Abdalhlim Hafiz, Danfeng Huang, Weigang Zhang, Ibrahim Abdurrahman, Lili Wu, Ke-Hu Wang, Khidirtajelsir, Yingpeng Su, Yulai Hu
College of Chemistry and Chemical Engineering, Northwest Normal University, Lanzhou City, China
Correspondence to: Yulai Hu, College of Chemistry and Chemical Engineering, Northwest Normal University, Lanzhou City, China.
Email: |  |
Copyright © 2017 Scientific & Academic Publishing. All Rights Reserved.
This work is licensed under the Creative Commons Attribution International License (CC BY).
http://creativecommons.org/licenses/by/4.0/

Abstract
A simple and practical approach has been developed for the synthesis of homoallylic amines which avoids the production of toxic stannanes. In this process, the hydrazone, which was formed by reacting benzaldehyde derivatives with 2-aminoisoindoline-1,3-dione, allylic bromide and tin metal under Lewis acid catalytic conditions, provides the target compounds in yields ranging from 53%-92%.
Keywords:
Tin powder, Allylation, Hydrazones, Lewis Acid, Homoallylic amines
Cite this paper: Abdalhlim Hafiz, Danfeng Huang, Weigang Zhang, Ibrahim Abdurrahman, Lili Wu, Ke-Hu Wang, Khidirtajelsir, Yingpeng Su, Yulai Hu, Lewis Acid Catalyzed Alkylation of Hydrazones by Allyl Bromide and Tin Powder: Direct access to Homoallylic Amines, American Journal of Organic Chemistry, Vol. 7 No. 1, 2017, pp. 7-12. doi: 10.5923/j.ajoc.20170701.02.
1. Introduction
Homoallylic amines are an important class of nitrogen containing compounds. Such molecules with carbon-carbon double bonds and nitrogen containing fragments can be further converted into other functional groups. [1-3] There have been many reports of the synthesis of natural products and biologically active molecules, which involve the use of homoallylic amines and their derivatives as important intermediates. [4-8] Among them, transition metal catalysts, Lewis acids and allyl organometallic reagents employed in carbon-nitrogen nucleophilic additions to the double bond are important methods for synthesis of homoallylic amines. [9-11]. Currently, “one–pot” reactions to synthesize homoallylic amine compounds exhibit a certain appeal. [12, 13].Among the available organometallic compounds, organotin compounds have good physical and chemical properties; they are widely used in the synthesis of homoallylic amines. Currently, tributyl tin is widely used, even though only one group can transfer to the product molecule and the Bu3Sn–portion must be discarded as a toxic by-product. [13-15]. Since the by-product is toxic, further treatment is required to effectively remove the Bu3Sn derivate. Conversely, the efficacious use of tin powder in some reactions to generate in situ organotin reagents has been reported in the literature. [16, 17] This not only takes advantage of the catalytic capacity of organotin reagents, but also avoids tedious organotin reagent preparation and use of highly toxic organotins, while greatly enhancing atom utilization. Herein, we describe a novel four-component reaction of a hydrazone, allyl bromide, tin powder and a Lewis acid to facilitate the synthesis of homoallylic hydrazones (Scheme 1). Compared to common single pot reactions using a carbonyl compound, an amine, allylic bromide and tin powder [18, 19], our process avoids the use of the toxic organotin reagents and permits the reactions to proceed under mild conditions (Scheme 2) to give the corresponding products in high yields.
2. Experimental
2.1. Materials and Equipment
All reagents and chemicals were purchased from Sigma Aldrich and used without further purification. Melting points were measured on an X-4 type micro-melting apparatus, and are uncorrected. The NMR spectra were collected on a Mercury 400 plus NMR instrument, using TMS as an internal standard. Petroleum ether and ethyl acetate were distilled prior to use. In the following work, petroleum ether is represented by PE, and ethyl acetate is represented by EA. Liquid column chromatography was performed using 200-300 mesh silica gel.
2.2. General Procedure for the Synthesis of N-aminophthalimide
A flask was charged with hydrazine hydrate (0.44g, 0.014mmol) in 96% ethanol (10ml), was treated with powdered phthalimide (1.20g, 0.008mmol) and mixed for 2 min at room temperature. The resulting spongy solution was observed, quickly heated and refluxed for 3min (ammonia was evaporated), then water (5ml) was added at once, followed by approximately 20mL of water. Pure N-aminophthalimide crystallized in about an hour and was recrystallized from 96% ethanol. Yield obtained was 50-60%, m.p. 200-205°C [20].
