Yoshimitsu Kumai 1, Ryuta Miyatake 2, Yuka Sugeno 1, Akira Ohta 1, Mitsunori Oda 1
1Department of Chemistry, Faculty of Science, Shinshu University, Matsumoto, Nagano, Japan
2Centre of Environmental Conservation and Research Safety, University of Toyama, Toyama, Japan
Correspondence to: Mitsunori Oda , Department of Chemistry, Faculty of Science, Shinshu University, Matsumoto, Nagano, Japan.
Email: |  |
Copyright © 2015 Scientific & Academic Publishing. All Rights Reserved.
Abstract
The title compound 9 was synthesized by condensation of 2,2’-biindole and 1-chloro-3-(N,N-dimethylamino) propenium chloride in 1,2-dichloroethane. NMR and UV-vis absorption spectra of 9 under neutral, acidic and basic conditions were discussed in respect of its protonation and deprotonation. A crystal structure of its protonated species, 9H+TfO–, was determined by X-ray diffraction analysis, indicating its planar and symmetrical structure. Change of absorption spectra of 9 in the presence of a large excess of various metal ions was studied to find out a mode of interaction between 9 and metal ion.
Keywords:
Biindole, Azaazulene, Azepine, X-ray structure, Absorption spectra, DFT calculations
Cite this paper: Yoshimitsu Kumai , Ryuta Miyatake , Yuka Sugeno , Akira Ohta , Mitsunori Oda , Synthesis and Spectroscopic Properties of 1H-Cyclohepta[2,1-b:3,4-b’]diindole and Molecular Structure of its Protonated Species, American Journal of Organic Chemistry, Vol. 5 No. 1, 2015, pp. 10-13. doi: 10.5923/j.ajoc.20150501.02.
1. Introduction
There were reported two marine natural products having two indole units around a fully unsaturated seven-membered ring, iheyamine A (1) [1] and cauresin (2) [2–5], the former of which exhibits cytotoxic activity against tumor cells. [1] In respect to their unique structures and biological activity, various compounds (3–8) structurally related to 1 and 2 have been synthesized (Figure 1). [3–6] 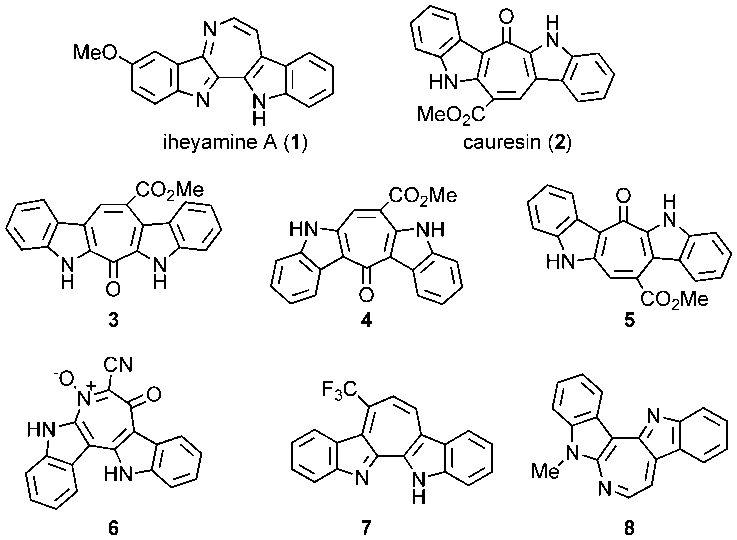 | Figure 1. Marine natural products (1–2) having two indole units and structurally related synthetic compounds (3–8) |
However, basic structural features and spectroscopic properties of a simple compound without any substituent for these
systems have not been reported yet. Herein we describe synthesis of the title compound 9, 1H-cyclohepta[2,1-b:3,4-b’]diindole, which is the parent compound for 7, its absorption properties under various conditions, and also X-ray structure of its trifluoromethane- sulfonic acid (TfOH) salt.
