Priyadarsan K. P.1, S. Panda1, A. Nayak2, M. R. Acharya3
1Department of Mathematics, NIT Calicut, NIT Campus (P.O), Kozhikode, Kerala, India
2Department of Mathematics, Silicon Institute of Technology, Bhubaneswar, Bhubaneswar, India
3Department of Physics, College of Basic Science, and Humanities, OUAT, Bhubaneswar, India
Correspondence to: Priyadarsan K. P., Department of Mathematics, NIT Calicut, NIT Campus (P.O), Kozhikode, Kerala, India.
Email: |  |
Copyright © 2015 Scientific & Academic Publishing. All Rights Reserved.
This work is licensed under the Creative Commons Attribution International License (CC BY).
http://creativecommons.org/licenses/by/4.0/

Abstract
Our endeavour is to present a numerical analysis of momentum and mass transfer characteristics with chemical reaction in a transient viscoelastic fluid flow influenced by a vertical stretching sheet in a porous medium. Moreover, the flow is mixed convection type and controlled by magnetic field. Soret (thermal diffusion) and Dufour (diffusion thermo) effects due to coupled heat and mass transfer also augment the scope of the analysis together with transient case. Solutions are obtained for unsteady state using Keller box method. A parametric study illustrating the influence of viscoelastic parameter, magnetic parameter, permeability parameter, radiation parameter, Soret number, Dufour number and chemical reaction parameter have been studied and illustrated graphically.
Keywords:
Porous media, Magnetic field, Soret and Dufour number, Keller-Box method
Cite this paper: Priyadarsan K. P., S. Panda, A. Nayak, M. R. Acharya, Transient Mixed Convection Flow of a Viscoelastic Fluid over a Vertical Stretching Sheet Coupled with Heat-Mass Transfer and Chemical Reaction, American Journal of Fluid Dynamics, Vol. 5 No. 3, 2015, pp. 76-86. doi: 10.5923/j.ajfd.20150503.02.
1. Introduction
Viscoelastic fluid flow finds lot of application in engineering processes. Such process include glass fiber, fiber and paper production, crystal growing, drawing of plastic sheets etc. Other applications include drilling of petroleum, manufacturing of foods and slurry transportation. Mass transfer effect have also been important in chemical processing equipment. In some polymer processing unit the flow is also driven over porous or impermeable stretching surface. According to Eckert and Drake [1] for isotropic separation the thermal diffusion (Soret) effect and diffusion thermo (Dufour) effect cannot be neglected. In many chemical engineering processes chemical reaction takes place between a foreign mass and the working fluid which moves due to stretching of surfaces. The simplest chemical reaction is the first order reaction in which rate of reaction is directly proportional to the species concentration. A comprehensive paper on mass transfer with chemical reacting species for viscoelastic fluid over a porous stretching sheet have been studied by Cortell [2]. Hayat et al.[3] considered heat and mass transfer for Soret and Dufour effect on mixed convection boundary layer flow over a stretching vertical surface in a porous medium filled with viscoelastic fluid. Shateyi et al. [4] have considered the influence of magnetic field on heat and mass transfer of a mixed convection over a vertical surfaces in presence of Soret and Dufour effect as well as Hall effect in a porous medium. Makinde [5] studied the mixed convection of a Boussinesq fluid with Soret and Dufour effect over a vertical plate embedded in a porous medium. The flow is also controlled by magnetic field. Recently a mathematical model describing mass transfer with Soret and Dufour effect on mixed convection boundary layer flow over a stretching sheet filled with viscoelastic fluid in presence of magnetic field has been reported by Gbadeyan et al. [6]. As already stated Soret effect referred to species differentiation developing in an initial homogeneous mixture submitted to a thermal gradient and Dufour effect referred to heat flux produced by a concentration gradient. Cheng [7] considered these effects with mixed convection over a vertical wedge in a porous medium. He found that wedge angle parameter is insignificant for natural and mixed convection flow.Above mentioned work refers to steady state phenomena. However unsteady conduction both transient and periodic is very important in many applications of heat transfer. Designers in technological areas are often faced with start up, operating or instability transients. These must be understood sufficiently well to guide material selection. This relate to the temperature limitation of the materials. Unsteady conduction mechanisms are also very important in many earth science due to ever changing effects of solar radiation and atmospheric condition. For example, both daily and seasonal temperature changes cause complicated time dependent temperature variations in the soil. There are basically two kinds of unsteady processes. One is transient, where temperature field changes with time from an initial condition towards an eventually steady state. An example arises when an object at an initial temperature is immersed in surrounding at some different temperature. The temperature difference decays with time. The second process is periodic in which the temperature at each location in the region vary periodically with time. Appropriately the temperature of the soil surface into downward vary periodically due to annual or delay variation of atmospheric conditions.In this connection recent unsteady works on mixed convection viscoelastic flow are cited below. This include the study of unsteady MHD mixed convection stagnation point flow of a viscoelastic fluid on a vertical surface by Ahmad and Nazar [8]. Bhargava and Singh [9] employed element-free Galerkin method (EFMG) to solve the coupled non-linear differential equations evolved in unsteady MHD flow and heat transfer of a second grade fluid with viscous dissipation and Joule heating. Literature survey also witnessed unsteady flow of third grade fluid with Soret and Dufour effects [3]. Most recently Mohamed et al. [10] have reported unsteady MHD double diffusive convection boundary layer flow past a radiative hot vertical surface with chemical reactions.The growing need of chemical reactions in chemical and hydro metallurgical industries requires the study of chemical reaction with heat and mass transfer. Elaborate study of chemical reaction by Mohapatra et al. [11] exercises its need. Recently Nayak et al. [12] extensively analyzed the Soret and Dufour effects on mixed convection unsteady MHD boundary layer flow over a stretching sheet. In view of above, the aim of present study is to examine transient phenomena of Soret and Dufour effect in mixed convection viscoelastic fluid flow over a vertical stretching sheet controlled by magnetic field. Porous media are generally used to insulate a heated body to maintain its temperature, which is generated due to chemical reaction. Porous media are also used in diminishing fluid temperature. Therefore it is essential to estimate chemical reaction on heat and mass transfer.