2.3. General Procedure for the Synthesis of Different Hydrazones
A benzaldehyde derivative (1.5 eq) was added to N-aminophthalamide (1.0 eq) dissolved in ethanol. Then AlCl3/CAN 20% was added to the solution and the reaction mixture refluxed until the reaction was finished. TLC was checked during the duration of reaction. The reaction mixture was extracted with EA, and column chromatography or recrystallization was performed to purify the major product.
2.4. General Procedure for the Synthesis of Homoallylic Hydrazones
To a round bottom flask was added a hydrazone (0.5mmol, 1 equiv) dissolved in 10 ml ethanol, allyl bromide (0.121g, 1mmol, 2 equiv), tin powder (0.148g, 1.25mmol, 2.5 equiv), and catalytic AlCl3 (20%, 0.013g, 0.2 equiv). The reaction system was refluxed until the reaction was finished (TLC was checked during the reaction). Then, water and NH4Cl was added to quench the reaction after removal of the ethanol and extracted.2-((1-phenylbut-3-en-1-yl)amino)isoindoline-1, 3-dione 5a: white solid; 0.134g, 92% yield, m.p. 75-76°C. 1H NMR (400 MHz, CDCl3) δ 7.76-7.75 (m, 2H), 7.68-7.66 (m, 2H), 7.43 (d, J = 6.8 Hz, 2H), 7.28 (d, J = 8.0 Hz, 3H), 5.85 (t, J = 6.8 Hz, 1H), 7.09 (d, J = 17.2 Hz, 1H), 5.0, J = 10.4 Hz, 1H), 4.49(t, J = 7.2Hz, 1H), 2.58(t, J = 7.2 Hz, 2H). 13C NMR (150 MHz, CDCl3) δ 166.8, 139.8, 134.21, 134.1, 130.1, 128.3, 127.9, 123.3, 118.2, 62.8, 39.9.HRMS (ESI): calculated for C18H16N2O2Na [M+Na] 315.1108; found 315.1104.2-((1-(p-tolyl)but-3-en-1-yl)amino)isoindoline-1, 3-dione 5a-a: Solid, 0.101g, 66% yield, m.p. 64-66oC. 1H NMR (600 MHz, CDCl3) δ 7.75-7.74 (m, 2H), 7.67-7.65 (m, 2H), 7.31 (d, J = 7.8 Hz, 2H), 7.08 (d, J = 7.8 Hz, 2H), 5.82-5.75 (m, 1H), 5.17 (d, J = 17.4 Hz, 1H), 5.07 (d, J = 10.2 Hz, 1H), 4.76 (s, 1H), 4.45 (t, J = 6.6 Hz, 1H), 2.57 (t, J = 7.8 Hz, 2H), 2.27 (s, 3H). 13C NMR (151 MHz, CDCl3) δ 166.8b, 137.6, 136.7b, 134.4, 134.1, 130.1, 129.0, 127.9, 123.3, 118.1, 62.6, 40.1, 21.1. HRMS (ESI): calculated for C19H18N2O2Na [M+Na] 329.1265; found 329.1260.2-((1-(m-tolyl)but-3-en-1-yl)amino)isoindoline-1,3-dione 5b-a: White solid, 0.102g, 66% yield, m.p. 101-102°C. 1H NMR (600 MHz, CDCl3) δ 7.76-7.75 (m, 2H), 7.67-7.66 (m, 2H), 7.26 – 7.21 (m, 2H), 7.12 (t, J = 7.2 Hz, 1H), 5.82-5.76 (m, 1H), 5.17 (d, J = 16.8 Hz, 1H), 5.07 (d, J = 10.2 Hz, 1H), 4.44 (t, J = 6.6 Hz, 1H), 2.57 (t, J = 7.2 Hz, 2H), 2.30 (s, 3H). 