Abraham González-González 1, David M. Aparicio-Solano 2, Hidemí Aguilar-Mariscal 3, Abraham Gómez-Rivera 1, Luis F. Roa 1, Cuauhtémoc Alvarado-Sánchez 1, Cárlos E. Lobato-García 1, Nancy Romero-Ceronio 1
1División Académica de Ciencias Básicas, Universidad Juárez Autónoma de Tabasco, Tabasco, México
2Centro de Química, Instituto de Ciencias, Benemérita Universidad Autónoma de Puebla, Pue, México
3Laboratorio de Farmacología, Unidad de Producción, Cuidado y Experimentación de Animales, División Académica de Ciencias de la Salud, Universidad Juárez Autónoma de Tabasco, Tabasco, México
Correspondence to: Nancy Romero-Ceronio , División Académica de Ciencias Básicas, Universidad Juárez Autónoma de Tabasco, Tabasco, México.
Email: |  |
Copyright © 2016 Scientific & Academic Publishing. All Rights Reserved.
This work is licensed under the Creative Commons Attribution International License (CC BY).
http://creativecommons.org/licenses/by/4.0/

Abstract
Coumarins are versatile organic compounds that possess multiple biological properties. Recently, their synthesis and biological activities have received a great deal of interest. We report the synthesis of four coumarins derived from the Knoevenagel condensation of o-vanillin aldehyde and dimethyl or diethyl malonate and further transesterification. The condensation was performed by a one pot procedure, and the yield reached 96-97%. Meanwhile, the transesterification procedure took place in two steps with a 60% yield. We also explored the anti-inflammatory activity of one of the derivatives by using the carrageenan-induced rat paw edema.
Keywords:
Coumarin, Knoevenagel condensation, Anti-inflammatory activity
Cite this paper: Abraham González-González , David M. Aparicio-Solano , Hidemí Aguilar-Mariscal , Abraham Gómez-Rivera , Luis F. Roa , Cuauhtémoc Alvarado-Sánchez , Cárlos E. Lobato-García , Nancy Romero-Ceronio , Synthesis of 3-carboxylated Coumarins by Knoevenagel Condensation and Exploratory Anti-inflammatory Activity Evaluation by in vivo model, American Journal of Organic Chemistry, Vol. 6 No. 1, 2016, pp. 17-28. doi: 10.5923/j.ajoc.20160601.03.
1. Introduction
Coumarins are a class of organic compounds with a wide distribution in nature. There are a number of reports concerning to their biological properties as anti-inflammatory, antitumor and antibacterial among others. [1] There are several diseases that elicit inflammation. This is a natural response of the organism to an external aggression that can be originated by mechanical injuries or caused by infectious diseases. This biological process includes the formation of various mediators, which help the organism to recover homeostasis by fighting microorganisms and repairing damaged cells. Between these cell mediators, we could highlight the enzymes cyclooxygenase-1 and cyclooxygenase-2 since they are directly involved in the inflammation mechanism; therefore, if they are blocked, the inflammation process could be interrupted. This is important since different types of anti-inflammatory drugs, are capable to block this enzymes. [2] Several studies have shown that functionalization of the coumarin structure could enhance the biological activities. [3] Coumarins could be an attractive alternative as anti-inflammatory drugs, [3, 4] they can be classically synthesized by the Perkin, Pechmann or Knoevenagel reactions. Recently, the Wittig, the Kostanecki–Robinson and the Reformatsky reactions have also been reported as useful synthetic strategies for coumarin derivatives. [1, 5] Here, we present a new neat methodology under ultrasound irradiation for the synthesis of 4 previously reported coumarin derivatives, via the Knoevenagel condensation between o-vanillin aldehyde and dimethyl or diethyl malonate followed by a transesterification process. The reaction products were structurally confirmed by analytical techniques. We also report our first approach on the anti-inflammatory study of this group of compounds, by presenting the protective anti-inflammatory effect of compound (1b), see scheme 1.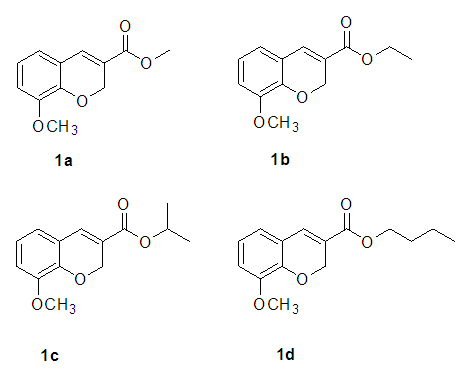 | Scheme 1. 3-carboxylated coumarins |
2. Experimental
2.1. Materials and Equipment
All reagents and solvents were of chemical grade and purchased from Sigma Aldrich. They were used without further purification. Melting points were measured on a Fisher-Jones apparatus and left uncorrected. The structural characterization was done by High Resolution Mass Spectrometry (HRMS), Infrared Spectroscopy (IR) and1H and 13C NM. Mass Spectra were recorded in a Thermo Fischer Scientific LQT-Orbitrap ESI; Infrared spectra (IR) were recorded on a Perkin-Elmer Spectrum One FTMS spectrometer. NMR spectra were recorded on a Varian VX-400 spectrometer with tetramethylsilane (TMS) as an internal standard, and chemical shifts are reported in δ (ppm); spectroscopic data are provided as supplementary material. Thin-layer chromatography (TLC) was performed on precoated silica gel F254 plates. Detection was by iodine vapor staining and UV light irradiation (UV lamp, model UV-IIB). Column chromatography was carried out with silica gel H (200-300 mesh or 500 mesh). Organic solutions were dried over anhydrous sodium sulfate.
