Simon Mburu Njoroge1, Thomas Mutuku Munyao2, Odipo Osano3
1Department of Environmental Health, Moi University, Eldoret, Kenya
2Department of Environmental Biology and Health, University of Eldoret, Eldoret, Kenya
3Department of Environmental Earth Science, University of Eldoret, Eldoret, Kenya
Correspondence to: Simon Mburu Njoroge, Department of Environmental Health, Moi University, Eldoret, Kenya.
Email: |  |
Copyright © 2016 Scientific & Academic Publishing. All Rights Reserved.
This work is licensed under the Creative Commons Attribution International License (CC BY).
http://creativecommons.org/licenses/by/4.0/

Abstract
Pesticides have many different properties that affect their behaviour in the environment. Pesticide’s solubility in water has a great impact on leaching potential and environmental fate. The objective of this study was to determine the relationship between organic carbon based partition coefficient (koc) and pesticides solubility (S) of pesticides used along the shore of Lake Naivasha using regression analysis. The properties (S, and soil/water equilibrium partition coefficient (kd)) of pesticides selected from an inventory of pesticides used in farms around Lake Naivasha, were determined from the manufacturers’ materials safety data sheets. The organic carbon (foc) of the soil from the study area was then determined using the loss-on-ignition (LOI) method and used to calculate koc. The results showed that the soils around Lake Naivasha had a mean organic carbon (foc) content of 1.770% and a regression equation for koc and S for the area to be log koc = -0.368logS + 3.256. It was concluded that this relationship can be used to estimate the organic carbon based partition coefficient (koc) of a pesticide where S is available, and the results compared with values determined experimentally and from other models.
Keywords:
Pesticides, Groundwater contamination, Partition coefficient, Solubility, Organic carbon, Lake Naivasha
Cite this paper: Simon Mburu Njoroge, Thomas Mutuku Munyao, Odipo Osano, Modeling Relationship between Organic Carbon Partition Coefficient and Pesticides Solubility of Pesticides used along the Shore of Lake Naivasha, Kenya, American Journal of Environmental Engineering, Vol. 6 No. 2, 2016, pp. 33-37. doi: 10.5923/j.ajee.20160602.01.
1. Introduction
Pesticides have many different properties that affect their behaviour in the environment. Pesticide properties include physical and chemical characteristics such as solubility, adsorption, volatility, and the potential for degradation. Some of these properties play a significant role in the contamination processes of groundwater. For a water contaminant to be available for the downward movement of the water flow, it should be water soluble, not strongly adsorbed to soil particles, resistant to biotic and abiotic degradation reactions, and not very volatile [1-3]. Other factors influencing the processes of pesticide transport are climate, soil pedology, physical and chemical conditions and soil biota and management ([4] and [5]).Pesticide’s solubility in water has a great impact on leaching potential and environmental fate. In the study of the environmental fate of organic chemicals, the octanol/ water partition coefficient (kow) has become a key parameter. It has been shown to be correlated to water solubility, soil/sediment sorption coefficient (kd), and bioconcentration [6, 7]. Of the three properties that can be estimated from kow, water solubility is the most important because it affects both the fate and transport of chemicals [8, 9]. For example, highly soluble chemicals become quickly distributed by the hydrologic cycle, have low sorption coefficients for soils and sediments, and tend to be more easily degraded by microorganisms [10, 11].Pesticide chemicals that dissolve readily in water are highly soluble and, thus, are generally carried with the water flow. Such pesticides have a tendency to leach from the soil to groundwater. As the water solubility of a pesticide increases, there is a greater likelihood it will be leached through the soil to groundwater or runoff into surface water. In general, pesticides with solubility of greater than 30 part per million have an increased tendency to leach [12]. Once a pesticide enters a soil, a portion adheres to soil particles and some remains in solution in the soil water [13]. The soil/water equilibrium partition coefficient, also known as the adsorption coefficient (kd) is defined as the ratio between the concentration in soil and the concentration in water. It can therefore be used to estimate the dissolved or the adsorbed fraction in a soil-water system for any chemical. In a study of six pesticides (bentazone, dimethoate, MCPA, pirimicarb, propiconazole and fenpropimorph), [14] it was found that the ratio of lost to applied pesticide decreased with increasing adsorption coefficient.Each pesticide behaves differently in different soil. In order to evaluate the behaviour of a pesticide at a specific site, the organic carbon normalized soil/water equilibrium partition coefficient (koc) is used. Koc provides a measure of mobility in which the effects of soil type and management history are specifically accounted for. Organic carbon content (foc) of a site (estimated to be 58 percent of the organic matter content, is used in the calculation [15, 16]. The US EPA kd/koc conversion is based on an organic matter content of 2 percent and an organic carbon content of 1.16 percent [15]. | (1) |
Where kd = soil/water equilibrium partition coefficient, Cs = Pesticide concentration in soil Cw = pesticide concentration in water  | (2) |
Where foc = fraction of organic carbon in the soil. The larger the koc, the more strongly the pesticide attaches to soil particles and organic matter. The smaller the koc value the greater the pesticide concentration in water. Therefore, pesticides with smaller koc values are more likely to leach. The objective of this study was to determine the relationship between organic carbon based partition coefficient (koc) and pesticides solubility (S) of pesticides used along the shore of Lake Naivasha. Lake Naivasha is located in Nakuru County in the Eastern Rift Valley, about 100km Northwest of Nairobi, Kenya’s capital. Bordering the lake are some of the biggest flower farms in the world, where 80% of the cut flowers production is found around the Lake basin, making it the most important area for cut flowers in Kenya [26].