13C NMR (150 MHz, CDCl3) δ 166.9, 136.5, 134.3, 133.7, 133.5, 130.1, 129.2, 128.5, 127.7, 126.5, 123.4, 118.5, 62.0, 38.2. HRMS (ESI): calculated for C19H18N2O2Na [M+Na] 329.1266; found 329.1260.2-((1-(o-tolyl)but-3-en-1-yl)amino)isoindoline-1,3-dione 5c-a: White solid, 0.88g, 86% yield, m.p. 96-97°C. 1H NMR (600 MHz, CDCl3) δ 7.77-7.75(m, 2H), 7.73 (d, J = 7.2 Hz, 1H), 7.67-7.66 (m, 2H), 7.22 (t, J = 7.2Hz, 1H), 7.12 (t, J = 7.2Hz, 1H), 7.06 (d, J = 7.8Hz, 1H), 5.79-5.73 (m, 1H), 5.14 (d, J = 17.4 Hz, 1H), 5.03 (d, J = 10.2 Hz, 1H), 4.84 (t, J = 6.6 Hz, 1H), 4.71 (s, 1H), 2.56-2.52 (m, 2H), 2.32 (s, 3H). 13C NMR (151 MHz, CDCl3) δ 167.0, 137.8, 136.2, 134.3, 134.1, 130.1 (d, J = 19.3 Hz), 127.5, 127.2, 126.2, 123.3, 118.1, 58.2, 39.7, 19.3. HRMS (ESI): calculated for C19H18N2O2Na [M+Na] 329.1267; found 329.1260.2-((1-(4-methoxyphenyl)but-3-en-1-yl) amino)isoindoline-1,3-dione 5d-a: Pale yellow oil, 0.26g, 81% yield. 1H NMR (600 MHz, CDCl3) δ 7.74-7.73 (m, 2H), 7.667.64 (m, 2H), 7.32 (d, J = 8.4 Hz, 2H), 6.79 (d, J = 8.4 Hz, 2H), 5.80-5.73 (m, 1H), 5.14 (d, J = 17.4 Hz, 1H), 5.05 (d, J = 10.2 Hz, 1H), 4.74 (d, J = 2.4 Hz, 1H), 4.44-4.42 (m, 1H), 3.73 (s, 3H), 2.55 (t, J = 7.8 Hz, 2H). 13C NMR (151 MHz, CDCl3) δ 166.81, 159.23, 134.35, 134.08, 131.69, 130.03, 129.10, 123.28, 118.10, 113.64, 77.25, 77.04, 76.83, 62.22, 55.11, 40.06. HRMS (ESI): calculated for C19H18N2O3Na [M+Na] 345.1214; found 345.1210.2-((1-(3-methoxyphenyl) but-3-en-1-yl)amino)-1H-indene-1,3(2H)-dione 5e-a: Pale yellow oil, 0.131g, 82% yield. 1H NMR (600 MHz, CDCl3) δ 7.75 -7.73 (m, 2H), 7.66-7.65 (m, 2H), 7.16 (t, J = 7.5 Hz, 1H), 7.06 (s, 1H), 6.96 (d, J = 7.2 Hz, 1H), 6.76-675 (m, 1H), 5.85-5.78(m, 1H), 5.19 (d, J = 18.0 Hz, 1H), 5.09 (d, J = 10.2 Hz, 1H), 4.77 (s, 1H), 4.49 (t, J = 7.2 Hz, 1H), 3.79 (s, 3H), 2.56 (t, J = 7.2 Hz, 2H). 13C NMR (150 MHz, CDCl3) δ 166.8, 159.5, 141.5, 134.2 (d, J = 17.8 Hz), 130.0, 129.2, 123.3, 120.3, 118.3, 114.0, 112.7, 62.6, 55.2, 40.4. HRMS (ESI): calculated for C19H18N2O3Na [M+Na] 345.1215; found 345.1210.2-((1-(4-chlorophenyl)but-3-en-1-yl)amino)isoindoline-1,3-dione5f-a:Whitesolid,0.067g, 46% yield, m.p. 80-82°C. 1H NMR (600 MHz, CDCl3) δ 7.76 -7.76 (m, 2H), 7.69-7.67 (m, 2H), 7.37 (d, J = 8.4 Hz, 2H), 7.25 (d, J = 8.4 Hz, 2H), 5. 82-5.75 (m, 1H), 5.18 (d, J = 18.0 Hz, 1H), 5.10 (d, J = 10.2 Hz, 1H), 4.49 (t, J = 7.2 Hz, 1H), 2.54 (t, J = 7.8 Hz, 1H). 13C NMR (151 MHz, CDCl3) δ 166.93, 136.46, 134.16, 133.73, 133.55, 130.06, 129.20, 128.47, 127.68, 126.50, 123.40, 118.51, 77.20, 76.99, 76.77, 62.03,38.28. HRMS (ESI): calculated for C19H18ClN2O2Na [M+Na] 349.0718; found 349.0714.2-((1-(3-chlorophenyl) but-3-en-1-yl) amino) isoindoline-1,3-dione 5g-a: White solid, 0.078g, 48% yield, m.p. 76-79°C. 1H NMR (600 MHz, CDCl3) δ 7.78-7.77 (m, 2H), 7.69-7.68 m, 2H), 7.43 (s, 1H), 7.36 (d, J = 7.2 Hz, 1H), 7.24-7.19 (m, 2H), 5.83-5.76 (m, 1H), 5.19 (d, J = 16.8 Hz, 1H), 5.12 (d, J = 10.2 Hz, 1H), 4.75 (s, 1H), 4.46 (t, J = 7.2 Hz, 1H), 2.54 (t, J = 7.2 Hz, 2H). 