2.2. General Procedure for the Synthesis of 1a and 1b
In a round-bottom flask, were placed one equivalent of o-vanillin Alde Hyde and 1.2 equivalents of diethyl or diethyl malamute. Then 0.35 equivalents of piper dine acetate and 0.10 equivalents of lithium sulfate were added. The mixture reaction was placed in an ultrasonic bath at 50°C for 15 minutes. The completion of the reaction was monitored by TLC using EtOAc/petroleum ether (1:8) as eluent. After the reaction was completed, the mixture was extracted with EtOAc (3 x 15 mL). The organic phase was separated and dried over anhydrous sodium sulfate and rotary evaporated to dryness with a vacuum pump. The products were purified by crystallization (ethyl acetate / hexane, 1:1). In both reactions a crystalline solid was obtained (97% and 96% yield respectively).Methyl-8-methoxy-2-oxo-2H-chromene-3-carboxylate. 1a. Yellow solid (97% yield), mp 142 °C. MS (m/z: 234.02, M+); HRMS (m/z: 235.0359, M+1); IR (1729, 1609 and 1574 cm-1). 1H NMR (400 MHz, CDCl3) δ = 3.95 (s, 3H-1), 3.97 (s, 3H-11), 7.20 (m, 3H-6,7,8), 8.50 (s, 1H-4). 13C NMR (100 MHz, CDCl3) δ = 52.76, 56.15, 115.77, 117.83, 118.23, 120.52, 124.67, 144.67, 146.86, 149.31, 156.04, 163.58.Ethyl-8-methoxy-2-oxo-2H-chromene-3-carboxylate. 1b. White solid (96% yield), mp 82 °C. MS (m/z: 248.04, M+) HRMS (m/z: 249.0492 M+1); IR (1761, 1609 and 1574 cm-1). 1H NMR (400 MHz, CDCl3) δ = 1.41 (t, J=4.0 Hz, 3H-1), 3.97 (s, 3H-10), 4.41 (q, J=4.0 Hz, 2H-2), 7.18 (m, 1H-9), 7.19 (m, 1H-7), 7.26 (dd, J=6.0, 6.7Hz, 1H-8), 8.50 (s, 1H-5). 13C NMR (100 MHz, CDCl3) δ = 14.42, 56.48, 61.40, 115.68, 118.30, 120.43, 124.62, 144.69, 146.91, 148.73, 156.06, 162.95.
2.3. General Procedure for the Synthesis of 1c and 1d
In a round-bottom flask, one equivalent of 1b was dissolved in methylene chloride and 5 eq of sodium hydroxide solution (10%) was added. The reaction was placed in an ultrasonic bath at 50 °C for 15 minutes. The hydrolysis of the ester was followed by thin layer chromatography (TLC). Once completed, the reaction mixture was washed (3 x 15 mL of brine) in a separation funnel and the organic phase was separated, dried over anhydrous sodium sulfate, and then taken to dryness using a rotary evaporator and a vacuum pump. Subsequently, 6 equivalents of thionyl chloride and 4 equivalents of isopropanol or n-butanol were added. This reaction mixture was set to reflux for 4 hours. The reaction was monitored by TLC. After completed the reaction, the crude product was purified by column chromatography (SiO2, 70/30 hexane-ethyl acetate) to afford the corresponding 3-substituted coumarin. In both reactions, a crystalline solid was obtained (65% and 61% yield respectively).Isopropyl-8-methoxy-2-oxo-2H-chromene-3-carboxylate. 1c. Light yellow solid (65% yield), mp 120 °C. MS (m/z: 262.06, M+) HRMS (m/z: 263.0642, M+1); IR (1758, 1610 and 1574 cm-1). 1H NMR (400 MHz, CDCl3) δ = 1.39 (d, J=8.0 Hz, 6H-1,2), 3.97 (s, 3H-12), 5.26 (m, 1H-3), 7.25 (m, 3H-8,9,10), 8.44 (s, 1H-6). 13C NMR (100 MHz, CDCl3) δ = 21.73, 56.21, 69.56, 115.71, 118.36, 118.75, 120.51, 124.64, 144.69, 146.95, 148.17, 156.13, 162.22. Butyl-8-methoxy-2-oxo-2H-chromene-3-carboxylate. 1d. Yellow solid (61% yield), mp 72 °C. MS (m/z: 276.07, M+) HRMS (m/z: 277.0791, M+1); IR (1740, 1695, 1609 and 1574 cm-1).1H NMR (400 MHz, CDCl3) δ = 0.98 (t, J=4.0 y 8.0 Hz, 3H-1), 1.47 (m, 2H-2), 1.76 (m, 2H-3), 3.97 (s, 3H-13), 4.35 (t, J= 4.0 Hz, 2H-4), 7.25 (m, 3H-9,10,11), 8.48 (s, 1H-7). 13C NMR (100 MHz, CDCl3) δ = 13.70, 19.11, 30.56, 56.27, 65.78, 115.73, 118.42, 118.55, 120.55, 124.63, 144.83, 148.65, 156.09, 163.16.
2.4. Anti-inflammatory Evaluation of 1b
A preliminary exploration of the anti-inflammatory effect of compound 1b, was assayed by the carrageenan induced paw edema in rat. [6] Four sets of 6 female Wistar rats (150-200 g) were arranged. The animals were provided by the Unidad de producción Cuidado y Experimentación de Animales, División Académica de Ciencias de la Salud, Universidad Juárez Autónoma de Tabasco, México. The rats were kept fasted for 12 hours before the experiment. Compound 1b was suspended in dimethyl sulfoxide and prepared at doses for 50, 100 and 150 mg/kg to be orally administered to each set of rats. A set of 6 animals was treated as control group.After the administration of the correspondent dose of 1b, edema was induced in the right hind paw by intradermal injection of 0.1 mL of a 3% suspension of carrageenan in water. Prior to this, the paws were measured by a Hugo Basille digital pletismometer, then measurements were made every hour during six hours of the experiment. Throughout this process, the animals had free access to water. For each animal the maximum anti-inflammatory protective effect was measured by using equation (1):  | (1) |