2. Results and Discussion
2.1. Synthesis and properties of 1H-cyclohepta- [2,1-b:3,4-b’]diindole (9)
The title compound 9 was synthesized, in a similar way of Baraznenok et al., [6] by condensation of 2,2’-biindole (10) [9] with 1-chloro-3-(N,N-dimethylamino)propenium chloride (11), which was generated in situ by reaction of 3-dimethylaminoacrolein with oxalyl chloride (Scheme 1) [10].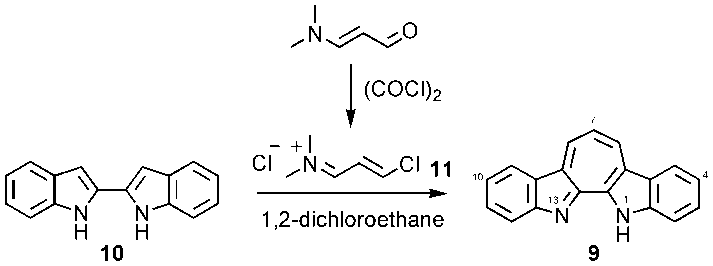 | Scheme 1. Synthesis of 9 |
The compound 9 was isolated as dark red plates in 57 % yield. The structure of 9 was confirmed by spectroscopic analysis and the results of X-ray crystallographic diffraction of its protonated compound (vide infra). Seven signals in the 1H-NMR spectrum of 9 and ten signals in the 13C-NMR indicate its symmetrical structure around the central molecular axis through the 7 carbon atom, concomitantly evidencing a faster hydrogen shift between two tautomeric structures than the NMR time scale. It is worthy to note that there is a relatively large difference of proton chemical shifts between the 6(8) and 7 positions
as seen in azulenes and azaazulenes, which have alternatively fluctuated
density around their peripheral ring frameworks [11, 12].The UV-vis spectrum of 9 in CH3CN exhibits mainly two absorption bands, strong band around 330 nm and weak band at 495 nm (Figure 2). In an acidic medium of trifluoroacetic acid (TFA), the long wavelength absorption exhibits a blue shift by 35 nm and color of the solution turns to yellow orange. On the other hand, in a basic medium of 16%. 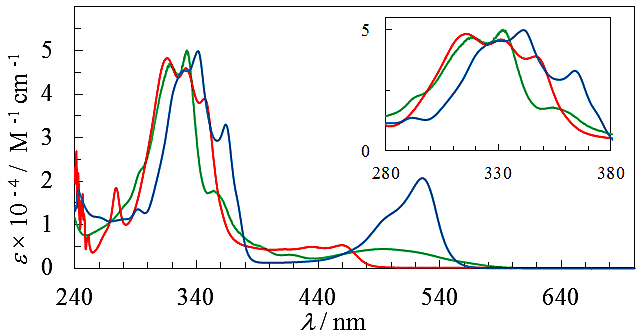 | Figure 2. Absorption spectra of 9 in CH3CN (green), TFA (red), and NaOH aq./DMSO (blue). Inset is expanded spectra at a range of 280–380 nm |
NaOH aq. in DMSO, both absorption bands show a red shift. While the shift value of the short wavelength absorption is small as 9 nm, that of the long wavelength absorption is 31 nm. Color of the basic solution is bright red, which is visibly distinguishable from those of the neutral (dark red) and acidic (yellow orange) solutions. The color changes under acidic and basic conditions can be attributed to the protonation and deprotonation of 9 to generate ionic species, 9H+ and 12 (Scheme 2), respectively. Formation of these ionic species was confirmed by 1H NMR data in TFA-d1 and NaOD/DMSO-d6; [13] i.e., the hydrogens of 9H+ (
8.25 ppm) are deshielded and those of 12 (
7.91 ppm) slightly shielded compared with that of 9 (
7.93 ppm) in CDCl3.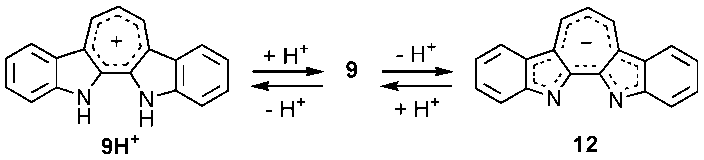 | Scheme 2. Protonation and deprotonation of 9 |