13C NMR (151 MHz, CDCl3) δ 166.8, 142.1, 134.2, 134.1, 133.7, 130.0, 129.6, 128.2 (d, J = 3.4 Hz), 126.1, 123.4, 118.7,62.4, 40.3. HRMS (ESI): calculated for C19H18ClN2O2Na [M+Na] 349.0719; found 349.0714.2-((1-(2-chlorophenyl)but-3-en-1-yl)amino)isoindoline-1,3-dione 5h-a: White solid, 0.072g, 49% yield, m.p. 97-98°C. 1H NMR (400 MHz, CDCl3) δ 7.92 (d, J = 8.0 Hz, 1H), 7.78-7.76 (m, 2H), 7.70-7.67 (m, 2H), 7.32 (t, J = 8.8Hz, 1H), 7.24 (s, 1H), 7.20-7.14 (m, 1H), 6.00-5.89 (m, 1H), 5.24 (d, J = 17.2 Hz, 1H), 5.17 (d, J = 10.8 Hz, 1H), 5.12-5.08 (m, 1H), 2.62 (t, J = 14.4Hz, 1H), 2.56–2.48 (m, 1H). 13C NMR (150 MHz, CDCl3) δ 166.8, 137.5, 134.1, 133.9, 133.8, 130.1, 129.2 (d, J = 9.8 Hz), 128.8, 127.0, 123.4, 118.9, 58.2, 39.2. HRMS (ESI): calculated for C19H18ClN2O2Na [M+Na] 349.0720; found 349.0714.2-((1-(furan-2-yl)but-3-en-1-yl)amino)isoindoline-1,3-dione 5i-a: Pale yellow oil, 0.11g, 78% yield. 1H NMR (600 MHz, CDCl3) δ 7.78-7.76 (m, 2H), 7.69-7.67 (m, 2H), 7.33 (s, 1H), 6.21 -6.20(m, 1H), 6.18 (d, J = 2.4Hz, 1H), 5.85-5.78 (m, 1H), 5.22-5.19 (m, 1H), 5.10 (d, J = 11.4 Hz, 1H), 4.88-4.87 (d, J = 4.88Hz, 1H), 4.42-4.38 (m, 1H), 2.69 (t, J = 7.2 Hz, 2H). 13C NMR (151 MHz, CDCl3) δ 166.2, 152.6, 142.5, 134.1, 133.6, 130.1, 123.3, 118.4, 110.1, 108.4, 56.5, 36.4. HRMS (ESI): calculated for C16H14N2O3Na [M+Na] 305.0901; found 305.0897.2-((1-(thiophen-2-yl)but-3-en-1-yl)amino)-1H-indene-1,3(2H)-dione 5j-a: Pale yellow oil, 0.123, 83% yield. 1H NMR (600 MHz, CDCl3) δ 7.80-7.79 (m, 2H), 7.71-7.70 (m, 2H), 7.23 (d, J = 5.4Hz, 1H), 6.97 (d, J = 2.4 Hz, 1H), 6.88-6.87 (m, 1H), 5.88 – 5.81 (m, 1H), 5.24-5.21 (m, 1H), 5.13 (d, J = 10.2 Hz, 1H), 4.85 (d, J = 4.8Hz, 1H), 4.77-4.74 (m, 1H), 2.71-2.67 (m, 2H).13C NMR (151 MHz, CDCl3) δ 166.54, 143.10, 134.18, 133.72, 130.02, 126.28 (d, J = 17.7 Hz), 125.45, 123.39, 118.65, 77.20, 76.98, 76.77, 58.36, 40.38. HRMS (ESI): calculated for C16H14N2O2SNa [M+Na] 305.0671; found 305.0668.2-((1-(2-fluorophenyl)but-3-en-1-yl)amino)isoindoline-1,3-dione 5k-a: White solid, 0.054g, 35% yield, m.p. 83-85°C. 1H NMR δ 7.76-7.74 (m, 2H), 7.69-7.66 (m, 3H), 7.21-7.18 (m, 1H), 7.12 (t, J = 8.0 Hz, 1H), 6.92 (t, J = 8.0 Hz, 1H), 5.89-5.82 (m, 1H), 5.19 (d, J = 12.0 Hz, 1H), 5.10 ( d, J = 8.0 Hz, 1H), 4.87-4.82 (m, 2H), 2.82 (t, J = 5.2 Hz, 2H). 