2. Problem Formulation
Let us consider an unsteady laminar incompressible viscoelastic two-dimensional mixed convection flow of an electrically conducting fluid due to stretching of a heated or cooled vertical sheet in a porous medium in presence of chemical reaction. The x-axis is taken along the stretching direction of the sheet and y-axis is normal to it (Fig. 1). The flow field is subjected to a transverse uniform magnetic field of strength
. Prior to time (
), the sheet has uniform temperature
and it is at rest in an unbounded quiescent fluid at constant temperature
. Then at time
, the sheet is suddenly stretched with a velocity
which varies linearly with the distance
from the origin along the surface. At the same time the surface temperature is suddenly raised from
to
and thereafter maintained at constant temperature
. This sudden change in surface velocity and surface temperature gives rise to unsteadiness in the velocity and thermal fields. Here the stretching surface is assumed to be electrically non-conducting. The magnetic Reynolds number is considered to be small such that induced magnetic field is negligible in comparison to applied magnetic field. The electric field due to polarization of changes is negligible due to absence of external applied electric field. Similarly, the electric field
is zero for small and moderate values of magnetic field. Consequent upon this Hall term in Ohm's law is absent as it has less effect on the flow field. Therefore, only the magnetic field contributes to Lorentz force. In most of stretching problem the velocity of stretching surface is small, therefore the effect of viscous dissipation term in the energy equation is neglected. Small values of magnetic field implies the absence of Ohmic heating and Hall term in the energy equation. The flow is generated due to two equal and opposite forces (buoyancy force and force corresponding to stretching along the x-axis.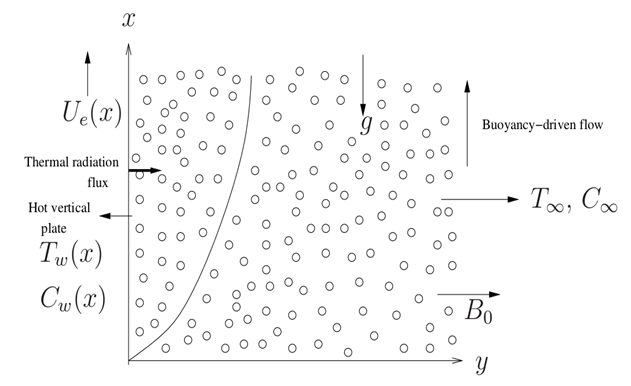 | Figure 1. Sketch of flow geometry |
The variable temperature
and concentration
of the surface are assumed to be suddenly increased or decreased to the uniform ambient temperature
and the far away concentration
. For the assisting flow the x-axis directs upward in the direction of the stretching hot surface such that the stretching induced flow and the thermal buoyant flow assist each other. For the opposing flow, the x-axis directs vertically downward in the direction of the stretching hot surface but in this case the stretching induced flow and the thermal buoyant flow oppose each other. The density