The crystal structure of a TfOH salt of 9 was determined by X-ray crystallographic analysis. There are two independent molecules of 9H+ with slightly different bond lengths and angles in a cell. ORTEP drawings of one of the structures are shown in Figure 3. The structure of 9H+ is planar and almost symmetrical around a central molecular axis through the C7 carbon atom. Average bond lengths of the two different molecules of 9H+ and its calculated structure at the B3LYP/6-31G(d) level of theory [14] are shown in Figure 4. Difference between them is less than 0.017 Å, indicating that the results of calculations at the level of theory are reliable. Since difference of bond lengths of the seven-membered ring in the calculated structure of 9H+ is less than 0.046 Å and average of their bond lengths is 1.410 Å, the ring indicates bond-convergency, besides the benzene rings. On the other hand, the calculated structure of 9 has maximum difference of bond lengths of the seven-membered ring is 0.087 Å, indicating slight bond- alternation around the seven-membered ring.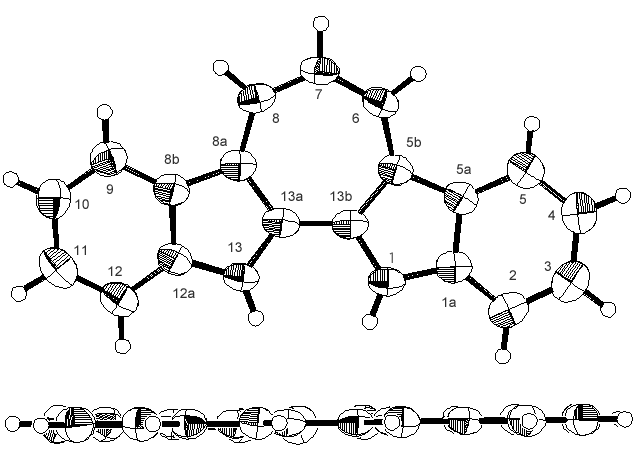 | Figure 3. ORTEP drawings of 9H+TfO–, one of two different molecules. Top views (top) and side view (bottom). The counter anion (TfO–) was omitted |
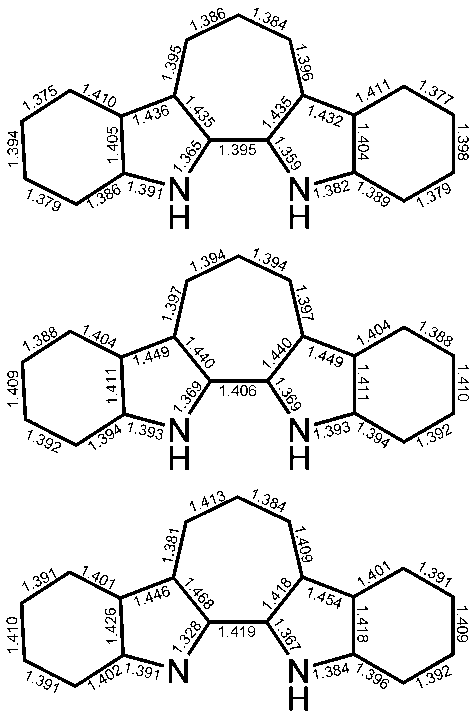 | Figure 4. Average bond lengths (in Å) of the crystal structures of 9H+TfO– (top) and bond lengths of the calculated structures of 9H+ (middle) and 9 (bottom) |
Changes of the absorption spectrum of 9 were also observed in the presence of metal ion. While any clear change was not observed in the presence of Li+, Na+, and Ag+, the spectrum varied in the presence of other metal ions. The spectra of 9 in the presence of Mg2+, Hg2+, and Pb2+ are very similar to the one observed in TFA, suggesting that these metal ions probably interact with 9 like protonation to form complex A, shown in Figure 6. On the other hand, the spectra of 9 in the presence of Zn2+ and Cd2+ are different from these spectra, showing a slightly intense shoulder band at 360 nm and two relatively intense absorption bands at a range between 450–550 nm (Figure 5). For these metal ions, 9 may form bidentate complex B. According to Shannon [16], the order of ionic radii of these divalent metal ions is Pb2+ (0.98 Å) > Cd2+(0.78 Å) > Hg2+(0.69 Å) > Zn2+(0.60 Å) > Mg2+(0.57 Å). Therefore, Mg2+ seems to be too small and Pb2+ too large for their bidentate complexation with 9. Although the ionic radius of Hg2+ is between Cd2+ and Zn2+, nature of its softness [17] may make difficult to form a stable complex with 9, which has relatively more electronegative nitrogen atoms as a ligand center. In order to clarify this hypothesis, isolation of the metal-ion complexes of 9 is now under progress.