Where: Vcarr = volume of the rear paw of the rat which received carrageenan i.pl.; Vtreat = volume of the rear paw of the rat who received the coumarin 1b prior the application of carrageenan and Vo = volume of the rear paw of the rat at zero time. The results of the maximum protective effect were performed on Sigma Plot 12.0. [2]
3. Results and Discussion
3.1. Synthesis of Coumarin Derivates
Scheme 2 presents a resume of the methodology employed in the synthesis of compounds 1a and 1b.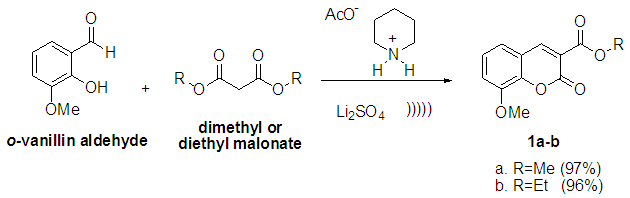 | Scheme 2. Synthesis of coumarin derivatives 1a and 1b |
In order to establish the best reaction conditions, we performed a preliminary optimization study. Previous reports employed piperidine as a base, but when we applied this strategy, the results were not quite satisfactory (50% yield). [7] To overcome this drawback, we instead used piperidine acetate which promoted better yields. We also modified the amount of lithium sulfate, piperidine acetate and reaction time as shown in table 1. The best results were obtained with the use of 10% mol of LiSO4 (entry 3), whereas in the absence of LiSO4, the reaction proceeded in very low yields (entry 1). Additionally, diethyl malonate was employed instead of dimethyl malonate and compound 1b was obtained with similar yields (entry 6).Table 1. Synthesis results of coumarin derivatives 1a-1b 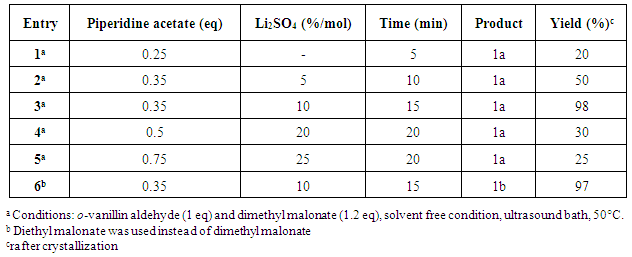 |
| |
|
The attempt to perform the direct condensation without any catalyst was not satisfactory since we obtained only a 20% yield (entry 1). An explanation for this may be the in situ water formation because of the condensation process. The efficiency of the reaction was improved by using 10 percent of lithium sulfate. It is well known that lithium has an affinity for the oxygen of carbonyl compunds and hence it is possible to consider a coordination between lithium and the oxygen of the o-vanillin reagent, which may increment the electrophilicity of the carbonyl group; besides that, it is also possible that the anion sulfate could scavenge water molecules, forcing the reaction to completion.The derivatives 1c and 1d, were obtained starting from the derivative 1b through a two-step process. Scheme 3 describes the reactions.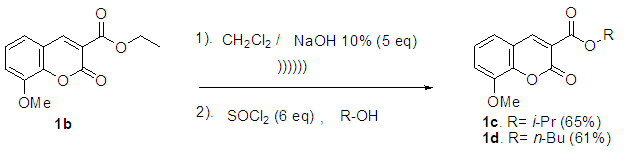 | Scheme 3. Synthesis of derivatives 3c and 3d |
This strategy proved to be satisfactory to obtain our target molecules.The spectroscopic data are in good agreement with those previously reported. [7-8] For instance, the X-Ray analysis of the crystalline structure for 1b was compared with the data provided in the literature, as summarized in table 2. [9]Table 2. Crystal data comparison of 1b 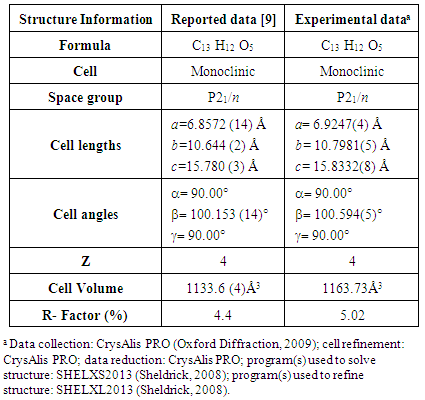 |
| |
|
3.2. Anti-inflammatory Activity Evaluation
Using the model of carrageenan induced rat paw edema assessed the in vivo evaluation of the anti-inflammatory effect of 1b and the results are summarized in Figure 1.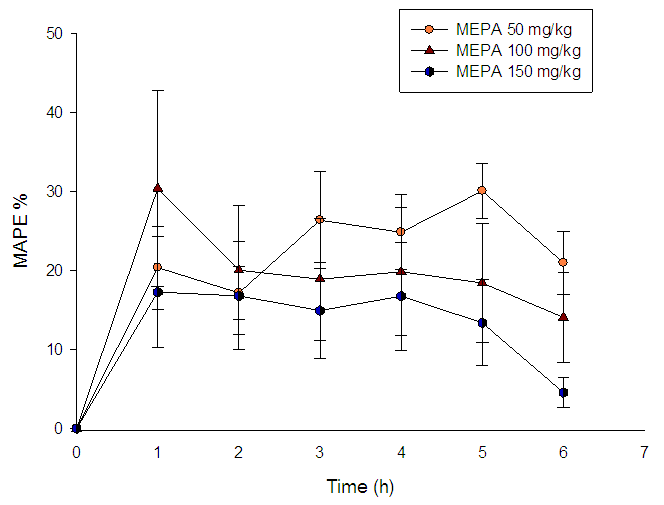 | Figure 1. Time course of the maximum anti-inflammatory protective effect of 18b compound at doses of 50, 100 and 150 mg/kg. Each point represents average ± standard error (n=6) |
At a dose of 50mg/kg, it is possible to see a moderated protective effect at the first hour after the administration, which had a positive trend up to the fifth hour in which it started decreasing. On the other hand, the protective effect of compound 1b was barely above 30% after the first hour of the administration of a 100 mg/kg dose and it remained steady over the rest of the treatment. Finally, when a dose of 150 mg/kg of compound 1b was administered, the protective effect was slightly lower when compared to the other doses, and in general had a similar steady tendency. These results are encouraging and motivate us for future research in order to get a deeper insight in the biological activities of the coumarin moiety.
4. Conclusions
We synthesized four coumarin derivatives via a Knoevenagel condensation. The process involved solvent-free conditions, the use of lithium sulfate as a catalyst and ultrasound was employed as activation source. In addition, we implemented a procedure to modify the alkoxycarbonyl chain in two steps through the in situ transesterification of the corresponding acyl chloride. The preliminary evaluation of the anti-inflammatory protective effect of one of the compounds confirms the potential of the coumarin moiety as anti-inflammatory agent and leads us to the design of further studies in order to ascertain how structural differences affect anti-inflammatory effectiveness.
ACKNOWLEDGEMENTS
The authors thank the “Programa de Redes Temáticas del CONACYT” for financial support via the project “Red de investigación en Organocatálisis Asimétrica”, 252435. Abraham González-González is grateful to CONACyT for a Scholarship (No. 558296).
