Urbain C. Kasséhin1, 2, Hope A. Amou1, Fernand A. Gbaguidi1, Jacques H. Poupaert1, 2
1Laboratoire de Chimie Pharmaceutique Organique (MOCL), Ecole de Pharmacie, Faculté des Sciences de la Santé, Université d'Abomey-Calavi, Cotonou, Bénin
2Medicinal Chemistry Research Group (CMFA), Louvain Drug Research Institute (LDRI), Université Catholique de Louvain., Bruxelles, Belgium
Correspondence to: Urbain C. Kasséhin, Laboratoire de Chimie Pharmaceutique Organique (MOCL), Ecole de Pharmacie, Faculté des Sciences de la Santé, Université d'Abomey-Calavi, Cotonou, Bénin.
Email: |  |
Copyright © 2017 Scientific & Academic Publishing. All Rights Reserved.
This work is licensed under the Creative Commons Attribution International License (CC BY).
http://creativecommons.org/licenses/by/4.0/

Abstract
Using tetracyclone (2,3,4,5-tetraphenyl-2,4-cyclopentadien-1-one), and N-phenylmaleimide in a Diels-Alder (DA) approach, the synthesis of a lipophilic cantharidine-like phamacological probe has been designed to permit its topical use for the treatment of colon cancer or the West-African vector-borne parasitic trypanosomiasis. For the development of the target compound, special emphasis was put on implementation of green chemistry practices. This work underscored the anti-aromatic nature of tetracyclone and its consequences regarding the reactivity of this diene. Tuning of this peculiar DA reaction (n-butanol, 120°C, and 1.5 h) allowed to obtain selectively the endo-adduct with a final yield of 88%.
Keywords:
Tetracyclone, N-phenylmaleimide, Cantharidine, Diels-Alder reaction, Anti-aromatic
Cite this paper: Urbain C. Kasséhin, Hope A. Amou, Fernand A. Gbaguidi, Jacques H. Poupaert, Green Synthesis of a Cantharidine Model Compound Based on the Diels-Alder Reaction of Anti-Aromatic Tetracyclone and Maleimides as a Pharmacological Probe for Topical Application, American Journal of Organic Chemistry, Vol. 7 No. 1, 2017, pp. 1-6. doi: 10.5923/j.ajoc.20170701.01.
1. Introduction
In the course of a medicinal chemistry-driven program, our research consortium recently became interested in cyclopentadienone derivatives as a pivotal intermediate, which shares to some extent structural similarities with tetrcyclone (i.e. 2,3,4,5-tetraphenyl-2,4-cyclopentadien-1-one, 1). Tetraphenylcyclopentadienone (i.e. tetracyclone) is a dark purple to black crystalline solid that is poorly soluble in most organic solvents. The central cyclopentadienone core of the molecule is planar and conjugated with the four flanking phenyl groups. The C2-C3 and C4-C5 bond lengths are in the order of 1.35 Å, while the C1-C2, C3-C4, C5-C1 are closer to normal C-C single bonds with distances are around 1.5 Å [1]. The four flanking phenyl groups of 2,3,4,5-tetraphenyl-2,4-cyclopentadien-1-one adopt a propeller-like shape in their three-dimensional conformational behavior. The four peripheral phenyl rings are indeed rotated out of the plane of the central cyclopentadienone ring in a so-called gear-rotation situation because of the steric effect expressed by colliding ortho-hydrogen atoms (1,6-interaction, Pifzer strain) [2].In this paper, using tetracyclone as pivotal template and a Diels-Alder (DA) approach, we report our works concerned with the synthesis of a lipophilic cantharidine-like phamacological probe intentionally designed to be used topically (for example, by direct application within the intestinal lumen for the treatment of colon cancer) and more generally under the form of subcutaneously injected depot for the treatment of the Western African variant of the trypanosomiasis, which is a life-threatening parasitic (Trypanosoma brucei) vector-borne (tse-tse fly) disease and is responsible for the sleeping sickness in humans [3]. Throughout this contribution, special emphasis was put on implementation of "green chemistry" in our good laboratory practices (GLP) for the development of the target compound.