13C NMR δ 166.6, 161.0 (d, J = 244.5 Hz), 134.1, 133.9, 130.0, 129.3 (d, J = 6.0 Hz), 129.2 (d, J = 1.5 Hz), 126.9 (d, J = 9.0 Hz), 124.1 (d, J = 3.0 Hz), 123.3, 118.6, 115.2 (d, J = 22.5 Hz), 55.8, 38.8. HRMS (ESI): calculated for C19H18FN2O2Na [M+Na] 333.1015; found 333.1010.2-((1-phenylhex-5-en-3-yl)amino)isoindoline-1,3-dione 5l-a: Pale yellow oil, 0.101g, 50% yield. 1H NMR (600 MHz, CDCl3) δ 7.84-7.82 (m, 2H), 7.72-7.71 (m, 2H), 7.23 (t, J = 7.2 Hz, 2H), 7.19 (d, J = 7.8 Hz, 2H), 7.13 (t, J = 7.2 Hz, 1H), 5.93-5.86 (m, 1H), 5.19 (d, J = 16.8 Hz, 1H), 5.13 (d, J = 10.2 Hz, 1H), 4.58 (s, 1H), 3.34 – 3.32 (m, 1H), 2.88 – 2.83 (m, 1H), 2.67 – 2.65 (m, 1H), 2.40 – 2.36 (m, 1H), 2.32-2.27 (m, 1H). 13C NMR (151 MHz, CDCl3) δ 167.1, 141.9, 134.5, 134.2, 130.2, 128.4 (d, J = 5.9 Hz), 125.8, 123.38, 118.1, 58.3, 37.1, 34.2, 31.5. HRMS (ESI): calculated for C20H20ClN2O2Na [M+Na] 343.1421; found 343.1417.(E)-2-((1-phenylhexa-1,5-dien-3-yl)amino)isoindoline-1,3-dione 5m-a: White solid, 0.124g, 78% yield, m.p. 105-106°C. 1H NMR (600 MHz, CDCl3) δ 7.81-7.79 (m, 2H), 7.69-7.68 (m, 2H), 7.29-7.27 (m, 4H), 7.19 (t, J = 7.2 Hz, 1H), 6.43 (d, J = 16.82 Hz, 1H), 6.16-6.12 (m, 1H), 5.93-5.86 (m, 1H), 5.24 (d, J = 18.0 Hz, 1H), 5.16 (d, J = 10.2 Hz, 1H), 4.70 (d, J = 3.6 Hz, 1H), 3.98 (s, 1H), 2.50–2.46 (m, 2H), 1.58 (s, 2H). 13C NMR (150 MHz, CDCl3) δ 166.8, 142.1, 134.2, 134.1, 133.7, 130.0, 129.6, 128.0 (d, J = 4.9 Hz), 126.1, 123.4, 118.7, 62.4, 40.2. HRMS (ESI): calculated for C20H18N2O2Na [M+Na] 341.1266; found 341.1260.2-((1-(4-(trifluoromethyl)phenyl)but-3-en-1-yl)amino)-3-dione5n-a: white solid,0.123g, 68% yield, m.p.86-87°C; 1H NMR (600 MHz, CDCl3) δ 7.77-7.76 (m, 2H), 7.69-7.67 (m, 2H), 7.59 (d, J =7.8 Hz, 2H), 7.55 (d, J = 8.4 Hz, 2H), 5.85-5.78 (m, 1H), 5.22-5.19 (m, 1H), 5.14 (d, J = 10.2 Hz, 1H), 4.78 (d, J = 2.1 Hz, 1H), 4.60 (t, J = 7.2 Hz, 1H), 2.55 (t, J = 7.2 Hz, 2H).13C NMR(151 MHz, CDCl3) δ 166.8, 144.1, 134.3, 133.5, 129.9, 125.3 (q, J = 3.8 Hz), 123.4, 119.0, 62.3, 40.5. HRMS (ESI): calculated for C19H15F3N2O2Na [M+Na] 383.0984; found 383.0978.4-(1-((1,3-dioxoisoindolin-2-yl)amino)but-3-en-1-yl)benzonitrile 5o-a: Pale yellow oil, 0.103g, 65% yield; 1H NMR (600 MHz, CDCl3) δ 7.74-7.73 (m, 2H), 7.68-7.66 (m, 2H), 7.59–7.55 (m, 4H), 5.83-5.76 (m, 1H), 5.19-5.11 (m, 2H), 4.79 (s, 1H), 4.56 (t, J = 7.2 Hz, 1H), 2.53 (t, J = 4.5 Hz, 2H). 13C NMR (151 MHz, CDCl3) δ 166.7, 145.6, 134.4, 133.2, 132.1, 129.8, 128.7, 123.4, 119.3, 118.69, 111.8, 62.4, 40.4. HRMS (ESI): calculated for C19H15N3O2Na [M+Na] 340.1061; found 340.1056.