Suplementary Data
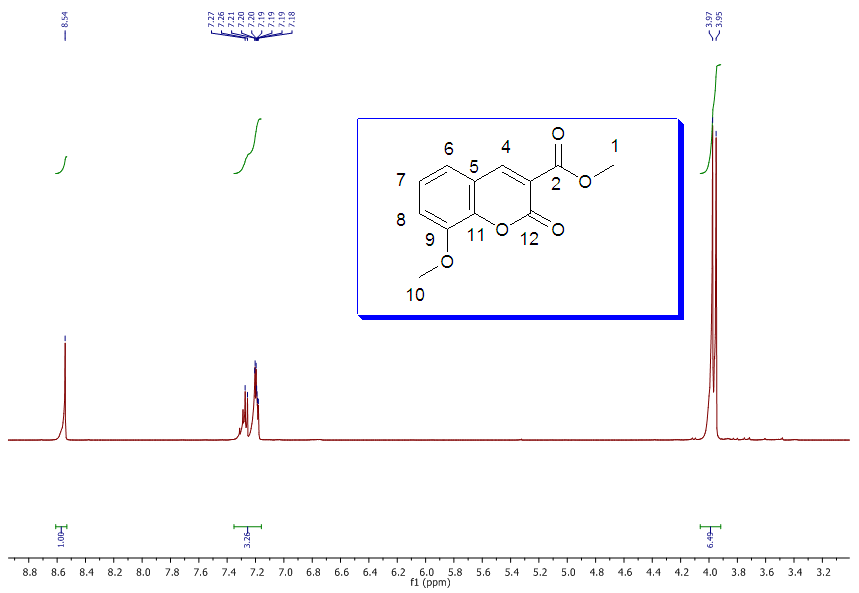 | 1H NMR Spectrum for compound 1a. (400 MHz, CDCl3) |
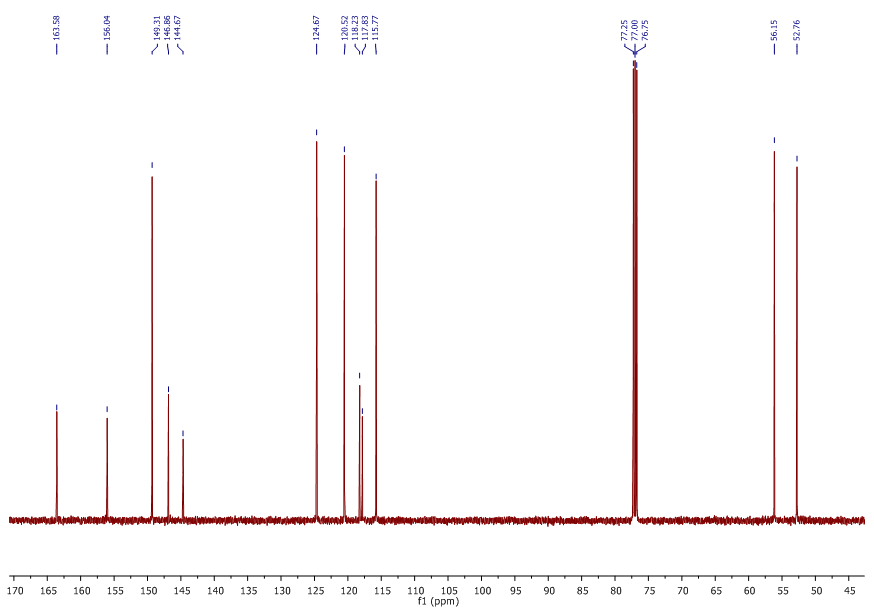 | 13C NMR Spectrum for compound 1a. (100 MHz, CDCl3) |
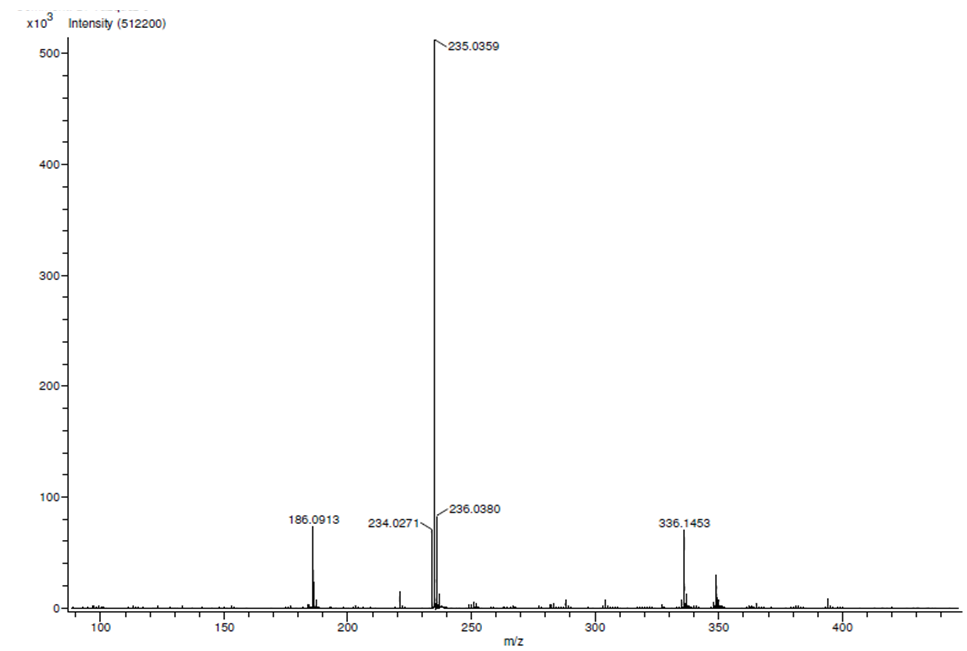 | HRMS for compound 1a. |
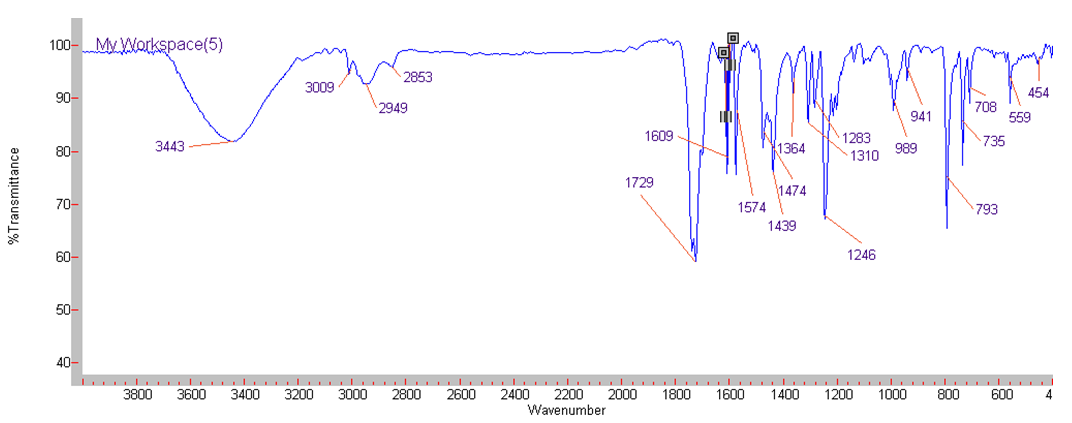 | IR Spectrum for compound 1a. (KBr) |
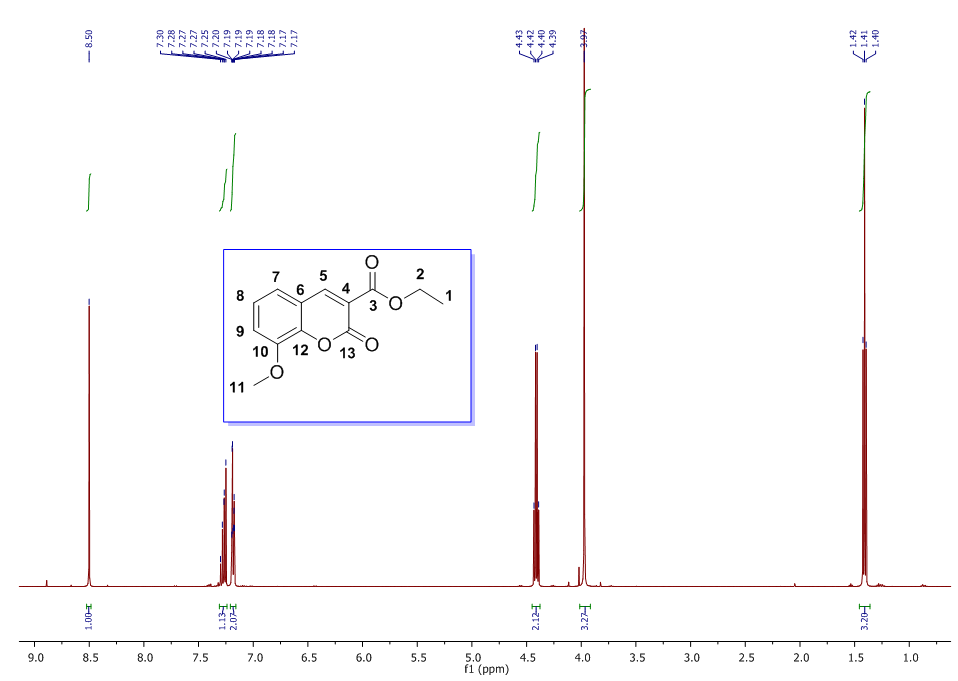 | 1H NMR Spectrum for compound 1b. (400 MHz, CDCl3) |
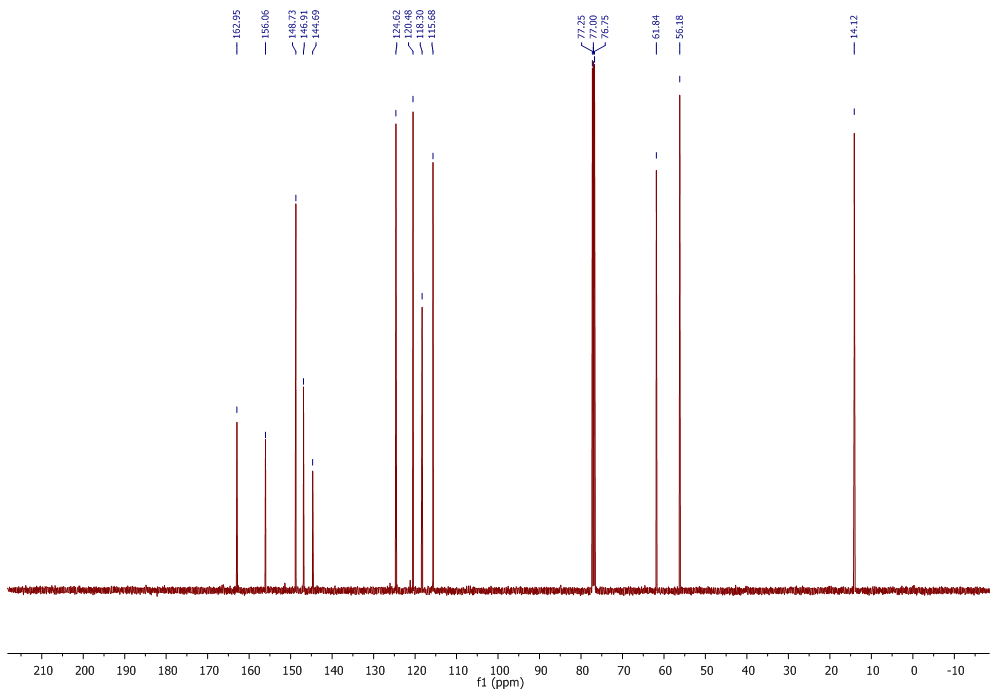 | 13C NMR Spectrum for compound 1b. (100 MHz, CDCl3) |
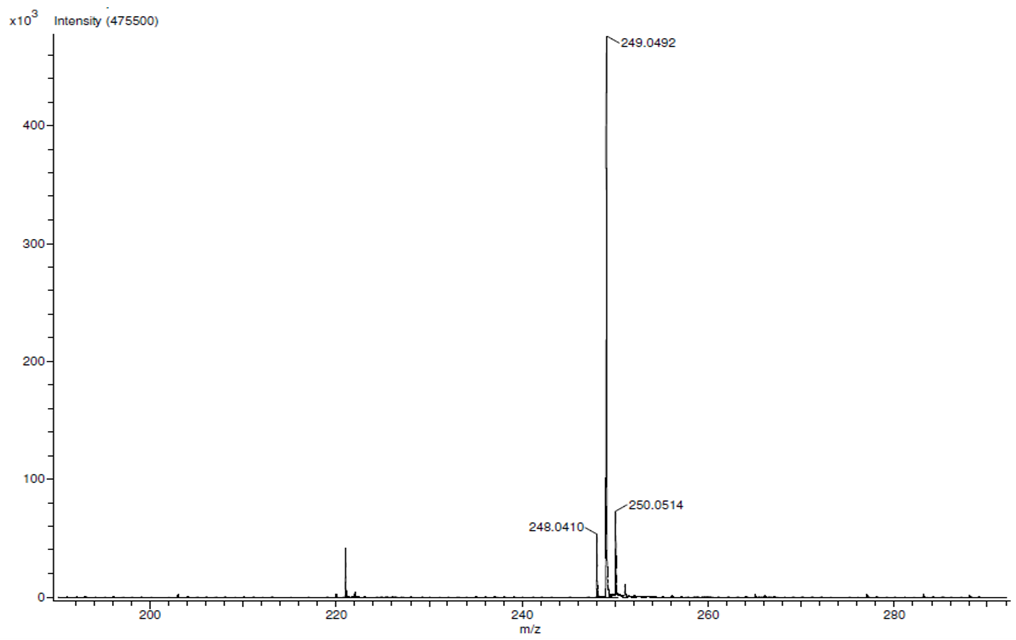 | HRMS for compound 1b. |
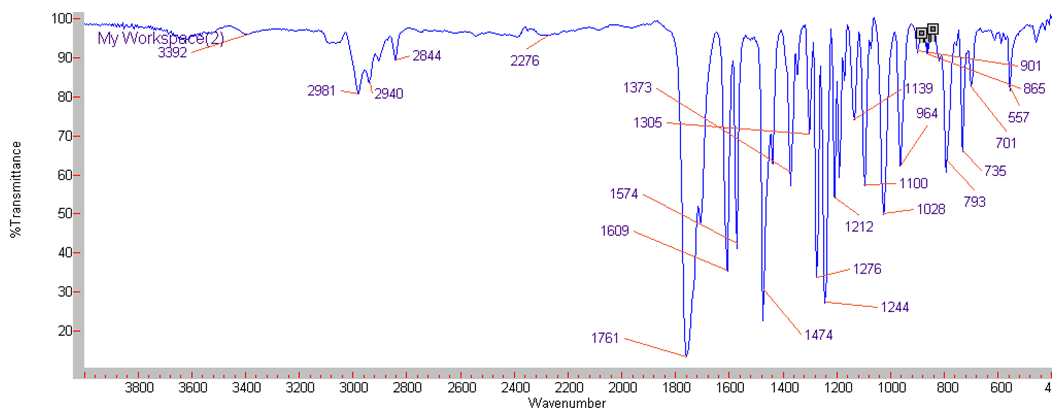 | IR Spectrum for compound 1b. (KBr) |
