Motasem Saidan1, Khaled Rawajfeh1, Manar Fayyad2
1Chemical Engineering Department, University of Jordan, Jordan
2Water Researches Center, University of Jordan, Jordan
Correspondence to: Motasem Saidan, Chemical Engineering Department, University of Jordan, Jordan.
Email: |  |
Copyright © 2012 Scientific & Academic Publishing. All Rights Reserved.
Abstract
Total Trihalomethanes (THMs) content can vary greatly, depending both on water quality and on treatment conditions, in the same water supply system. Most municipal water treatment plants use chlorine to keep water biologically safe during the distribution process. The effect of various parameters on the formation of THMs has been studied in this investigation using water samples collected from Zai water treatment plant in Jordan. These parameters are reaction time, temperature, pH, TOC, free chlorine, and bromide ion content. An increase in the value of any of these parameters has positive effects on the formation of THMs. A general correlation for the prediction of THMs as a function of these parameters is proposed. Its predictions are in good agreement with the observed results regardless of the fact that it is restricted to this study. The kinetics of THMs formation were also investigated and found to follow first order kinetics with 0.0022 hr-1 rate constant.
Keywords:
THMsFormation, Chlorination, Drinking Water, Water Quality Model
Cite this paper: Motasem Saidan, Khaled Rawajfeh, Manar Fayyad, Investigation of Factors Affecting THMs Formation in Drinking Water, American Journal of Environmental Engineering, Vol. 3 No. 5, 2013, pp. 207-212. doi: 10.5923/j.ajee.20130305.02.
1. Introduction
Chlorine, while an effective disinfectant, reacts with organic materials that might be present in the source drinking water to produce a group of chlorinated organic compounds known as Disinfection Byproducts (DBPs)[1, 2]. The DBPs include THMS (chloroform, bromodichloromethane, dibromochloromethane, and bromoform). The presence of THMs in drinking water has been the subject of increasing public concern since the 1970s[3]. Toxicological studies suggested that THMs have been linked to the occurrence of human cancer in many instances in 1976. This was soon followed by an act by the Environmental Protection agency (EPA) to regulate their concentrations in drinking water. In the past, various investigations had been conducted to determine factors that might influence THMs formation[4]. From the mid of 1980s, several models simulating the propagation and transformation of contaminants in a distribution system over time were developed and applied[5, 6]. In spite of water treatment, finished water may sustain substantial changes in quality while being transmitted through the distribution system before reaching the consumer’s tap. These changes may be associated with complex physical, chemical and biological processes that take place during transport. Statistical correlations has been developed to predict the concentration of chloroform formation as function of pH, temperature and distance (near or far) between a reservoir and a point of interest in the distribution system. This correlation is qualitative in the sense that distance and time are not included implicitly in it[7]. Multiple regression model has been generated for predicting THMs level in the finished water leaving a treatment plant. The correlation implies that THMs formation is higher in summer through autumn and lower in winter through spring[8].Mathematical model that applies second order kinetics has been proposed to predict the chlorine residuals and demonstrates that THMs formation can be characterized as a function of chlorine demand. The parameters of this model are correlated as a function of pH, TOC, temperature and chlorine residual[9].The formation of THMs in drinking water has been shown to be a function of various water quality parameters and chlorination conditions. THMs formation is one of the slowest known reactions in water treatment and a number of important factors influence this reaction. These include residence time, pH, temperature, bromide ion concentration, chlorine dosage, type of organic precursor and the Total Organic Carbon (TOC).In the present paper, the overall objective is to develop an improved understanding of the effect of water parameters on THMs formation in drinking water, and also develop a mathematical model for the prediction of THMs concentrations for Zai water treatment plant.
2. Materials and Methods
The experiments in this study were conducted on samples taken from Zai water treatment plant located near the capital Amman in Jordan. The procedure is based on collecting 2 liters samples from the feed and the effluent streams of the plant. The samples were stored at a given constant temperature. For THMs measurement smaller samples were taken from the original sample in 40 ml septum vials containing few crystals of sodium thiosulfate (sufficient to eliminate any residual chlorine and thus stop the trihalomethane formation reaction), filled to zero head space, and stored in the dark at the desired experimental conditions prior to analysis. The THMs were measured using Head Space Analyzer GC with ECD.The experimental conditions under which the effect of each parameter was studied were fixed as follows maintaining the conditions for other parameters fixed at some predetermined values.
2.1. Effect of Reaction Time
The concentrations of THMs were determined after the following reaction times have elapsed: 0, 1.25, 5.25, 10.0, 18.0, 23.0, 29.25, and 49.25 hr. The other parameters were fixed at: temperature 16℃, pH 7.8, TOC 1.14 ppm, free chlorine 1.04 ppm, and bromide ion 0.42 ppb. Trihalomethanes formation potential was determined after 168 hr (seven days).
2.2. Effect of Temperature
The study of the effect of temperature on the formation of THMs was carried out at temperatures of 5, 14, 28, and 40℃. The other parameters were fixed at: TOC 0.829 ppm, pH 7.71, free chlorine 1.2 ppm, reaction time 3 hr, and bromide ion 0.5 ppb.