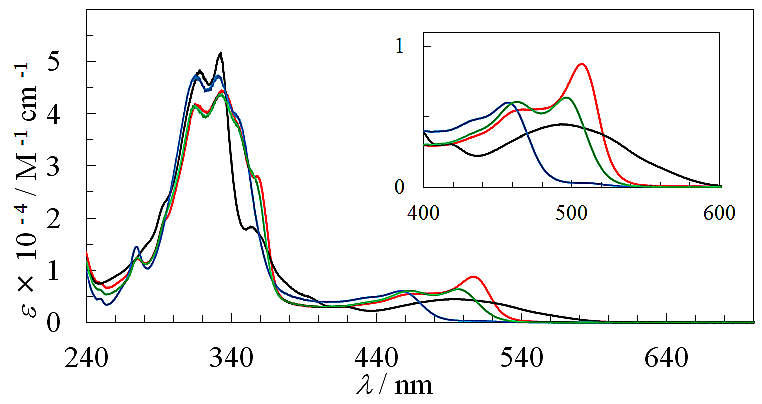 | Figure 5. Absorption spectra of 9 in the presence of a large excess (100 eq.) of Li+ (black), Mg2+ (blue), Zn2+ (green), and Cd2+ (red). Inset is expanded spectra at a range of 400–600 nm |
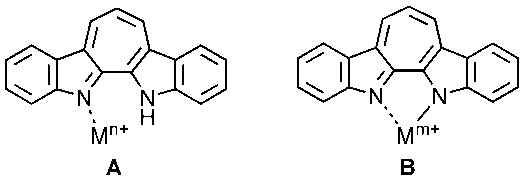 | Figure 6. Modes of complexation of 9 with metal ions |
3. Experiments
3.1. General
Melting points were measured on a Yanaco MP-3 and are uncorrected. IR spectra were recorded on a JASCO FT/IR-4100 spectrometer. UV-vis spectra were measured on a Shimadzu UV-2550 spectrometer. 1H- and 13C-NMR spectra were recorded on JEOL
400 and ECA500 spectrometers. Chemical shift value of tetramethylsilane (
0 ppm) for 1H-NMR and 13C-NMR spectra was used as internal standard. Mass spectra were measured on a JMS-700 mass spectrometer. Column chromatography was performed with Silica gel 60N from Kanto Chem. Co. 1,2-Dichloro- ethane was purchased from Kanto Chem. Co. and was distilled over CaH2. Oxalyl chloride was purchased from Wako Chem. Co. and was used without purification. TfOH and TFA were purchased from Tokyo Chem. Industry, Inc. 2,2’-Biindole was prepared in two steps from o-anisidine according to a procedure reported by Bergman et al. [9]
3.2. 1H-Cyclohepta[2,1-b;3,4-b’]diindole (9)
A solution of 119 mg (1.20 mmol) of N,N-dimethylamino-acrolein in 3 mL of 1,2-dichloroethane (DCE) was added to an ice-cooled solution of 151 mg (1.00 mmol) of oxalyl- chloride in 2 mL of DCE. To this mixture was added a suspension of 232 mg (1.00 mmol) of 2,2’-biindole (10) in 15 mL of DCE and the mixture was refluxed on an oil bath for 16 h under nitrogen atmosphere. The resulted bright yellow reaction mixture was poured into a saturated Na2CO3 aqueous solution and the water layer was extracted with chloroform (50 mL x 3). The combined organic layer was washed with a brine and was dried over Na2SO4. The solvent was removed and the residue was purified by silica gel column chromatography eluted with 5%EtOH-chloroform to give 154 mg (57% yield) of 9 as dark red plates, m.p. = 297–298℃. 1H NMR (400 MHz, CDCl3)
= 4.72 (br. 1H, N–H), 7.46 (t, J = 7.7 Hz, 2H, H-4,10), 7.56 (t, J = 7.7 Hz, 2H, H-3,11), 7.64 (d, J = 7.7 Hz, 2H, H-2,12), 7.70 (t, J = 10.0 Hz, 1H, H-7), 8.36 (d, J = 7.7 Hz, 2H, H-5,9), 8.90 (d, J = 10.0 Hz, 2H, H-6,8) ppm; 1H NMR (400 MHz, TFA-d)