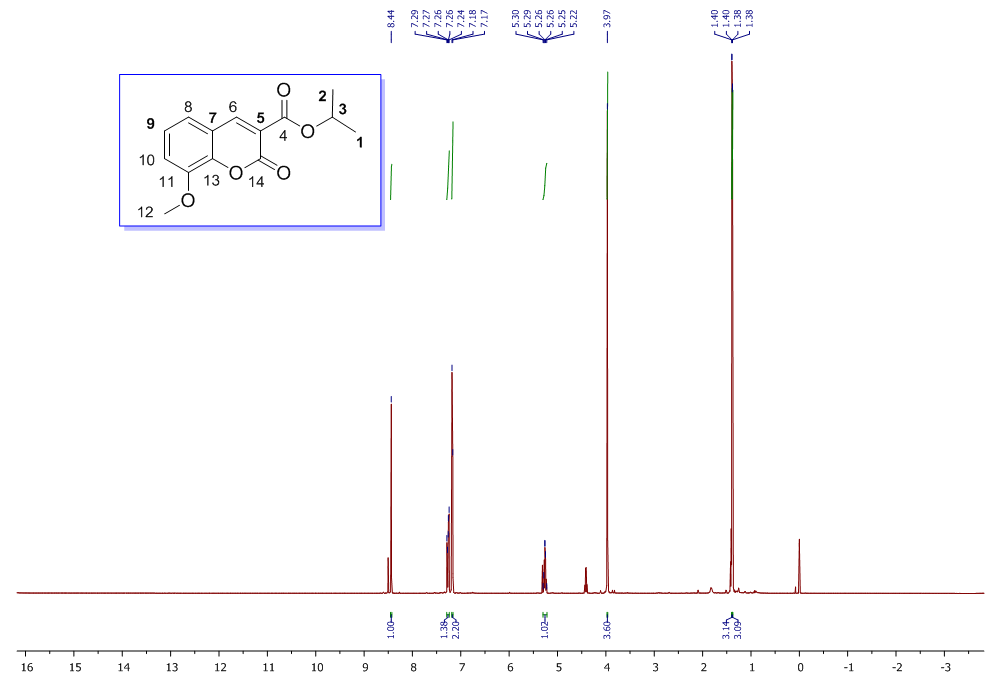 | 1H NMR Spectrum for compound 1c. (400 MHz, CDCl3) |
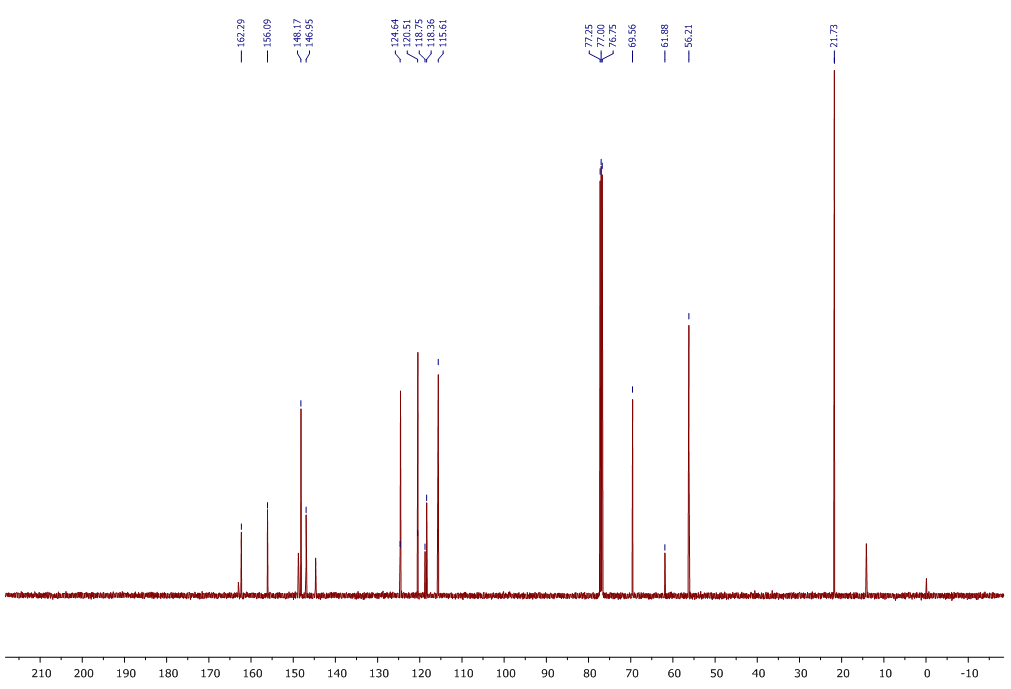 | 13C NMR Spectrum for compound 1c. (100 MHz, CDCl3) |
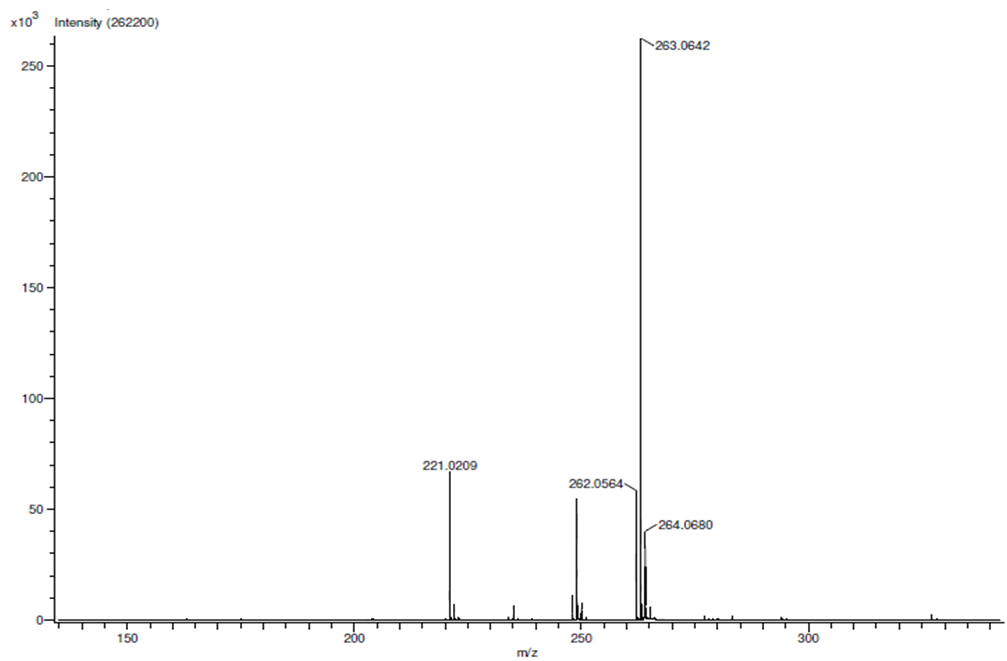 | HRMS for compound 1c |
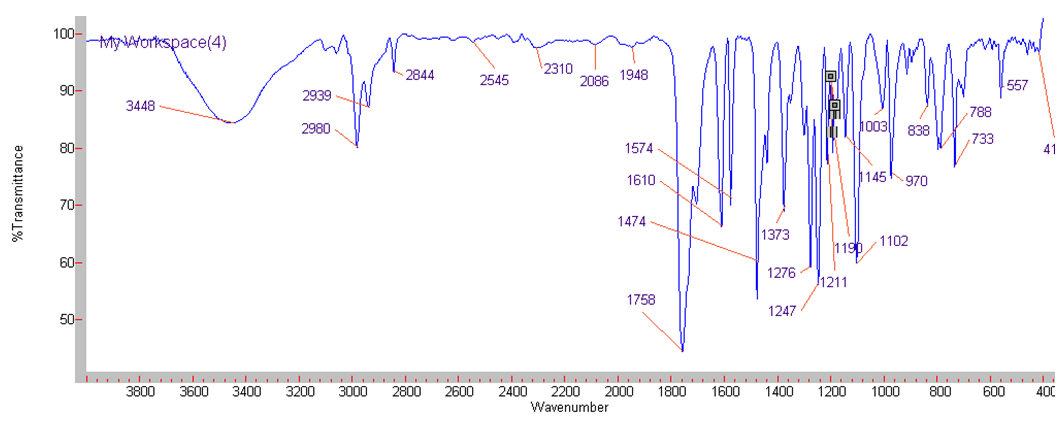 | IR Spectrum for compound 1c. (KBr) |
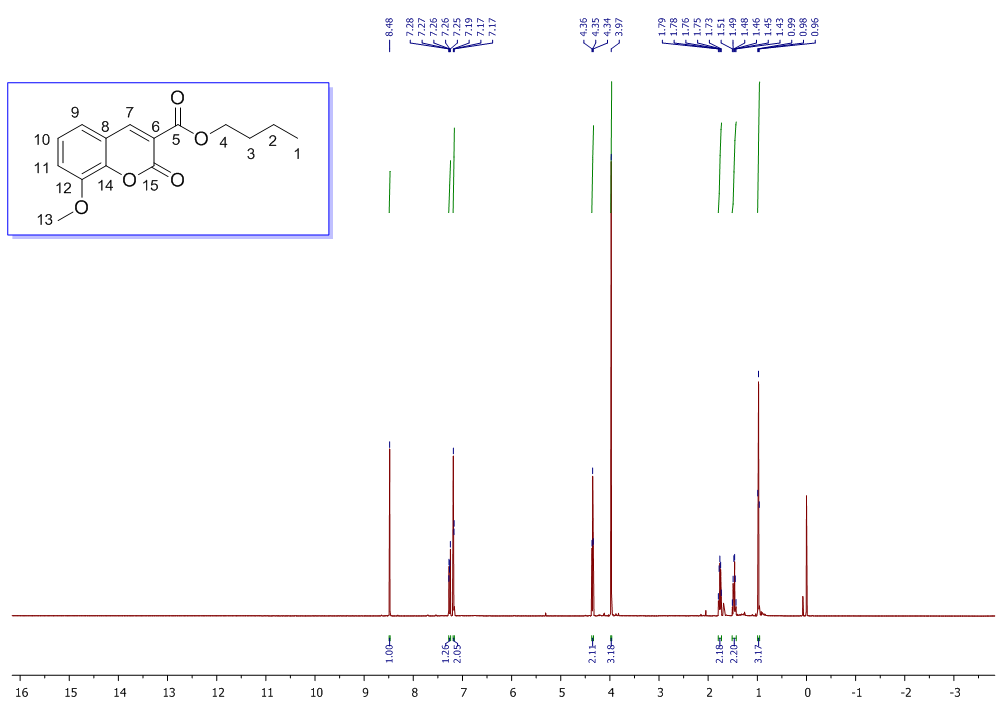 | 1H NMR Spectrum for compound 1d. (400 MHz, CDCl3) |
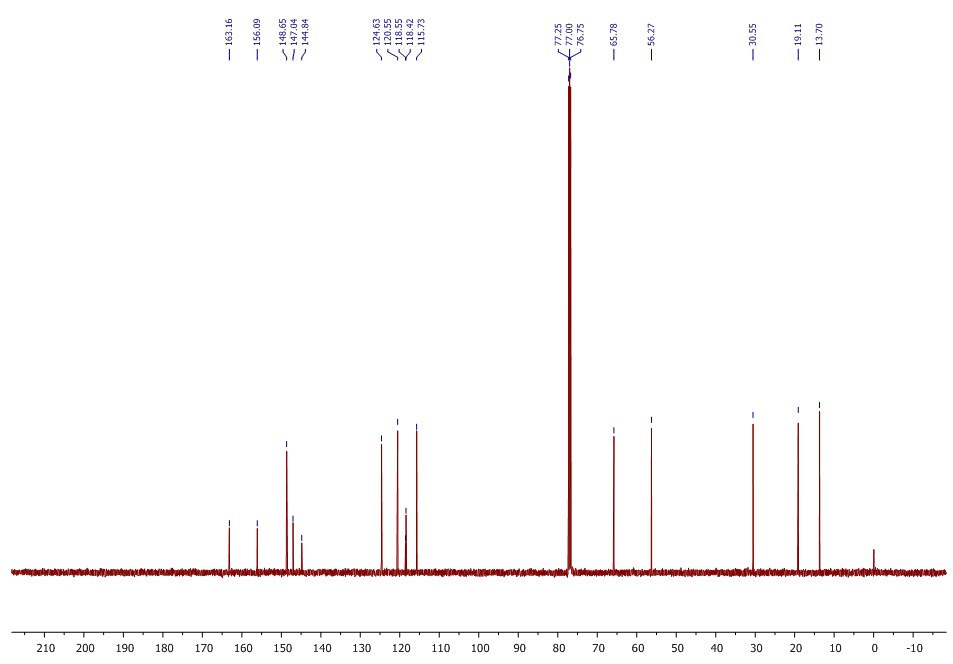 | 13C NMR Spectrum for compound 1d. (100 MHz, CDCl3) |
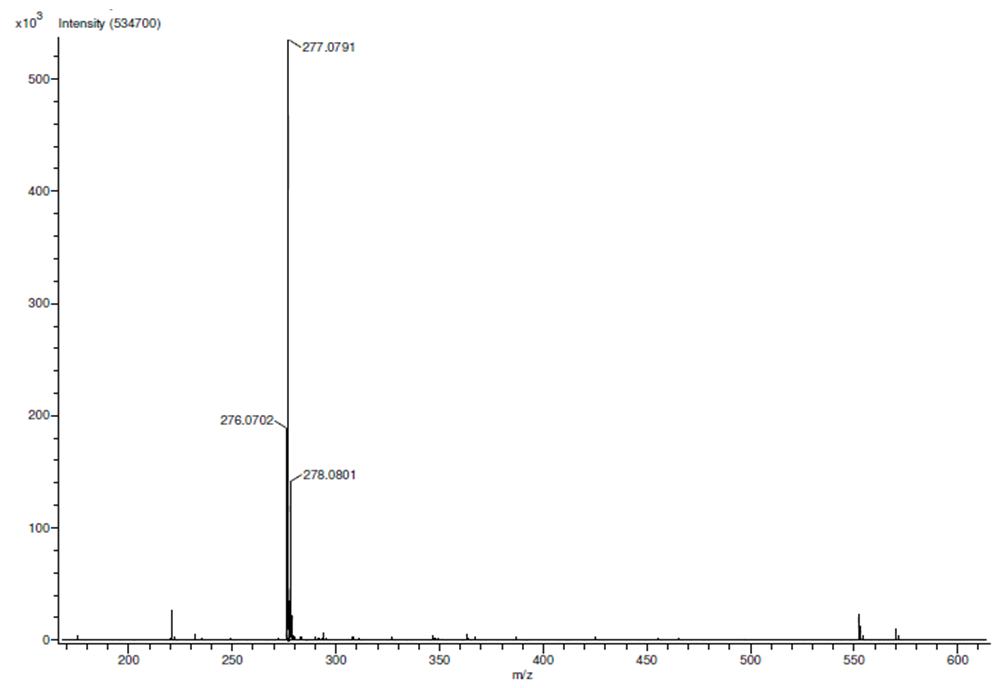 | HRMS for compound 1d |