3. Results and Discussion
Initially, the allylation reaction was investigated with the stable hydrazone 1a, which was prepared from the reaction between benzaldehyde and phthalimide. The reaction was performed in refluxing ethanol and catalyzed by commercially available Lewis acids (20%) with allyl bromide as the allyl source and promoted by tin powder (Scheme 1 and Table 1). Various mole ratios, various Lewis acids and various solvents refluxing were screened during the optimization. In our investigation to achieve this allylation, we started by using the hydrazone (1equiv), allylic bromide (1equiv) and tin powder (1equiv). The corresponding homoallylic product was readily formed, but the yield was only 53% (Table 1, entry 5). In order to optimize the product yield, different mole ratios of the substrates and solvents with different Lewis acids were checked. As summarized in Table 1, the best product yield was obtained by carrying out the reaction in refluxing ethanol with a hydrazone/allylic bromide/tin/AlCl3 mole ratio of 0.5/1.0/1.25/.013, respectively. Under these conditions, 5a-a was obtained in 92% yield in 10h (Table 1, entry 4).To examine the scope of this new protocol, reactions of various hydrazones with allylic bromide were investigated (Scheme 2 and Table 2). Scheme 3 shows the one-pot, multi-step reaction sequence leading to the allylated amine product series 5. Hydrazones bearing electron-withdrawing groups such as Cl in the para, meta and ortho positions underwent allylation smoothly to furnish the desired adducts 5a in slightly lower yields (Table 2, entries 5f-a, 5g-a and 5h-a). Hydrazones with electron-donating groups in both the para and ortho position provide the corresponding homoallylic amines in good to excellent yields. 