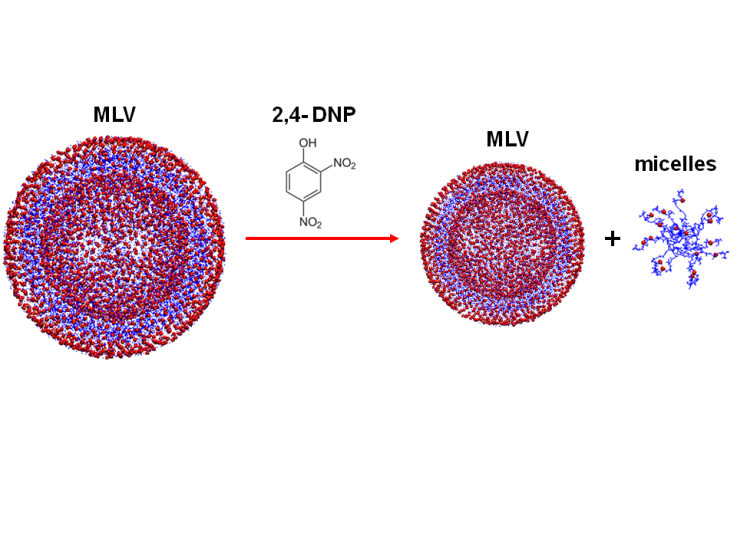 | Figure 4. A proposed mechanism of 2,4-DNP effect on POPS MLVs |
ACKNOWLEDGEMENTS
We would like to thank Genesis Hospital-Zanesville and Ohio University Zanesville for the financial support, the facilities and chemicals provided to our students to prepare the samples. We would also like to thank the Ohio State University Campus Chemical Instrument Center (OSU-CCIC) for running the samples.
References
[1] | Chu, Z., Abu-Baker, S., Palascak M.B, Ahmad, S.A., Franco R., Qi, X. “Targeting and cytotoxicity of SapC-DOPS Nano vesicles in pancreatic cancer.” PloS One, 2013, 8(10), e75507. |
[2] | Abu-Baker, S., Lorigan, G., “Solid-State NMR Spectroscopic Approaches to Investigate Dynamics, Secondary Structure and Topology of Membrane Proteins.” Open Journal of biophysics, 2012, 2(4), 109. |
[3] | Abu-Baker, S., Qi, X., Newstadt, J., Lorigan, G., “Structural changes in a binary mixed phospholipid bilayer of DOPG and DOPS upon saposin C interaction at acidic pH utilizing 31P and 2H solid-state NMR spectroscopy.” Biochimica et Biophysica Acta (BBA)-Biomembranes, 2005, 1717(1) 58-66. |
[4] | Cross, T. “Solid-State Nuclear Magnetic Resonance Characterization of Gramicidin Channel Structure.” Methods in Enzymology, 1997, 289, 672-696. |
[5] | Cross, T. and Opella, S. “Solid-State NMR Structural Studies of peptides and Proteins in Membranes.” Current Opinion in Structural Biology, 1994, 4(4), 574-581. |
[6] | Abu-Baker, S., Lu J., Chu S., Shetty K., Gor’kov, P., and Lorigan G.A., “The Structural Topology of Wild-Type Phospholamban 15N Solid-State NMR Spectroscopy.” Protein Science, 2007, 16 (11), 2345-2349. |
[7] | Seelig J., “31P Nuclear Magnetic Resonance and the Head Group Structure of Phospholipids in Membrane.” Biochimica Biophysica Acta, 1978, 515, 105-140. |
[8] | Pointer-Keenan, C., Lee, D., Hallock, K., Tan, A., Ramamoorthy, A., “Investigation of the interaction of Myelin Basic Protein with phospholipid Bilayers Using Solid-State NMR Spectroscopy.” Chemistry and Physics of Lipids, 2004, 132 (1), 47-54. |
[9] | Abu-Baker, S., and Lorigan, G., “Phospholamban and Its Phosphorylated Form Interact Differently with Lipid Bilayers: A 31P, 2H, and 13C Solid-State NMR Spectroscopic Study.” Biochemistry, 2006, 45(44) 2006, 13312-13322. |
[10] | Mack, J., Torchia, D., Steinert, P., “Solid State NMR Studies of the Dynamics and Structure of Mouse Keratin Intermediate Filaments.” Biochemistry, 1988, 27(15), 5418-5426. |
[11] | Marcotte, I., Dufourc, E., Ouellet, M., and Auger, M., “Interaction of the Neuropeptide Met-Enkephalin with Zwitterionic and Negatively Charged Bicelles as Viewed by 31P and 2H Solid-State NMR.” Biophysical Journal 2003, 85(1), 328-339. |
[12] | Watts, A., “Solid-State NMR Approaches for Studying the Interaction of Peptides and Proteins with Membranes.” Biochim Biophys Acta, 1998, 37(2), 297-318. |
[13] | Abu-Baker S., Qi X., and Lorigan G., “Investigating the interaction of Sapocin C with POPS and POPC phospholipid membrane: A solid-state NMR Spectroscopic study.” Biophysical Journal 2007, 93 (10), 3480-3490. |