variation is taken into consideration, so that the Boussinesq approximation for both the temperature and concentration gradient can be adopted. Since heat and mass transfer occur simultaneously the driving potentials are more indicate in nature. For isotope separation and in mixture between gases of light molecular weight and medium molecular weight the diffusion thermo and thermal diffusion effects can not be neglected. We also consider a chemical reaction taking place between a foreign species and working fluid which moves due to stretching of surfaces. Under these assumptions the transient boundary layer equations under Boussinesq approximations are given below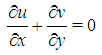 | (1) |
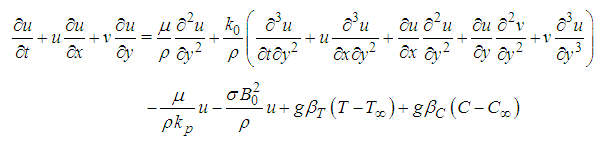 | (2) |
 | (3) |
 | (4) |
The symbol
and
denote the fluid velocity in the x- and y- direction. Here
and
represent the temperature and concentration fields respectively,
is the density,
is the coefficient of viscosity,
is the viscoelastic parameter,
is the permeability of the porous medium,
is the electrical conductivity,
is the acceleration due to gravity,
is thermal expansion coefficient,
is the volumetric coefficient of expansion with concentration,
, k is the thermal conductivity of the porous medium) is the thermal diffusivity,
is the specific heat at constant pressure,
is the radiative heat flux,
is the volumetric heat generation/absorption rate,
is the mass diffusivity,
is the thermal diffusion ratio,
is the concentration susceptibility,
is the chemical reaction rate on the species concentration and
is the mean fluid temperature. Using the Rosseland approximation (see e.g. [13], [14]) the radiative heat flux
could be expressed by 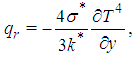 | (5) |
where
represents Stefan-Boltzmann constant and
is the Rosseland mean absorption coefficient. Assuming that the temperature difference within the flow is small,
can be written as 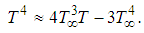 | (6) |
The initial and boundary conditions of Eqs. (1)-(4) are 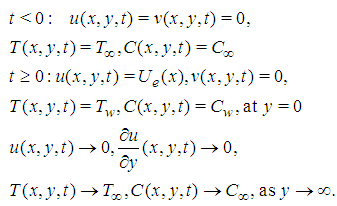 | (7) |
The subscripts
and
refer to the wall and free stream conditions. Both the wall temperature and concentration are assumed to have linear form i.e. 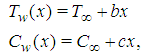 | (8) |
where
and
are the constants with
for heated plate
and
for cooled surface
, respectively.For an impulse motion the change of variables are given in Rashad et al. [15], Ishak et al. [16] and Williams et al. [17] 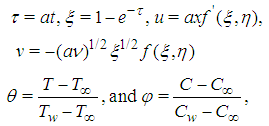 | (9) |
where
,