2. Results and Discussion
This peculiar structure particularly drew our attention because of its anti-aromatic character in its dienic mesomeric form (Cfr left structure in Figure 1). Indeed, so far, the unsubstituted cyclopentadienone has remained a rather elusive structure due to its intrinsic anti-aromatic instability [4]. In support of the anti-aromatic, we can cite some spectroscopic evidence, and specially the UV-VIS spectrum which allows to evidence the existence of an internal charge-transfer. A charge-transfer complex or electron-donor-acceptor complex is an association of two molecules, or of different parts of the same molecule, in which a fraction of electronic charge is transferred externally or internally between the molecular entities. Many such complexes can undergo an electronic transition into an excited electronic state. The excitation energy of this transition occurs very frequently in the visible region of the UV-VIS spectrum, which produces the characteristic intense color and often deep fluorescence of such complexes. These optical absorption bands are often referred to as charge-transfer bands. UV-VIS spectroscopy is a powerful technique to characterize charge-transfer bands. In support of above assertion comes Figure 2: indeed, inspection of the Electrostatic Potential (ESP) contour mapping of 9-fluorenoneone shows that the carbonyl within the central cyclopentadienone motif is not conjugated with the internal dienic system.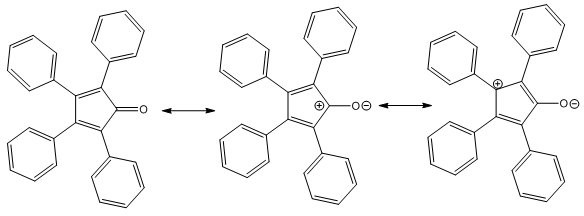 | Figure 1. A few representative canonical extreme mesomeric structures of tetracyclone generated according to the resonance theory |
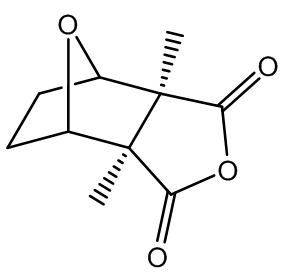 | Figure 2. Structure of cantharidine |
In this connection, a 1.0 mM dioxane solution of tetracyclone exhibits the following features in the UV-VIS range:
and 
The highest wavelength
of low extinction commonly referred to as a “charge transfer band” can be assigned to the forbidden transition from the valence bonding to that of the conduction (polarized) bonding. The appearance of such an anomalous band is frequently considered as diagnostic predictor of an anti-aromatic contributing resonance structure and it is that absorbance which gives rise to the deep dark color of tetracyclone. The 332 nm
and 259 nm
although showing a significant bathochromoic red shift, should not spark great concern at this level of our reasoning. There remains however a crucial question: if tetracyclone is in effect anti-aromatic, why does it exist and is so stable compared to unsubstituted cyclopentadienone? Reasons for such a stability are to be found firstly by the benzylic cationic character of the carbons at positions 2,3,4, and 5 owing to these four flanking phenyl groups, and secondly in the fact that tetracyclone is very insoluble and crystallizes out very quickly (even at high temperatures) from the reaction medium. Scavenged in the crystalline matrix, this high melting point compound (low motional capability in the solid matrix) does not dimerize and can be stored at room temperature for years without decomposition.As to the special anomalous nature of this “ketonic” carbonyl, we would like to point out its total lack of reactivity towards nucleophiles, even those with exceptional nucleophilicity and in particular those benefiting of the so-called “alpha-effect” such as 4-phenylthiosemicarbazide (4-PTSC). Indeed even with efficient catalysis (DMF.I2, anilinium chloride, anthranilic or sulfanilic acid, etc.) recently developed by our group or under forced harsh conditions (as for instance, refluxing in glacial acetic acid for 24h), tetracyclone opposed to 4-phenylthiosemicarbazone (4-PTSC) remained absolutely intact as judged by TLC, NMR and absence of the deep color discharge. Let us keep in mind that in the canonical extreme resonance structures, when the positive charge