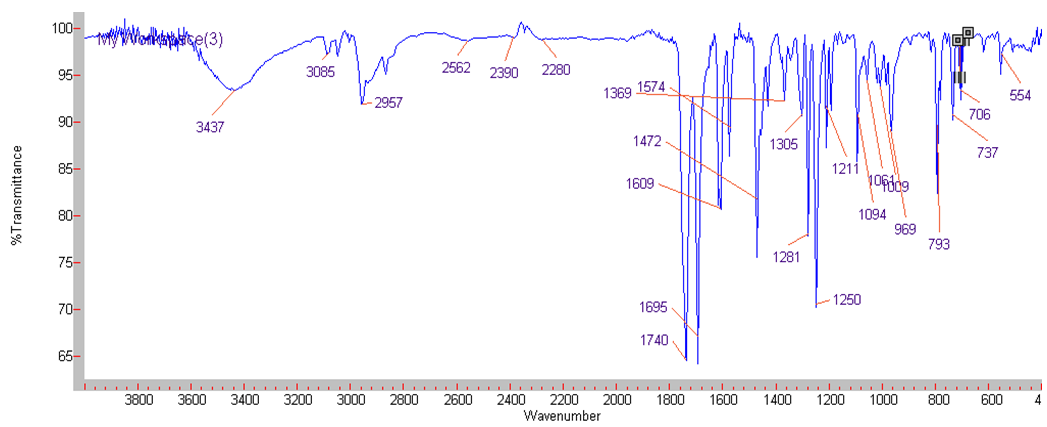 | IR Spectrum for compound 1d. (KBr) |
References
[1] | Borges, F.; Roleira, F.; Milhazes, N.; Santana, L. and Uriarte. E., Simple Coumarins and Analogues in Medicinal Chemistry: Occurrence, Synthesis and Biological Activity, Current Medicinal Chemistry, 12 (8), 887-916. (2005). |
[2] | Gómez-Rivera, A.; Aguilar-Mariscal, H.; Romero-Ceronio, N.; Roa, L., F.; Lobato-García, C., E., Synthesis and anti-inflammatory activity of three nitro chalcones, Bioorganic & Medicinal Chemistry Letters, 23, 5519–5522. (2013). |
[3] | Kontogiorgis, C., A.; Sawoglou, K. and Hadjipavlou-Litina, D. Antiinflaininatory and antioxidant evaluation of novel coumarin derivatives, Journal of Enzyme Inhibition and Medicinal Chemis, 21(1): 21-29. (2006). |