is the dynamic viscosity and the prime denotes differentiation with respect to
. It can be noted that Eq. (9) is compatible with Eq. (1) and the definition of
indicates that the region of time integration
may become finite, i.e.
.Using Eqs. (5), (6) and (9) the model Eqs. (2)-(4) reduces to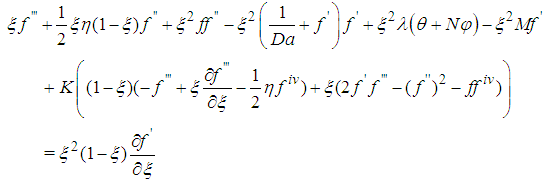 | (10) |
 | (11) |
 | (12) |
The dimensionless numbers are • The Prandtl number
that represents the ratio of momentum to thermal diffusivity, • The Schmidt number
that represents the ratio of momentum to mass diffusivity, • The dimensionless number
is the Dufour number,
is the Soret number,
is the Darcy number,
is the local magnetic field parameter,
is the ratio of buoyancy force due to thermal diffusion,
is the chemical reaction parameter 
is the mixed convection parameter,
is the visco-elastic parameter,
is the heat source parameter and
is the radiation parameter, where
is the local Grashof number and
is the local Reynolds number. It may be noted that for
corresponds to assisting flow (heated plate),
corresponds to opposing flow (cooled plate) and
corresponds to forced convection flow, respectively.In view of the scaling relations Eq. (9), the corresponding boundary conditions Eq. (7) are: 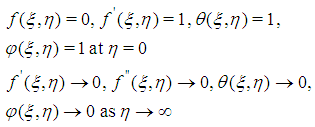 | (13) |
3. Numerical Procedure
The model Eqs. (10)-(12) together with boundary conditions Eq. (13) are solved using an implicit finite-difference scheme known as the Keller-box method (Cebeci and Bradshaw [18]). We first introduce new dependent variables in order to reduce the equations to a system of first order equations. Therefore, the new dependent variables are
,
and
, such | (14) |
So that Eqs. (10)-(12) can be written as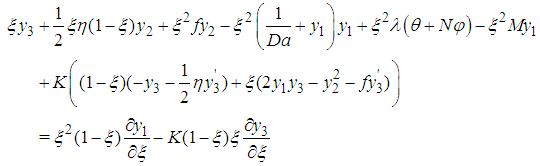 | (15) |
 | (16) |
 | (17) |
We now consider the net rectangle in the
plane and the net points defined as follows:  | (18) |
 | (19) |
where
and
are the uniform time and grid spacing.Next, the derivatives in the
-direction are replaced by finite difference, for example the finite difference form for any points are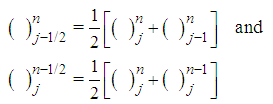 | (20) |
and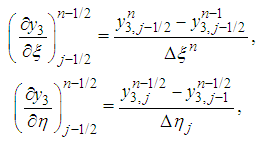 | (21) |
Now centering about
, the finite difference form of Eqs. (14) can be written as: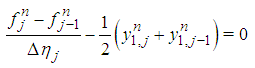 | (22) |
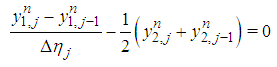 | (23) |
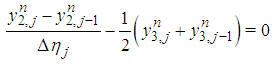 | (24) |
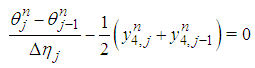 | (25) |
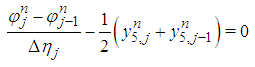 | (26) |
Now centering about
, Eqs. (15)-(17) can be written as | (27) |
 | (28) |
 | (29) |
Where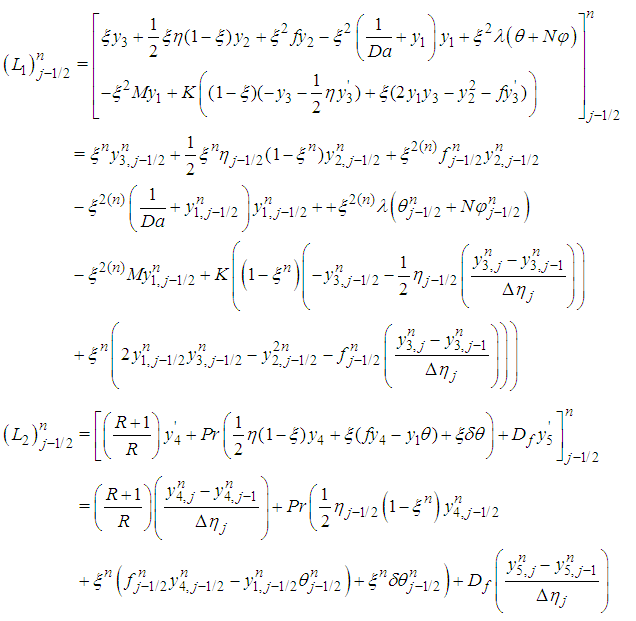