is located on a benzylic carbon (each four double bond carbons are eligible), this leaves the negatively charged oxygen in a stable enolate configuration and explains its non-reactivity. This total lack of reactivity towards 4-PTSC is quite reminiscent of the same puzzling behavior of the emblematic cyclopropylphenylketone, which suggests a potential participation (possibly through an anchimeric effect transmitted by a field effect) offered by the putative “sigma anti-aromaticity” of the cyclopropane ring. Indeed, the 1,3-dienic character of 1 has been largely exploited in the generation of various DA adducts. For example, 1 was used as a diene in the DA synthesis of 1, 2, 3, 4-tetraphenylnaphthalene with benzyne as dienophile [5] and it was equally employed to synthesize per-phenylbenzene (hexaphenylbenzene) using 1,2-diphenylacetylene [6]. Interestingly enough, in a similar way, pentaphenylpyridine derivatives were prepared via an unprecedented DA reaction between 1 and benzonitrile. Because of the high versatility of this reaction and the large chemical space potentially available via this route (i.e the chemodiversity centered on this template [7]), we successfully investigated a more flexible and "greener" access route to tetracyclone analogue derivatives in a previous publication [3].As to the Green Chemistry character of our present contribution, we would like to point out several avantages of our approach compared to previous contributions: (1) All solvents mentioned for running the key reaction are biodegradable and for the alcohols can be obtained by fermentation;(2) No solvent-costly chromatography was necessary for purifying the final product;(3) A rational plan was first designed before running experiments.In this connection, let us keep in mind that in principle the Diels–Alder reaction allows for generating in a single operation both the endo and exo diastereoisomeric species, a great asset in view of the ready availability of the automated separation technologies. The synthesis of tetracyclone (1) is more than well documented in the literature [8]. The title compound can be indeed prepared in principle even in good yield by consecutive aldol condensations be tween benzil and dibenzylketone (1,3-diphenyl-2-propanone) using a plethora of solvents and bases as homogeneous catalysts. Benzil is a standard building block in organic synthesis. A classic organic reaction of benzil is the benzilic acid rearrangement, in which base catalyses the conversion of benzil to benzilic acid [9]. This reactivity is exploited in the preparation of the drug phenytoin [10-11]. Benzil also reacts with 1, 3-diphenylacetone in an aldol condensation to give tetracyclone [12]. In our previous contribution, we provided the synthetic organic chemist community with a straightforward green method which we have taken advantage of in this note to study the potential accessibility of cantharidine mimeticks in an effort to generate a comprehensive compound library [13].The DA reaction remains in the realm of Synthetic Organic Chemistry a major player in creating new-formed carbon-carbon bonds. This remarkable reaction in its simplicity is the protypical example of a [4+2]
between a conjugated diene and a diversely substituted alkene, commonly termed the dienophile, to form a functionalized cyclohexene ring. This reaction was first disclosed by Otto Diels and Kurt Alder in 1928, for which they were awarded the Nobel Prize some 22 years later. This reaction now remains a “grand classic” among the concerted pericyclic reactions and it is by now commonly accepted that this reaction takes place generally via a single allegedly aromatic-like transition state, consequently without any intervention of one or several intermediates generated over the reaction coordinate of this transformation. As such, the DA reaction is governed by the law of orbital symmetry conservation and has been classified as a