2. Material and Methods
2.1. Pesticide Properties
An inventory of pesticides used in farms around Lake Naivasha was first prepared from which 39 pesticides were selected based on their WHO toxicity classification and annual rates of application (Table 1). The properties of selected pesticides (solubility (S), and soil/water equilibrium partition coefficient (kd)) were determined from the manufacturers’ materials safety data sheets and other literature. The organic carbon (foc) of the soil from the study area was then determined and used to calculate the organic carbon partition coefficient (koc).
2.2. Soil Organic Carbon Determination
Nineteen (19) soil samples for soil organic carbon analysis were conveniently sampled from farms along the shore of Lake Naivasha (Fig. 1). The soils were sampled at a depth of 15-30 cm to eliminate plant roots which could have given higher than correct values of organic matter and organic carbon. The samples were collected on different days of the week and stored at 4oC to minimize organic compounds loss. The organic matter in the samples was determined at Moi University School of Environmental Studies Analytical Laboratory using the loss-on-ignition (LOI) method [27, 28].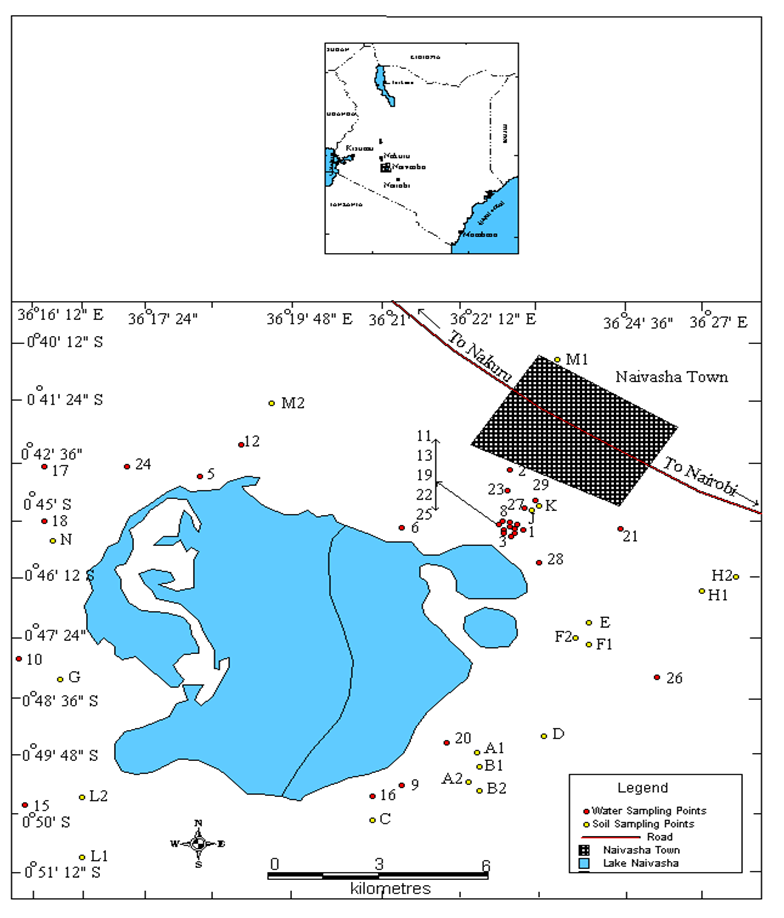 | Figure 1. Soil sampling sites |
The loss-on-ignition method was used to destroy the organic matter in the sample by heat destruction. The samples were first oven dried at 105oC to remove moisture (water content) and then weighed to determine their initial weights (wi). A known weight (wi) of sample was placed in a ceramic crucible which was then heated to 400 ±5oC overnight [27-30]. The sample was then cooled in a desiccator and weighed (wf).The organic matter (OM) was determined gravimetrically and calculated as the difference between the initial (wi) and final (wf) sample weights divided by the initial sample weight (wi) times 100% [28] : | (3) |