Eqs. (22)-(29) describes an implicit time discretization scheme. Since the governing equation is non-linear, a system of non-linear algebraic equations needs to be solved at each time step. We use fsolve in MATLAB for this purpose. A good initial starting guess is required to solve the non-linear equations. A reasonable initial guess for the velocity, temperature and concentration is chosen to be zero throughout the discrete domain at the first time step. The solution from the previous time step can be used otherwise. Convergence is achieved in less than 100 iterations and the convergence criterion is that the norm of the residuals should be less than
.
4. Results and Discussion
Figs. 2, 3 and 4 depict the velocity, temperature and concentration profiles at different times
(non-dimensional). For a particular value of
, velocity, temperature and concentration field decreases with increase in
and becomes zero at the outside of the boundary layer, which satisfies boundary condition
,
, and
. The velocity, temperature and concentration distribution falls very rapidly near the hot stretching sheet and then steadily decreases to zero away from the surface. 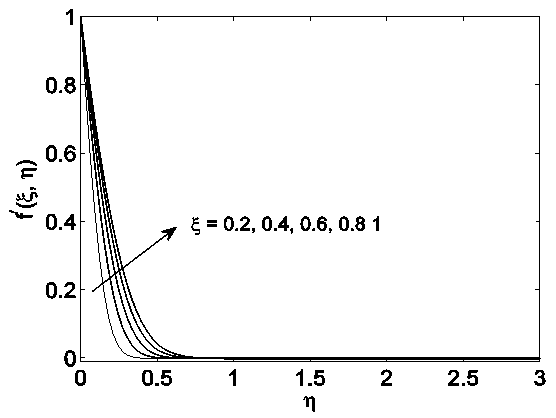 | Figure 2. Velocity profile demonstrating the progression of the transient solution towards the final steady-state solution. The parameters are    |
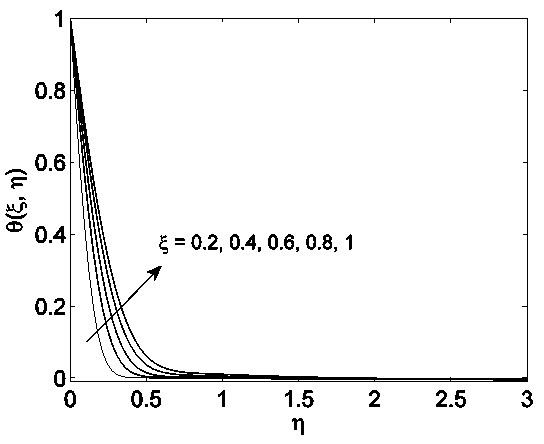 | Figure 3. Temperature profile demonstrating the progression of the transient solution towards the final steady-state solution. The parameters are     |
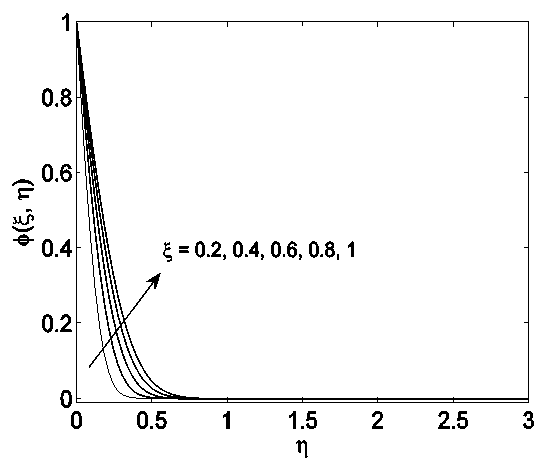 | Figure 4. Concentration profile demonstrating the progression of the transient solution towards the final steady-state solution. The parameters are    |
Fig. 5 show velocity profiles for different values of magnetic parameter
. It is observed that magnetic field decelerates fluid velocity and there is a decrease in boundary layer thickness. Physically this implies that increase in magnetic field increases induction drag, which tends to destroy motion across field lines. The effects of viscoelastic parameter have been depicted in Fig. 6. The velocity boundary layer decreases for large value of viscoelastic parameter. Fig. 7 depicts the effect of mixed convection parameter both for assisting and opposing flow: for
corresponds to
, i.e heat transfer takes place from wall to boundary. Consequently boundary layer thickness is more. For
corresponds to
i.e. the plate is cooler than the ambient and heat transfer occurs from outside to plate. So the velocity field decreases.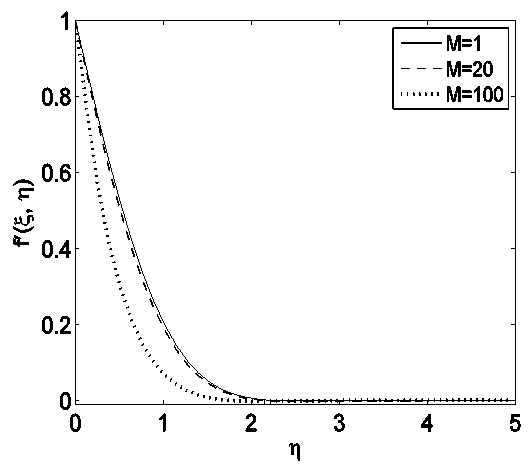 | Figure 5. Influence of M with   |