cycloaddition, for it proceeds via a suprafacial-suprafacial interaction of a
system with another
system, an interaction which is thermally allowed as a
cycloaddition [14].The extent of sigma-pi delocalization along with the bond making-breaking process at the transition state are strongly influenced by the concomitant aromatization process going on. The aromaticity trends in the resulting transition state parallel the activation reaction energies; the extent of aromatization thus increases with increasing reaction rate and exothermicity. This implies that aromaticity (lowering the level of energy of the transition state) is the driving force governing most DA-cycloadditions. However, the title reaction involving an anti-aromatic substrate stabilized by the four flanking phenyl groups requires rather high temperatures (typically 120°C and over to proceed favorably in good yields). Moreover, when considering the reactive electrons involved in our peculiar reaction, there is a move from a predominance of the
(in the reactants) to a
(in the product(s)) and therefore the transition state herein can be ascribed as a state of partial sigma-aromaticity, a concept first offered by pioneering US chemist Paul von Raguey-Schleyer.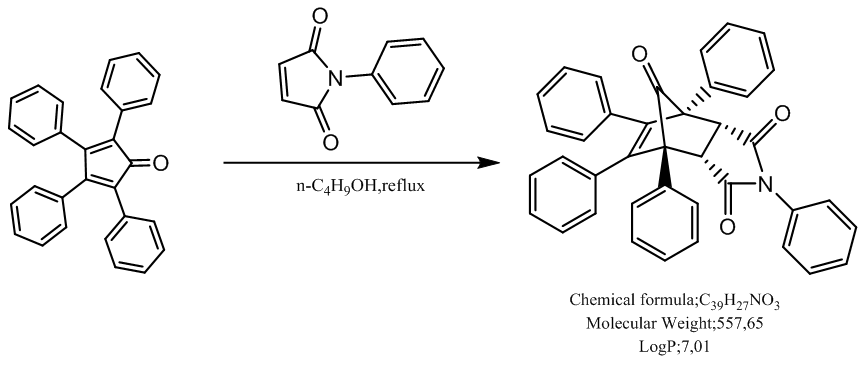 | Scheme 1. DA reaction of tetracyclone with N-phenylmaleimide (NPM) leading selectively to the endo-adduct |
N-Phénylmaleimide (NPM) was chosen for its unprecedented reactive dienophilic character in the DA reaction [3]. After tetracyanoethylene (TCNE), NPM is probably the most reactive dienophile in the DA reaction. For example, stable dienes normally are rather unreactive and undergo DA reactions only at elevated temperatures: however, anthracene and even naphthalene can function as viable dienes, leading to adducts only with highly reactive dienophiles, such as N-phenyl-maleimide. On the other hand, tetracyclone appears to be an excellent partner for NPM as it reacts for example with diphenylacetylene, a poorly reactive diene, to give hexaphenylbenzene after carbon monoxide elimination [6]. After careful optimization, we found out that the couple tetracyclone-NPM smoothly reacts to completion in refluxing n-butanol to give a single adduct, the endo-diastereoisomer species (see paragraph below for further discussion). This solvent was selected because of an adequate boiling range (bp 118°C), good solubilizing properties, and its relative low toxicity (LD50= 790 mg/kg, rat per os) [15]. In this regard, let us keep in mind that n-butanol occurs naturally as a minor component in the fermentation of carbohydrates (beer, wine) and is therefore present in many foods and beverages; it is permitted as artificial sweetener both in the United States and in the E.U.The crucial question, at this level of the discussion, remains the exact extent to which has progressed the DA reaction. In other words, is or not is carbon monoxide evolution taking place in our reaction conditions? It should be noted that whatever the temperature employed (even at room temperature), the DA reaction of tetracyclone with 1,2-diphenylacetylene proceeds down to the aromatization of the final product with concomitant carbon monoxide evolution. We reproduced that result (see Experimental Section and Figure 3). In our case, the same adduct was obtained (TLC, RMN) in several solvents while not exceeding 120°C (ethanol (80°C), n-propanol (110°C), n-butanol (120°C), and DMF (120°C)). However at higher temperature (DMF, 150°C), the product was heterogenous. In this regard, the 13C-NMR spectrum recorded in DMSO-d6 was of utmost significance as it showed a single signal at 195.15 ppm for the bridge-head ketonic carbonyl and another single one at 174.67 ppm for the two endo imidic carbonyls. This indicated that no carbon monoxide