A conversion factor of 1.724 was used to convert organic matter (OM) to organic carbon (foc) (based on the assumption that organic matter contains 58% organic carbon, i.e., foc = OM/1.724 [27].The organic carbon partition coefficient (koc) was calculated using pesticide partition coefficient (kd) and the mean organic carbon (foc) according to equation 2.The logarithm of the organic carbon partition coefficient (logkoc) was plotted against that of solubility (logS) to determine the relationship between the two properties influencing the mobility of a pesticide into groundwater (Fig. 2).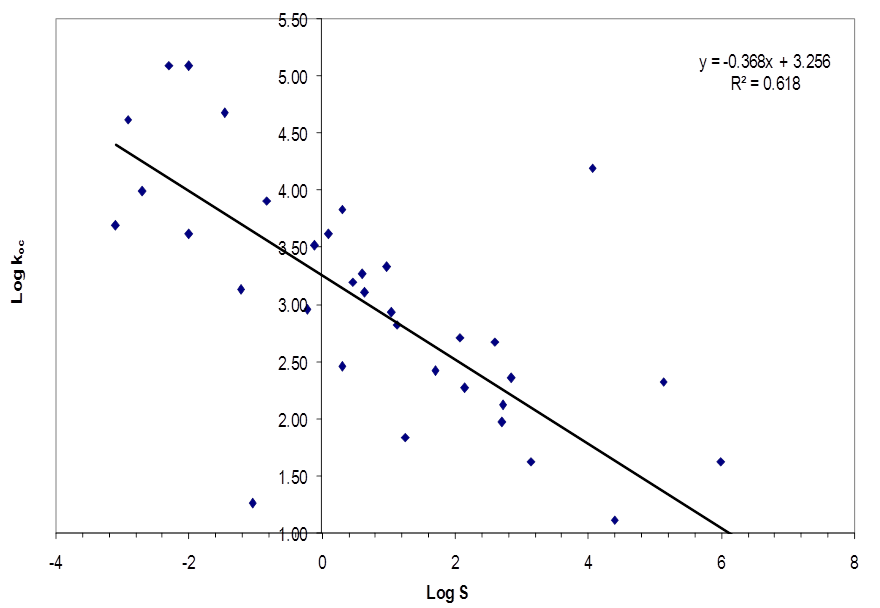 | Figure 2. Plot of Log Koc versus Log S of pesticides used on the shore of Lake Naivasha |
3. Results and Discussion
Table 1 shows the solubility and soil/water equilibrium partition coefficient (kd) of selected pesticides. Table 2 shows the values of organic matter and organic carbon of soils around Lake Naivasha. The mean organic carbon (foc) of 1.770% (table 2 was used to calculate the organic carbon based partition coefficient (koc) of 39 pesticides shown in Table 1. Figure 2 shows a plot of the logarithm of organic carbon based partition coefficient (log koc) versus the logarithm of solubility (log S).Table 1. Selected properties of pesticides used along the shore of Lake Naivasha 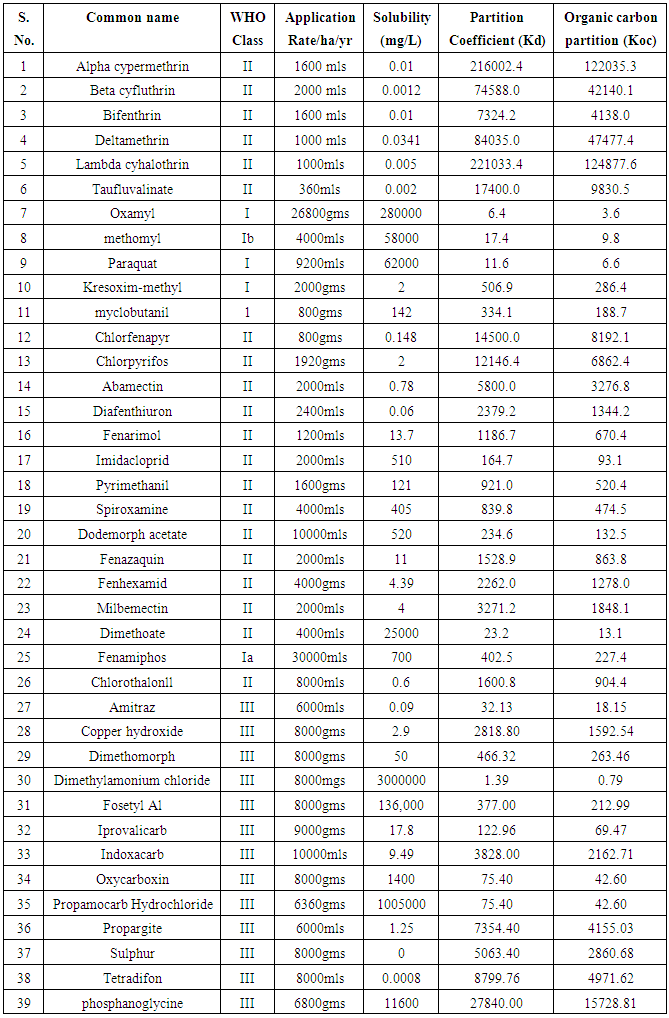 |
| |
|
Table 2. Soils organic matter and organic carbon |
| |
|
Figure 2 provides a relationship between pesticide solubility (S) and the organic carbon based partition coefficient (Koc) of the pesticides. Both properties describe the mobility of the pesticide in the soil. A linear best fit was found to describe the relationship with an equation: | (4) |
From equation 4 and Figure 2 the relationship between Koc and S is: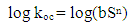 | (5) |
Where b is a constantEqn. 5 may be written as: | (6) |
Eqn. 6 gives us the relationship between Koc and S for the study area as: | (7) |