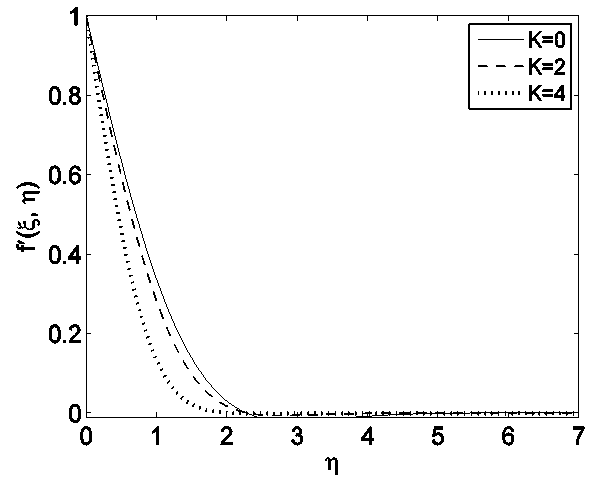 | Figure 6. Influence of K with  |
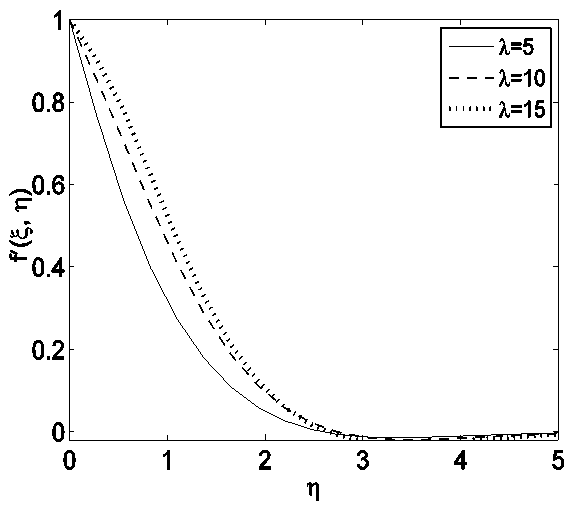 | Figure 7. Influence of with   |
In fact Dufour parameter
tends to thicken thermal boundary layer and thus decreasing heat transfer rate at the wall (Fig. 8). Similarly, Soret parameter
tends to thicken concentration boundary layer, thus decreasing the mass transfer rate in the wall (Fig. 9).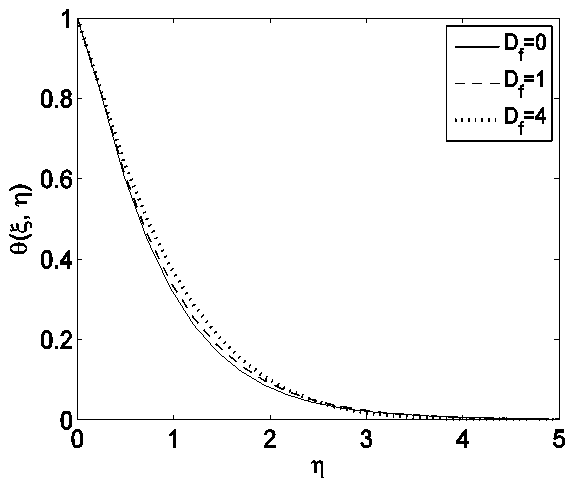 | Figure 8. Influence of with   |
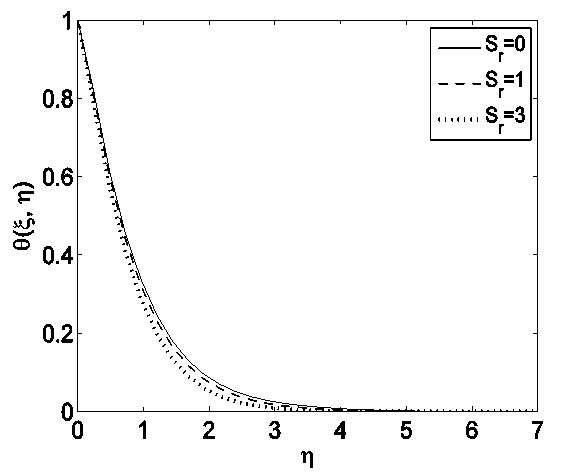 | Figure 9. Influence of with  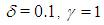 |
Fig. 10 illustrates decrease in temperature field with increase in radiation parameter. Again the effect of viscoelastic parameter on temperature profiles is illustrated in Fig. 11. Increasing viscoelastic parameter reduces temperature field. Temperature near the wall decreases rapidly and then decreases slowly away from the sheet. In case of assisting flow with increase in mixed convection parameter, temperature distribution field decreases (Fig. 12).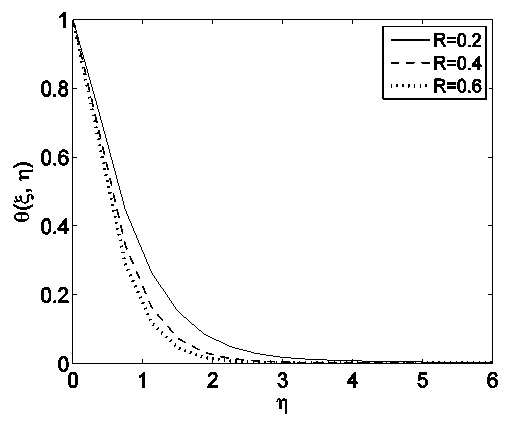 | Figure 10. Influence of R with   |
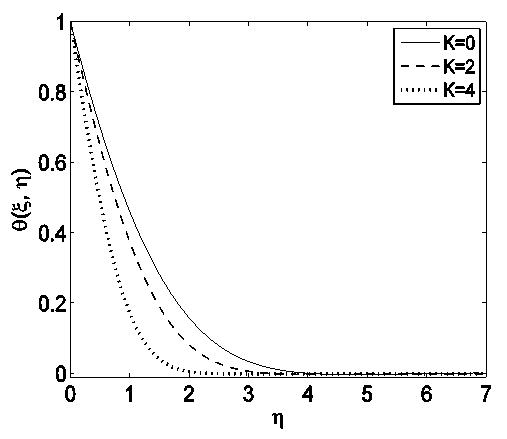 | Figure 11. Influence of K with   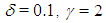 |
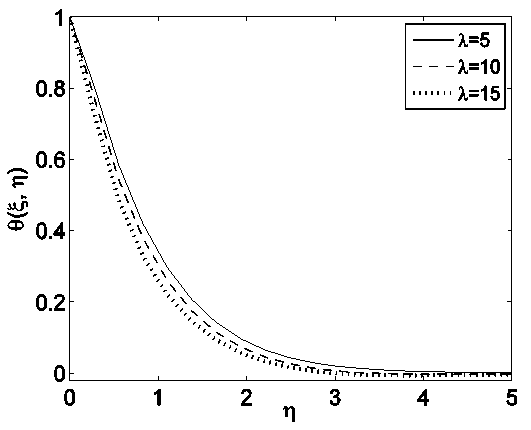 | Figure 12. Influence of with 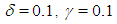 |
Fig. 13 and 14 illustrate the effect of Dufour number and Soret number in concentration profiles. It is observed that increasing Dufour effect, concentration profile decreases whereas increasing Soret number temperature field increases. Fig. 15 depicts the effect of ratio of momentum diffusivity to thermal diffusivity. When thermal diffusivity decreases concentration profiles are reduced. Effect of radiation parameter (Fig. 16) is opposite to that of effect of mixed convection parameter (Fig: 18) and effect of Schimdt number (Fig. 19) on concentration profiles. Viscoelastic and chemical reaction parameters reduce concentration profiles (Fig. 17). The effect of chemical reaction is shown in Fig. 20. For
(destructive reaction) the second term in the right hand side of Eq. (4) becomes positive and it contributes to the concentration reduction. Increasing
value decreases the concentration.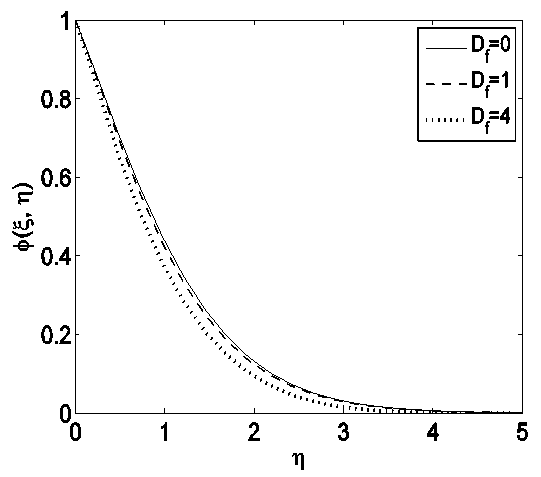 | Figure 13. Influence of with  |
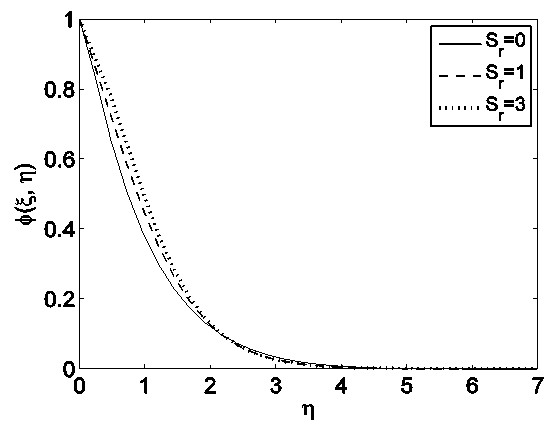 | Figure 14. Influence of with   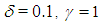 |
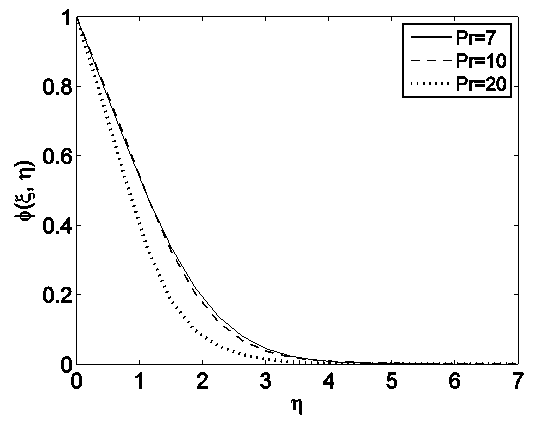 | Figure 15. Influence of with    |