evolution took place over the time course of our reaction in the conditions mentioned above, and also that, owing to the unicity of the two types of C=O, a single diastereoisomeric species was engendered. But which one? It is known that reaction of tetracyclone with maleic anhydride gives exclusively the endo-adduct as isolable intermediate [16], which is generally the case for most DA reactions (the so-called endo rule was already enounced by Diels and Alder in 1929), before carbon monoxide evolution finally takes place at higher temperature (150°C) precluding the formation of any exo-adduct. In other words, the exo-adduct requiring a higher reaction temperature cannot be formed since this would lead primarily and therefore irremediably to the loss of carbon monoxide. Additionally, extensive semi-empirical as well as ab initio quantum mechanics calculation showed that the endo-adduct is also more stable that the exo-adduct
In this regard, a rather great similarity in the 13C-NMR spectrum of both species gave us confidence as to endo-configuration.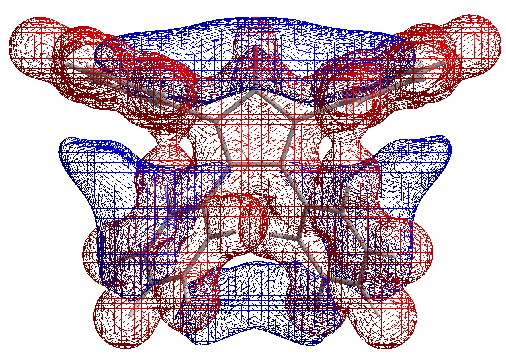 | Figure 3. Electrostatic Potential Contour Mapping of 9-fluorenoneone showing that the carbonyl within the central cyclopentadienone motif is not conjugated with the internal double bonds |
Canthraridine is known for its anticancer, herbicidal, pesticide, hair growth stimulation and aphrodisiac properties exploited in traditional medicine. However, cantharidine contains in its structure a cyclic anhydride moiety which reacts with nucleophiles present in biological surrounding and this in turn induces toxicity. There are clear indications in the literature that cantharidine targets specifically nucleophiles such as serine, threonine, and cysteine. In particular, the latter has a prominent role in the various cathepsins and in this regard, inhibition of these protein enzymes appear to be potential targets for Ebola and trypanosomiasis, a pandemic disease in Western Africa [17-19]. In a previous paper, we detailed the various considerations supporting the design of potentially druggable maleimide Diels-Alder adducts as cantharidine analogs [3]. In this paper, we reports our endeavors along this line taking the DA reaction of tetracyclone with N-phenylmaleimide as our benchmark reaction for the construction of a lipophilic cantharidine-like model drug compound for topical application. Drug design considerations were expressed in details in a previous publication.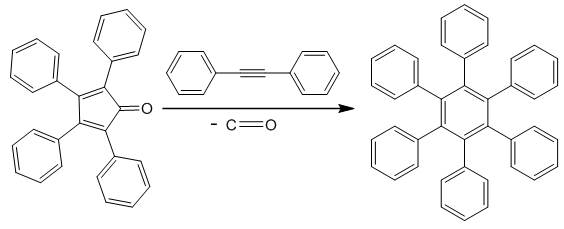 | Scheme 2. Synthesis of hexaphenylbenzene by DA reaction of tetracyclone with diphenylethylene |
Cantharidine is a vesicant toxic natural compound found in beetle insects and induces apoptosis in various cancer cell types through its inhibitory interaction with PP1 and 2A protein phosphatases [17]. In this regard, colon and rectum cancer causes about 9% lethality among all cancers. Treatment of bowel cancer using cantharidine is unthinkable due to its inherent hydrolytic instability (due to the presence of an inner carboxylic anhydride functionality) and reactivity towards nucleophiles present in the proteins found in the intestinal lumen. Moreover, as it is highly advisable that the drug remains confined with the lumen in order to avoid additional peripheral and central toxicity and in order to preclude the crossing of the intestinal barrier, a high molecular weight generally represents a good empirical predictor of poor oral biodisponibility (MW>500 daltons) and this also goes along with a high lipophilicity (logP>5). These molecular prerequisites have been successfully implemented in the title model compound with the adjunction of four phenyl groups decorating and stabilizing the anti-aromatic cyclopentadieneone template, which boosts the MW (>500 daltons) and the high lipophilicity (calculated cLogP=6.57 and experimental logP=7.0).