=7.67 (t, J = 7.3 Hz, 2H, H-4,10), 7.87 (t, J = 7.3 Hz, 2H, H-3,11), 7.90 (d, J = 7.3 Hz, 2H, H-2,12), 8.29 (t, J = 9.9 Hz, 1H, H-7), 8.42 (d, J = 7.3 Hz, 2H, H-5,9), 9.32 (d, J = 9.9 Hz, 2H, H-6,8) ppm; 1H NMR (400MHz, 16% NaOD- D2O/DMSO-d6 = 1 : 0.6)
= 7.36 (t, J = 7.8 Hz, 2, H-4,10), 7.38 (t, J = 9.5 Hz, 1H, H-7), 7.58 (t, J = 7.8 Hz, 2H, H-3,11), 7.95 (d, J = 7.8 Hz, 2H, H-2,12), 8.33 (d, J = 7.8 Hz, 2H, H-5,9), 8.84 (d, J = 9.5 Hz, 2H, H-6,8) ppm; 13C NMR (100 MHz, CDCl3)
=115.4 (C-2,12), 120.3 (C-5,9), 122.1 (C-7), 122.4 (C-4,10), 126.3 (C-5a,8b), 129.3 (C-8,11), 131.1 (C-5b,8a), 132.4 (C-6,8), 142.0 (C-13a,13b), 144.5 (C-1a, 12a) ppm; IR (KBr)
= 1402vs, 1393vs, 1215s, 1203s, 732s cm-1; UV-vis (CH3CN)
=
332 (4.70), 353 (4.25), 394sh (3.69), 419 (3.45), 494 (3.65) nm; MS (70 eV) m/z (rel int.) = 268 (M+, 100), 267 (13), 266 (11), 240 (4), 134 (10). HRMS Calcd for C19H12N2 268.1001; Found 268.1001.
3.3. 1,13H-Cyclohepta[2,1-b;3,4-b’]diindolium triflate (9H+TfO–)
To a solution of 11.2 mg
of TfOH in 10 mL of dichloromethane was added 20.0 mg (74.6 mol) of 9. The solvent was removed under reduced pressure and the residue was recrystallized from a mixture of dichloromethane and hexane to give 27.0 mg (88% yield) of 9H+TfO– as yellow solids, m.p. > 300℃. 1H NMR (500 MHz, CDCl3)
= 1.51 (s, 2H, N–H), 7.60 (t, J = 7.9 Hz, 2H, H-4,10), 7.83 (t, J = 7.9 Hz, 2H, H-3,11), 8.05 (d, J = 7.9 Hz, 2H, H-2,12), 8.23 (t, J = 10.0 Hz, 1H, H-7), 8.38 (d, J = 7.9 Hz, 2H, H-5,9), 9.27 (d, J = 10.0 Hz, 2H, H-6,8) ppm; 13C NMR (126 MHz, CDCl3)
= 114.5, 120.7, 124.2, 124.7, 126.2, 131.7, 132.5, 135.1, 136.9, 141.2ppm [15]; IR (KBr)
= 1620s, 1397vs, 1295vs, 1254s, 1210vs, 1166vs, 1027vs, 749vs, 731s, 635s cm-1. A sample for X-Ray crystallographic analysis was obtained by further recrystallization from methanol.
3.4. X-Ray Crystallographic Analysis of 9H+TfO–
Diffraction measurements were conducted using a Rigaku R-AXIS RAPID diffractometer at –100℃. Crystal data for 9H+TfO– are as follows; triclinic, space group; P-1 (# 2), a; 7.0362(2) Å, b; 12.5278(3) Å, c; 20.7784(4) Å,
96.7827(7)°,
90.0115(7)°,
90.0034(7)°,
1818.76(6) Å3, Z; 4, R; 0.0893, wR2; 0.2353, R1; 0.0729
and S; 1.092. The relatively large R values are mainly attributed to thermal vibration of fluorine atoms of the triflate anion. Tables of fractional atomic coordinates, thermal parameters, bond lengths, and angles have been deposited at the Cambridge Crystallographic Data Centre, 12 Union Road, Cambridge CB2 1EZ, United Kingdom (CCDC 1038190) [Derect line: +44 1223 762910, Fax: +44 (0) 1233 336033, e-mail: deposit@ccdc.cam.ac.uk].