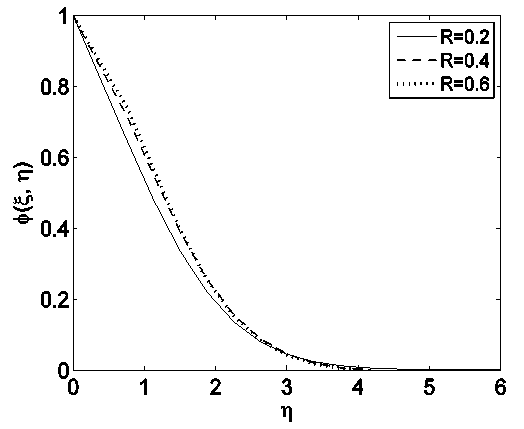 | Figure 16. Influence of R with    |
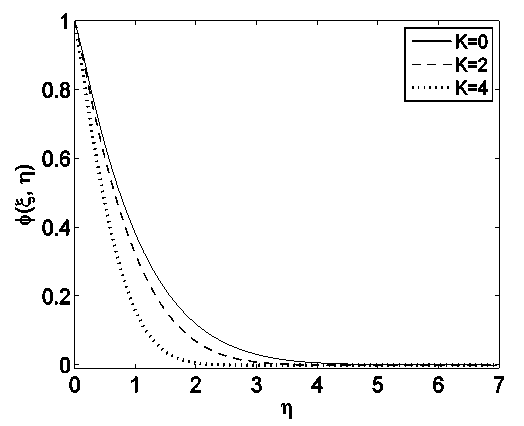 | Figure 17. Influence of K   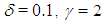 |
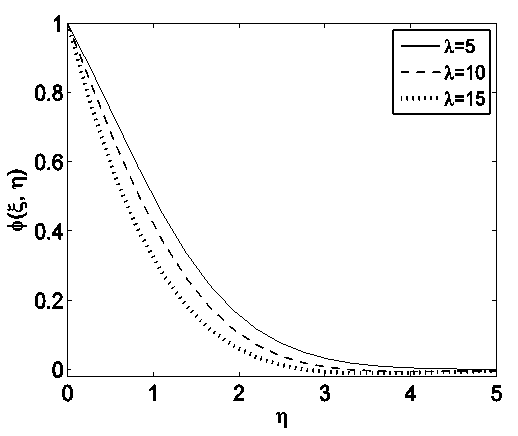 | Figure 18. Influence of with    |
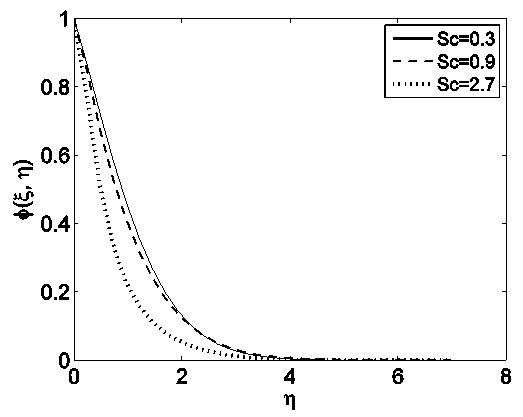 | Figure 19. Influence of Sc with  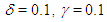 |
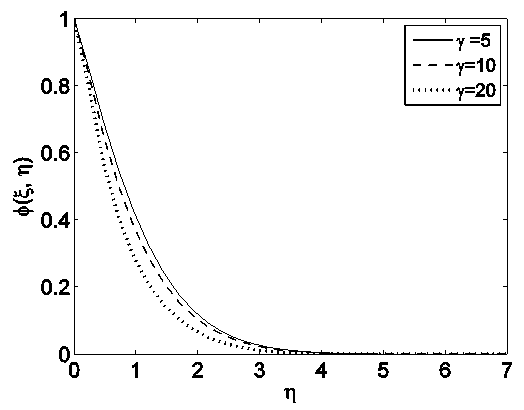 | Figure 20. Influence of with   |
5. Conclusions
This work studies the Soret and Dufour effects on mixed convection heat and mass transfer over a vertical stretching sheet in a porous medium with chemical reaction. The final outcomes of the discussion are mentioned below: • Time integration parameter enhances the flow • Viscoelastic parameter and magnetic parameter reduces the thickness of flow field • Mixed convection parameter
influences the flow depending on whether
or not. The positive value of
implies heat flow occurs from wall to surrounding and the negative value of
indicated heat flow occurs from ambient to wall • Dufour number increases the temperature field where as Soret number reduces the temperature field • Soret and Dufour numbers have significant effects on distribution of velocity, temperature and concentration • Increase in chemical reaction parameter produces a decrease in concentration
References
[1] | E R G Eckert and Robert M Drake, 1972, Analysis of Heat Transfer and Mass Transfer, McGraw-Hill. |
[2] | R Cortell, 2007, Toward an understanding of the motion and mass transfer with chemically reactive species for two classes of viscoelastic fluid over a porous stretching sheet, Chemical Engineering and Processing: Process Intensification, 46, 10, 982--989. |
[3] | T Hayat, M Awais, S Asghar, and S Obaidat, 2012, Unsteady flow of third grade fluid with soret and dufour effects, Journal of Heat Transfer, 134, 6, 062001-1 -- 062001-7. |
[4] | S Shateyi, S S Motsa, and P Sibanda, 2010, The effects of thermal radiation, hall currents, soret, and dufour on mhd flow by mixed convection over a vertical surface in porous media, Mathematical problems in Engineering, 2010, 1--20. |
[5] | O D Makinde, 2011, On mhd mixed convection with soret and dufour effects past a vertical plate embedded in a porous medium, Latin American applied research, 41, 1, 63--68. |
[6] | J A Gbadeyan, A S Idowu, A W Ogunsola, O O Agboola, P O Olanrewaju, et al, 2011, Heat and mass transfer for soret and dufour’s effect on mixed convection boundary layer flow over a stretching vertical surface in a porous medium filled with a viscoelastic fluid in the presence of of magnetic field, Global Journal of Science Frontier Research, 11, 8, 97--114. |