3. Experimental Section
Melting points were determined using an electrothermal melting point apparatus and are uncorrected. IR spectra were recorded on a Perkin-Elmer 457 spectrometer (London, UK) using vacuum-degassed anhydrous potassium bromide pellets. Wave numbers are expressed in cm−1. 1H- and 13C-NMR spectra were recorded at ambient temperature using a Brucker Spectrospin 400 spectrometer (Wissembourg, FR). Reported compounds were dissolved in CDCl3 or DMSO-d6 to obtain a 0.1 molar final solution. Chemical shifts are routinely expressed in the δ scale with TMS (tetramethylsilane) as internal standard. Thin layer chromatography (TLC) analyses were performed on Merck TLC plates (silica gel, 60 F 254, E. Merck, Darmstadt, DE, ref. 5735). For TLC, all compounds reported were insofar routinely monitored in two standard solvents, i.e. acetone/toluene/cyclohexane (solvent A, 5: 2: 3, v/v/v) and ethyl acetate/n-hexane (solvent B, 4: 6, v/v). The reverse-phase thin layer chromatography conditions were HPTLC plates RP-18 F-254 S (Merck) and methanol: water (75/25, v/v) as elution solvent. All compounds reported were found homogenous under such TLC and HPLC conditions. Specific reagents were all purchased from Aldrich (Milwaukee, WI, USA) or Acros (Geel, Belgium). All solvents respected ACS reagent grade specifications.Synthesis of TetracycloneInto a 250 ml round-bottomed flask equipped with a stirring bar, are placed 21.0g of benzil (0.1 mol), 23.1g of dibenzylketone (1,3-diphenyl-2-propanone, 0.11 mol) and 200 mL of absolute ethanol. Attach a water-cooled reflux condenser, and heat up the mixture in a hot water bath at 85°C until all solids get dissolved. Using a Pasteur pipette, add under rapid magnetic stirring and dropwise through the reflux condenser 25 mL of hot ethanolic potassium hydroxide (containing 5.7g of KOH, i.e. 0.12 mol) while keeping the water bath temperature still at 80°C, heat the solution for 15 min after which time, allow the mixture to cool down to room temperature, and then place it in an ice-water bath for 5 min. The solid product (a crystalline dark material) is isolated by vacuum filtration on a Büchner funnel, washed by small portions of ice-cold 95% ethanol, and dried overnight in a vacuum box at room temperature. Mp 219-220°C [20]. This material is chromatographically pure and its spectroscopic data are consistent with the proposed structure. 13C-NMR (CDCl3) 202.28 (C=0), 154.47 (C3, C4), 133.10 (C2, C5), 130.77 (arom. ipso carbon), 130.14 (arom. ipso carbon), 129.32, 128.47, 128.00, 127.44, 125.32 (arom. C-H carbons). UV-VIS in 0.02 M dioxane
504 (1410), 332 (7080), 259 (26000).Reaction of tetracyclone with N-phenylmaleimide.(This procedure is representative of all similar operations shown in the Table 1.)Table 1. Various conditions of the reaction of tetracyclone with N-phenylmaleimide 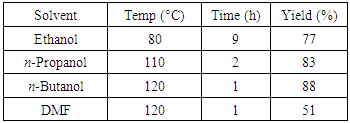 |
| |
|
Into a one-necked 250 mL round-bottomed flask equipped with a stirring bar and a reflux condenser, are placed 3.84 g of tetracyclone (10 mmol) and 1.73 g of NPM (10 mmol) in 50 mL of n-butanol. The reaction mixture is stirred and heated under reflux for 1.5 h until the deep dark violet color of tetracyclone is totally discharged. Cooling overnight in a refrigerator leaves a white-off powder. This material can be recrystallized from ethanol using a Solxhlet extractor. Yield = 4.90 (88%). The resulting white material is chromatographically pure and its spectroscopic data are consistent with the proposed structure. 13C-NMR (DMSO-d6) 195.15 (bridge-head C=0), 174. 67 (two endo imidic C=0), 141.57 (C=C), 133. 28 (arom. ipso carbon), 133.03 (arom. ipso carbon), 132.72, 130.88, 130.53, 129.79, 129.42, 128.20, 127. 87, 127.61, 127.52 (arom. C-H carbons), 65.37 (two quaternary carbons), 45.40 (two endo carbons).Reaction of tetracyclone with maleic anhydrideAn intimate mixture of 35.0 g (0.094 mole) of tetracyclone and 9.30 g (0.095 mole) of maleic anhydride is placed in a 200-ml. round-bottomed flask, and to it is added 25 mL of dry 2-dioxane. After the mixture has been refluxed gently for 8 hours, it is cooled overnight in a refrigerator, a small amount of dry diethyl ether is added to induce crystallization and a crystalline material slowly deposits. It is collected on a Büchner filter and washed with cold cyclohexane. The resulting white crystalline material is chromatographically pure and its spectroscopic data are consistent with the published structure by Allen et al. [21].Synthesis of HexaphenylbenzeneIn a test tube, 0.304 g of tetracyclone and 0.128 g of 1, 2-diphenylacetylene were dissolved in 1.0 ml diphenyl ether and heated to 220°C. After 24 h heating, the reaction was allowed to cool down to room temperature and loaded onto a short chromatography silica gel column. Elution (0 to 5% ethyl acetate in cyclohexane, followed by a single recrystallization from toluene: cyclohexane afforded the desired target molecule (392 mg, 93%). 1H-NMR (CDCl3) 6.88-6.80 (broad m, 30H); 13C-NMR (CDCl3) 140.60, 140.29, 131.40, 126.55, 125.16; IR (KBr, cm-1) 3020, 1600, 1495, 1440, 1400, 1073, 1027, 907, 782, 728, 693.