The relationship shown in regression equation 7 implies that an increase in solubility, S of the pesticide will result in a decrease in organic carbon based soil water partition coefficient (Koc) (i.e. negative correlation). This means the concentration of a highly soluble pesticide in soil particles will reduce, and a large fraction of the pesticide will be concentrated in water (solution); thereby increasing its leaching potential. Equation 7 is similar to the one obtained by Kanazawa (Log koc = -0.356*logS + 3.01; S in ppm, n = 15, r2 = 0.79, [24]. Equation 7 is also comparable to the ones obtained by Kenaga and Goring (Log koc = -0.55*logS + 3.64; S in mg/l, n = 106, r2 = 0.71, [22] and Mingelgrin and Gerstl (Log koc = -0.58*logS + 4.24 for polar hydrocarbons; S in µmoles/l, n = 15, r2 = 0.410, [25].Legislation in many countries requires extensive testing of all manufactured chemicals for environmental evaluation and registration [31]. Since the solubility (S) of a pesticide is usually provided in the manufacturer’s material safety data sheet, the above relationship can be used to estimate the organic carbon based partition coefficient (koc), and the results compared with values determined experimentally and from other models.
4. Conclusions
The soils around Lake Naivasha had a mean organic carbon (foc) content of 1.770%. The relationship between the organic carbon based partition coefficient (koc) and solubility (S) for the area along the shores of Lake Naivasha was determined to be: log koc = -0.368logS + 3.256.This relationship can therefore be used to estimate the organic carbon based partition coefficient (koc) of a pesticide where S is available, and the results compared with values determined experimentally and from other models.
ACKNOWLEDGEMENTS
This work is based on a PhD research by the corresponding author. The research was financially supported by the German Academic Exchange Service (DAAD).