3-Phenylpropanal, (E)-cinnamylbenzaldehyde, p-trifluoromethylbenzaldehyde and 4-cyanoylbenzaldehyde also give the corresponding homoallylic amines in good yields, respectively (Table 2, entries 5l-a, 5m-a, 5n-a and 5o-a). Interestingly, when we used 2-furyl- and 2-thienyl-substituted hydrazones, the yields were very good (Table 2, entries 5i-a and 5j-a). Finally, we observed a lower product yield when o-fluorobenzaldehyde was used, producing 5k-a in only 35% yield. | Scheme 1. Optimization reaction of compound 5a-a |
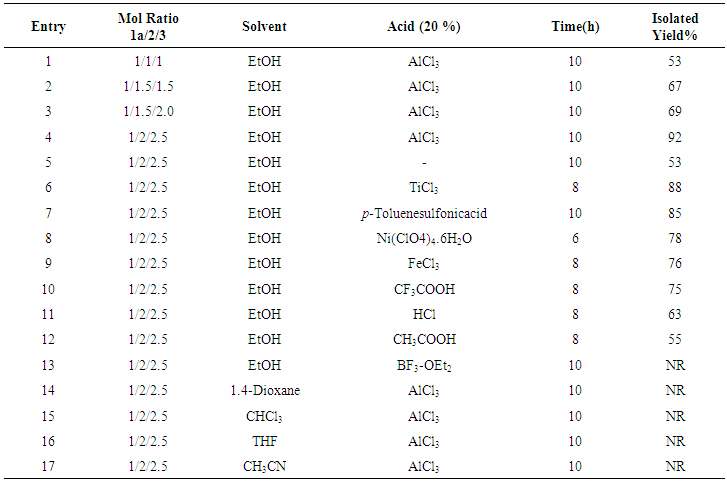 | Table 1. Allylation optimization study for the preparation of 5a-a |
 | Scheme 2. Synthesis of Homoallylic amines 5a-a - 5o-a |
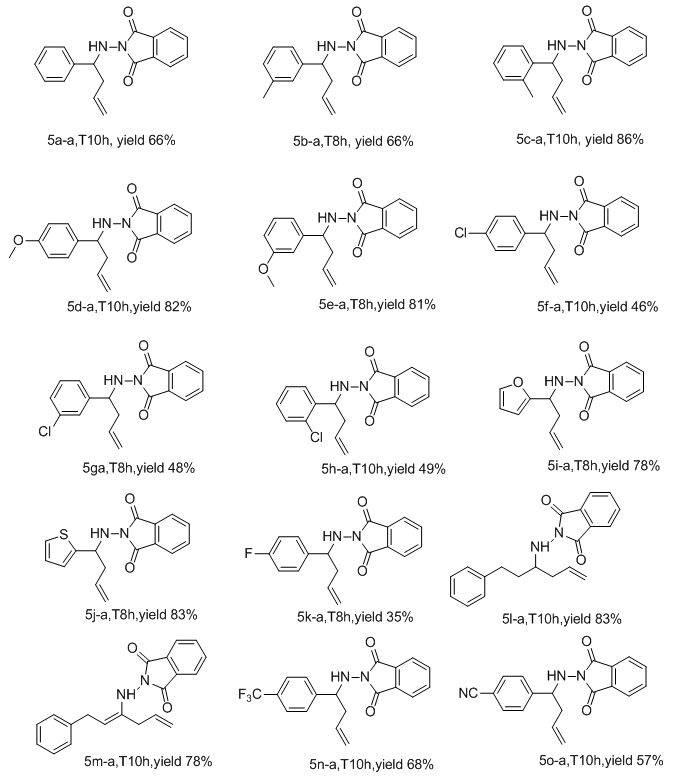 | Table 2. Homoallylic amines prepared via optimized method |
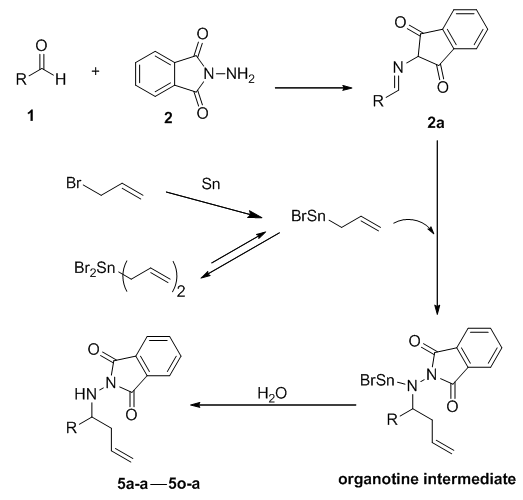 | Scheme 3. Proposed tin-mediated allylation reaction sequence |
4. Conclusions
In conclusion, we have developed an effective tin-mediated allylation reaction for the synthesis of homoallylic hydrazides from hydrazones, allyl bromide and a Lewis acid. This approach tolerates a broad substrate scope, including aldehydes with both electron-donating and electron-withdrawing substituents. Compared with reactions using different Lewis acids, this tin-promoted process has the following advantages: minimization of toxic by-products, operational simplicity, high yields and avoidance of preparation of allylic tin reagents beforehand.
ACKNOWLEDGMENTS
We are thankful for financial support from the National Natural Science Foundation of China, Key Laboratory Polymer Materials of Gansu Province (Northwest Normal University); Bioactive Product Engineering Research Center for Gansu Distinctive Plants; and State Key Laboratory of Applied Organic Chemistry Lanzhou University.