4. Conclusions
In this paper, we explored the synthesis of a cantharidine-like model compound as a probe confined within the lumen of the intestine specifically designed to treat in a prophylactic manner the familial polyposis which induces colon and rectum cancer. This venture raised many interesting chemical problems, and in particular the anti-aromatic nature of tetracyclone, the substrate opposed to N-phenylmaleimide, and the underlying consequences. Initial positive results allows for the development of a concise compound library along this axis.
ACKNOWLEDGEMENTS
The authors wish to acknowledge the generous contribution of the CTB/BTC (Coopération Technique Belge, Brussels, Belgium) both in terms of fellowship’s (U.C.K.) and financial support.
References
[1] | Barnes J. C.; Horspool W. M.; Mackie F. I. (1991)..Acta Crystallogr. C. 47: 164–168. |
[2] | Sheley, C. F.; Shechter, H. (1970). The Journal of Organic Chemistry. 35 (7): 2367–2374. |
[3] | Gbaguidi F.A.; Kasséhin, U. C.; Prevost J.R. C.; Fréderick, R.; McCurdy C.R.; Poupaert, J. H.: Journal of Chemical and Pharmaceutical Research, 2015, 7(7): 1109-1113. |
[4] | Kasséhin, U. C.; Gbaguidi, F. A.; Fréderick, R.; Lambert, D. M.; Poupaert, J. H. (submitted), 2015. |
[5] | Organic Syntheses, Coll. Vol. 5, p.1037 (1973); Vol. 46, p.107 (1966). |
[6] | Louis F. (1973). "Hexaphenylbenzene". Org. Synth. Coll. Vol., 5, p. 604. |
[7] | Kirkpatrick, P.; Ellis C. Nature, 2004, 432, 823–865. |
[8] | Leadbeater, N.; McGowan, C.C.; Fast Organic Chemistry: Microwave-assisted Laboratory Experiments; CEM Publishing: Matthews, NC, 2006. |
[9] | Liebig J. 1838– Ann., 25, p. 27. |
[10] | Biltz H. 1907- Ber., 40, p. 4806-4826. |
[11] | Biltz H. 1908- Ber., 41, p. 1379-1393. |
[12] | Diltey W. anf Quint F., J.prackt.Chem., (2), 128, 146(1930). |
[13] | Kasséhin U. C., Gbaguidi F. A., Carato P, McCurdy C.R., Accrombessi G.C., Poupaert J.H.:. American Journal of Organic Chemistry 2015, 5(5): 149-152. |
[14] | Dewar M J S; Olivella S; Stewart J J P J. Am. Chem. Soc., 1986, 108 (19): 5771–577. |
[15] | SIDS Initial Assessment Report, Geneva: United Nations Environment Programme, April 2005. |
[16] | Organic Syntheses, Coll. Vol. 3, p.807 (1955); Vol. 23, p.93 (1943). |
[17] | Yayha M. ; Fatemeh G. ; Abdolhesein D. ; Zohreh S. ; Zuhair H. Research Journal of Parasitology ISSN 1816-4943. |
[18] | Moed L.; Shwayder T.A.; Chang M.W. Arch Dermatol. 2001; 137(10):1357-1360. |
[19] | Dorn D.C.; Kou C.A.; Png K.J.; Moore M.A.S. Int. J. Cancer. 2009, 124: 2186–2199. |
[20] | Johnson, J. R.; Grummitt, O.; Org. Synth. 1943, 23, 92. 805. |
[21] | Allen C.F.H., Chem. Revs., 37, 209 (1945):62,653(1962). |