4. Conclusions
We have demonstrated that the title parent compound 9 could be synthesized from 2,2’-biindole (10) by its condensation with1-(N,N-dimethylamino)-3-chloropropenium chloride. Compound 9 shows not only basic nature but also acidic nature. Spectral changes of UV-vis and NMR spectra were discussed based on protonation and deprotonation of 9. Changes of the UV-vis spectrum in the presence of various metal ions were also described.
ACKNOWLEDGEMENTS
Financial support from the Faculty of Science in Shinshu University (for M.O.) is greatly acknowledged.
References
[1] | T. Sasaki, I. I. Ohtani, J. Tanaka, and T. Higa, 1999, Iheyamines, new cytotoxic bisindole pigments from a colonial ascidian, Polycitorella sp. Tetrahedron Lett., 40, 303–306. |
[2] | J.-Y. Su, Y. Zhu, L.-M. Zeng, and X.-H. Xu, 1997, A new bisindole from alga Caulerpa serrulata. J. Nat. Prod., 60, 1043–1044. |
[3] | P. M. Fresneda, P. Molina, and M. A. Saez, 1999, The first synthesis of the bis(indole) marine alkaloid caulersin. Synlett, 10. 1651–1653. |
[4] | N. Wahlström, B. Stensland, and J. Bergman, 2004, Synthesis of the marine alkaloid caulersin. Tetrahedron, 60, 2147–2153. |
[5] | Y. Miki, Y. Aoki, H. Miyatake, T. Minematsu, and H. Hibino, 2006, Synthesis of caulersin and its isomers by reaction of indole-2,3-dicarboxylic anhydrides with methyl indoleacetates. Tetrahedron Lett., 47, 5215–5218. |
[6] | I. L. Baraznenok, V. G. Nenajdenko, and E. S. Balenkova, 2003, Synthesis of CF3-heterocycles based 4-dimethylamino- 1,1,1-trifluoro-3-buten-2-one. Chem. Heterocyclic Comp., 39, 776–779. |
[7] | N. Wahlström, J.Slätt, B. Stensland, A. Ertan, J. Bergman, and T. Janosik, 2007, Synthetic applications of cyanoacetylated bisindoles: synthesis of novel cycloheptadiindoles, indolocarbazoles and related aza analogues. J. Org. Chem., 72, 5886–5889. |
[8] | J. B. Bremner, W. Sengpracha, and B. W. Skelton, 2008, A compact approach to an isomeric iheyamine. A system and x-ray crystal structure of 5-methyl-5H-azepino[2,3-b:4,5-b’] -diindole. Synth. Commun., 38, 1931–1939. |
[9] | J. B. Bergman, E. Koch, and B. Pelcman, 1995, 2,2’-Biindolyl revisited. Synthesis and reactions. Tetrahedron, 51, 5631–5642. |
[10] | M. Hanke and C. Jutz, 1979, Synthese des 5, 5’-Biazulenyls, Angew. Chem., 91, 227; Angew. Chem. Int. Ed. Engl., 18, 214-215. |
[11] | V. B. Mochalin and Y. N. Porshnev, 1977, Advances in the chemistry of azulene, Russ. Chem. Rev., 46, 530–547 |
[12] | K.-P. Zeller, 1985, in Houben-Weyl; Methoden der Organischen Chemie, 4th ed. Vol. 5, Part 2C, edited by H. Kropf, pp127–418, Georg Thieme, Stuttgart. |
[13] | For 1H NMR data in TFA-d1 and NaOD-DMSO-d6, see Experiments. |
[14] | DFT calculations were carried out by with the Gaussian 03 program, Revision D.01 program, Gaussian, Inc.: Pittsburgh, PA, 2003. |
[15] | Signals for the trifluoromethyl group of triflate anion were not observed because of their low intensity. |
[16] | R. D. Shannon, 1976, Revised effective ionic radii and systematic studies of interatomic distances in halides and chalcogenides, Acta. Cryst., A32, 751-767. |
[17] | The order of hardness parameters (ηA) of these metal ions, suggested by Parr and Pearson, is as follows; Mg2+(32.5 eV) > Zn2+(10.8 eV) > Cd2+(10.3 eV) > Pb2+(8.5 eV) > Hg2+(7.7 eV): R. G. Parr and R. G. Pearson, 1983, Absolute hardness: Companion parameter to absolute electronegativity. J. Am. Chem. Soc., 105, 7512-7516. |