References
[1] | Charbeneau RJ. Groundwater contaminant transport with adsorption and ion exchange chemistry: Method of characteristics for the case without dispersion. Water Resources Research 1981;17:705-13. |
[2] | Jury WA, Focht DD, Farmer WJ. Evaluation of pesticide groundwater pollution potential from standard indices of soil-chemical adsorption and biodegradation. Journal of environmental quality 1987;16:422-8. |
[3] | Arias-Estévez M, López-Periago E, Martínez-Carballo E, Simal-Gándara J, Mejuto J-C, García-Río L. The mobility and degradation of pesticides in soils and the pollution of groundwater resources. Agriculture, Ecosystems & Environment 2008;123:247-60. |
[4] | Petersen C, Hansen S, Jensen H, Holm J, Koch CB. Movement of suspended matter and a bromide tracer to field drains in tilled and untilled soil. Soil use and management 2004;20:271-80. |
[5] | de Jonge H, de Jonge LW, Jacobsen OH. [14C] Glyphosate transport in undisturbed topsoil columns. Pest Management Science 2000;56:909-15. |
[6] | Banerjee S, Yalkowsky SH, Valvani C. Water solubility and octanol/water partition coefficients of organics. Limitations of the solubility-partition coefficient correlation. Environmental Science & Technology 1980;14:1227-9. |
[7] | Chiou CT. Partition and adsorption of organic contaminants in environmental systems: John Wiley & Sons; 2003. |
[8] | Boening DW. Ecological effects, transport, and fate of mercury: a general review. Chemosphere 2000;40:1335-51. |
[9] | Hemond HF, Fechner EJ. Chemical fate and transport in the environment: Elsevier; 2014. |
[10] | Navarro S, Vela N, Navarro G. Review. An overview on the environmental behaviour of pesticide residues in soils. Spanish journal of agricultural research 2007:357-75. |
[11] | Chiou CT. Partition Coefficient and Water Solubility in Environmental Chemistry. Hazard Assessment of Chemicals: Current Departments 2012;1:117. |
[12] | US-EPA. Agricultural chemicals in groundwater: proposed pesticide strategy, USEPA office of pesticides and toxic substances. U.S. Environmental Protection Agency 1987. |
[13] | Gavrilescu M. Fate of pesticides in the environment and its bioremediation. Engineering in Life Sciences 2005;5:497-526. |
[14] | Kronvang B, Strm H, Hoffmann C, Laubel A, Friberg N. Subsurface tile drainage loss of modern pesticides: field experiment results. Water Science & Technology 2004;49:139-48. |
[15] | USEPA F. A screening model to estimate pesticide concentrations in drinking water. US Environmental Protection Agency, Washington, DC 2008. |
[16] | Moncada J. Spatial distribution of pesticide contamination potential around Lake Naivasha, Kenya. The Netherlands: Enschede; 2001. |
[17] | Arusei M, Sanga J, Tole M. The outflow of Lake Naivasha based on the stable isotope composition of groundwater. Patent NumberUS Patent: 6268508 2003:201-4. |
[18] | Zhou JL, Rowland S, Mantoura RFC. Partition of synthetic pyrethroid insecticides between dissolved and particulate phases. Water Research 1995;29:1023-31. |
[19] | Mekonnen M, Hoekstra A, Becht R. Mitigating the water footprint of export cut flowers from the Lake Naivasha Basin, Kenya. Water resources management 2012;26:3725-42. |
[20] | Kaoga J, Ouma G, Abuom P. Effects of Farm Pesticides on Water Quality in Lake Naivasha, Kenya. American Journal of Plant Physiology 2013;8. |
[21] | Pionke H, Glotfelty D. Nature and extent of groundwater contamination by pesticides in an agricultural watershed. Water research 1989;23:1031-7. |
[22] | Kenaga E, Goring C. Relationship between water solubility, soil sorption, octanol-water partitioning, and concentration of chemicals in biota. Aquatic toxicology 1980;707:78. |
[23] | Gerstl Z. Estimation of organic chemical sorption by soils. Journal of contaminant hydrology 1990;6:357-75. |
[24] | Kanazawa J. Relationship between the soil sorption constants for pesticides and their physicochemical properties. Environmental toxicology and chemistry 1989;8:477-84. |
[25] | Mingelgrin U, Gerstl Z. Reevaluation of partitioning as a mechanism of nonionic chemicals adsorption in soils. Journal of Environmental Quality 1983;12:1-11. |
[26] | Jolicoeur JLC. Groundwater contamination potential of agriculture around Lake Naivasha: Comparison of five different unsaturated soil zone. Enschede, The Netherlands. 2000. |
[27] | Nelson DW, Sommers LE. Total carbon, organic carbon, and organic matter. Methods of soil analysis Part 1996;3:961-1010. |
[28] | Schumacher BA. Methods for the determination of total organic carbon (TOC) in soils and sediments. Ecological Risk Assessment Support Center 2002;2002:1-23. |
[29] | <ACP-EU End of Project Quantitative Survey TOR.pdf>. |
[30] | Blume LJ, Schumacher P, Schaffer K, Cappo K, Papp M. Handbook of methods for acid-deposition studies. Laboratory analyses for soil chemistry. Lockheed Engineering and Sciences Co., Inc., Las Vegas, NV (United States); 1990. |
[31] | Briggs GG. Theoretical and experimental relationships between soil adsorption, octanol-water partition coefficients, water solubilities, bioconcentration factors, and the parachor. Journal of Agricultural and Food Chemistry 1981;29:1050-9. |