References
[1] | Piao, F., Mishra, M. K., & Jang, D. O. RETRACTED: Asymmetric synthesis of highly enantioenriched 2-substituted piperidines and 6-substituted piperidine-2-ones by a combination enantioselective hydrazone allylation with ring closing metathesis.Tetrahedron,68(35), 7050-7055. (2012). |
[2] | Morgen, M.; Bretzke, Z.; Menche, P. Li. D. Stereodivergent Synthesis of 1,3-syn-and-anti- Tetrahydropyrimidinones. Org. Lett.12, 4494-4497. (2010). |
[3] | Kropf, J. E.; Meigh, I. C.; Bebbington, M. W. P.; Weinreb, S. M. Studies on a total synthesis of the microbial immunosuppresive agent FR901483. Org. Chem. 71, 2046-2055. (2006). |
[4] | Yus, M.; Foubelo, F. Diastereoselective Allylation of Carbonyl Compounds and Imines: Application to the Synthesis of Natural Products. Chem. Rev. 113, 5595-5698. (2013). |
[5] | Pandey, M. K.; Bisai, A.; Pandey, A.; Singh, V. K. Imino-ene reaction of N-tosyl arylaldimines with a-methylstyrene: application in the synthesis of important amines. Tetrahedron Lett. 46, 46, 5039-5041. (2005). |
[6] | Schmidt, A. M.; Eilbracht, P. Synthesis of Pharmacologically Relevant Indoles with Amine Side Chains via Tandem Hydroformylation/Fischer Indole Synthesis. Org. Chem. 70, 5528. (2005). |
[7] | Allvaro, G.; Savoia, D. Addition of Organometallic Reagents to Imines Bearing Stereogenic N-Substituents. Stereochemical Models Explaining the 1,3-Asymmetric Induction. Synlett. 5, 651-673. (2002). |
[8] | Denmark, S. E.; Nicaise, O. J.-C. Ligand-mediated addition of organometallic reagents to azomethine functions. Chem. Commun. 9, 999-1004. (1996). |
[9] | Kobayashi, S.; Mori, Y.; Fossey, J. S.; Salter, M. M. Catalytic Enantioselective Formation of C-C Bonds by Addition to Imines and Hydrazones: A Ten-Year Update. Chem. Rev. 111, 2626-2704. (2011). |
[10] | Yus, M.; Foubelo, F. Catalytic Enantioselective Allylation of Carbonyl Compounds and Imines. Chem. Rev. 111, 7774-7854. (2011). |
[11] | Shen, Z.-L.; Wang, S.-Y.; Chok, Y.-K.; XuY.-L.; Loh, T.-P. Organoindium Reagents: The Preparation and Application in Organic Synthesis. Chem. Rev. 113, 271. (2013). |
[12] | Dömling, A.; Wang, W.; Wang, K. Chemistry and Biology of Multicomponent Reactions. Chem. Rev. 112, 3083. (2012). |
[13] | Dömling, A.; Ugi, I. Multicomponent Reactions with Isocyanides. Angew. Chem. Int. Ed. 39, 3168-3210. (2000). |
[14] | Roy, U. K.; Roy, S. Making and Breaking of Sn C and In C Bonds in Situ: The Cases of Allyltins and Allylindiums. Chem. Rev. 110, 2472-2535. (2010). |
[15] | Das, B.; Laxminarayana, K.; Ravikanth, B.; Ramarao, B. Efficient synthesis of homoallylic alcohols and amines using 2,4,6-trichloro-1,3,5-triazine. Tetrahedron Lett. 47, 9103. (2006). |
[16] | Lin, M. H.; Lin, W. C.; Liu, H. J.; Chang, T. H. Nitroalkeas Carbonyl Surrogates in Arylmethyl-homoallylic Alcohol Forming One-Pot Allylation Reactions in Water. Org. Chem. 78, 1278. (2013). |
[17] | Chan, T. H.; Yang, Y.; Li, C. J. Organometallic Reactions in Aqueous Media. The Nature of the Organotin Intermediate in the Tin-Mediated Allylation of Carbonyl Compounds. Org. Chem. 64, 4452-4455. (1999). |
[18] | Lu, A.; Huang, D.; Wang, K. H.; Su, Y.; Ma, J.; Xu, Y.; Hu, Y. Tin-Mediated One-Pot Synthesis of α,α-Disubstituted Homoallylic Hydrazides from Ketones, Acylhydrazines and Allyl Bromide. Synthesis, 48, 293-301. (2016). |
[19] | Li, J.; Lv, W.; Huang, D.; Wang, K. H.; Niu, T.; Su, Y.; Hu, Y. Sn-mediated one-pot four-component allylation of aldimines. Applied Organometallic Chemistry, 28, 286-289. (2014). |
[20] | Siu, T., & Yudin, A. K.). Electrochemical imination of sulfoxides using N-aminophthalimide. Org. Lett. 4, 1839-1842. (2002). |