[7] | C Y Cheng, 2012, Soret and dufour effects on mixed convection heat and mass transfer from a vertical wedge in a porous medium with constant wall temperature and concentration, Transport in porous media, 94, 1, 123--132. |
[8] | K Ahmad and R Nazar, 2010, Unsteady magnetohydrodynamic mixed convection stagnation point flow of a viscoelastic fluid on a vertical surface, JQMA, 6, 2, 105—117. |
[9] | R Bhargava and S Singh, 2012, Numerical simulation of unsteady mhd flow and heat transfer of a second grade fluid with viscous dissipation and joule heating using meshfree approach, World Academy of Science, Eng. and Tech, 6, 6, 1098--1104. |
[10] | R A Mohamed, A A Osman, and S M Abo-Dahab, 2013, Unsteady mhd double-diffusive convection boundary-layer flow past a radiate hot vertical surface in porous media in the presence of chemical reaction and heat sink, Meccanica, 48, 4, 931--942. |
[11] | N Mahapatra, G C Dash, S Panda, and M Acharya, 2010, Effects of chemical reaction on free convection flow through a porous medium bounded by a vertical surface, Journal of Engineering Physics and Thermophysics, 83, 1, 130--140. |
[12] | A Nayak, S Panda, and D K Phukan, 2014, Soret and dufour effects on mixed convection unsteady mhd boundary layer flow over stretching sheet in porous medium with chemically reactive species, Applied Mathematics and Mechanics, 35, 7, 849--862. |
[13] | E M Sparrow and R D Cess, 1970, Radiation Heat Transfer, Brooks Publishing Company |
[14] | H A M El-Arabawy, 2003, Effect of suction/injection on the flow of a micropolar fluid past a continuously moving plate in the presence of radiation, International Journal of heat and mass transfer, 46, 8, 1471--1477. |
[15] | A M Rashad and S M M El-Kabeir, 2010, Heat and mass transfer in transient flow by mixed convection boundary layer over a stretching sheet embedded in a porous medium with chemically reactive species, Journal of porous media, 13, 1, 75--85. |
[16] | A Ishak, R Nazar, and I Pop, 2006, Unsteady mixed convection boundary layer flow due to a stretching vertical surface, Arabian Journal for Science and Engineering, 31, 2B, 165--182. |
[17] | J C Williams, III and T B Rhyne, 1980, Boundary layer development on a wedge impulsively set into motion, SIAM Journal on Applied Mathematics, 38, 2, 215--224. |
[18] | T Cebeci and P Bradshaw, 1984, Physical and computational aspects of convective heat transfer, Springer-Verlag, New York, NY. |