[4] | Kulkarni. M. V.; Kulkarni, G. M.; Lin, C. and Sun C., Recent Advances in Coumarins and 1-Azacoumarins as Versatile Biodynamic Agents, Current Medicinal Chemistry, 13, 2795-2818. (2006). |
[5] | Valizadeh, H. and Vaghefi. S, One-Pot Wittig and Knoevenagel Reactions in Ionic Liquid as Convenient Methods for the Synthesis of Coumarin Derivatives, Synthetic Communications, 39, 1666–1678. (2009). |
[6] | González. M., C.; Ospina, L., F. and Velandia, J., R., Actividad Antiinflamatoria de Extractos y Fracciones de Myrcianthes leucoxila, Calea prunifolia, Curatella americana y Physalis peruviana en los Modelos Edema Auricular por TPA, Edema Plantar por Carragenina y Artritis Inducida por Colágeno, Biosalud, 10 (1), 9 – 18. (2011). |
[7] | Sugino, T. and Tanaka, K., Solvent-Free Coumarin Synthesis, Chemistry Letters, 30 (2), 110-111. (2001). |
[8] | Ramani, A.; Chandra, B., M.; Velu, S. and Sivasanker, S., One-pot synthesis of coumarins. Catalysis by the solid base, calcined Mg-Al hydrotalcite, Green Chem., 1 (3), 163-165. (1999). |
[9] | Takahashi, H.; Takechi, H.; Kubob, K. and Matsumoto, T., Ethyl 8-methoxy-2-oxo-2H-1-benzopyran-3-carboxylate, Acta Crystallographica Section E, E62, o2553–o2555. (2006). |