2.3. Effect of pH
The pH was checked and changed for each sample by the drop-wise addition of 0.1 M HCl or 0.1 M NaOH. The pH values used in this part of the investigation were: 6.04, 7.15, 7.71 and 8.7. The pH was measured using an ORION 960 Automatic System, with pH electrode (model No. 9172 BN). The other experimental conditions were fixed at temperature 20℃, TOC 0.829 ppm, free chlorine 1.15 ppm, reaction time 2.5 hr, and bromide ion 0.5 ppb.
2.4. Effect of Chlorine Dose
The residual chlorine checked and changed for each sample by the drop wise addition of standard chlorine solution prepared from 6% sodium hypochlorite solution. The DPD-colorimetric method was employed for residual chlorine measurement.The concentrations of THMs were determined at the following conditions: temperature 14℃, TOC 0.829 ppm, pH 7.71, reaction time 1.5 hr, and bromide ion 0.5 ppb, and at the following chlorine residuals values (1.2, 1.75 and 2.88 ppm).
2.5. Effect of TOC
In order to study the effect of TOC different values in the lab, drops of influent water were added to the effluent water to achieve the required TOC concentration for each final sample. The following TOC values: 1.33, 1.82, and 2.04 mg/l, were considered in this study. The TOC analyzer employed was a DC-180 (3300) (Dorhman/ Rosemount) with Non Dispersive Infrared Detector (NDIR).With these TOC levels, the concentrations of THMs were determined for water samples under the following conditions: reaction time 2 hrs, temperature 15℃ and bromide ion concentration of 0.55 ppb. In these experiments there were very slight variations in pH and chlorine residuals for the three samples. These changes in pH are due to the presence of organic acids resulting in pH values of: 7.84, 8.01, 7.88. Rapid chlorine decay reaction resulted in different levels of chlorine: for chlorine: 0.84, 0.7, 0.6 ppm. For those reasons an average pH value of 7.91 and an average chlorine residual of 0.74 mg/l were taken for those samples.
2.6. Effect of Bromide Ion
The effect of the presence of bromide ions on the formation of THMs was demonstrated by measuring the bromide concentration in water samples using an ion chromatographic method. A DX-120 Ion Chromatograph (DIONEX) with chemical suppressor for anions and conductivity detector was used for this purpose.
3. Results and Discussions
3.1. General Correlation
In order to describe the mechanisms of THM formation, several empirical models have been proposed[7, 8, 10]. The models developed range from over all reaction stoichiometry expressions to empirical equations derived from linear and nonlinear statistical regression analysis. These equations predict the concentration of THMs as function of the various parameters under consideration.In this study the following predictive equation is proposed:
in which:
The parameters of this equation were determined by applying standard regression techniques to the experimental data obtained in this study. The goodness of fit of the data predicted by this correlation to experimental data is demonstrated in Figure 1, with 0.982 coefficient of correlation. However, this predictive equation is only applicable to this study or any other study with the same conditions. This was concluded after many trials to apply this predictive equation to another data from different country. 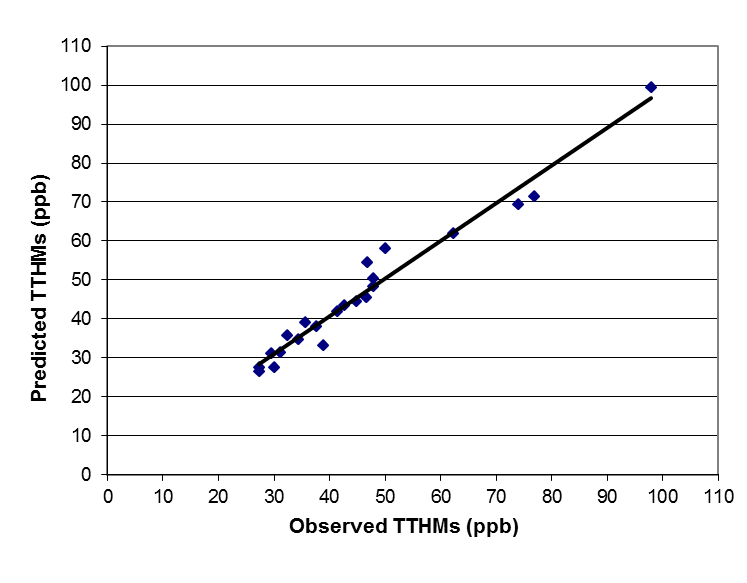 | Figure 1. Comparison between THMs observed and correlated data |
3.2. Effect of Residence Time
The formation of THMs under natural conditions is not instantaneous. Under some reaction conditions the formation of THMs may be completed in less than an hour, in other circumstances, several days may be required before the maximum yield of THMs occurs.Concentration levels of THMs formed after chlorination of drinking water issuing from Zai water treatment plant at various residence times are presented in Figure 2. It is seen that THMs concentrations increase as the residence time extends from 1.25 hr to 49.25 hr. The increase is initially rapid slowing down as time goes on. This trend resembles a first order behavior. The figure also illustrates the good agreement between experimental and predicted data.Analytical kinetic models of THMs formation have not yet been developed, mainly because of the complexity of the reactions of chlorine with organic materials in water. The consumption of chlorine in water occurs through complex sequential and parallel reaction pathways with unknown rates[11].It was proposed in the EPA model that THMs formation follows first order growth limited reaction, for which the trihalomethane formation potential (CFP) is the limiting concentration to which THMs can grow.This model is given by:
which upon integration yields the following equation:
where:
This equation can be rearranged into the following form: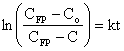
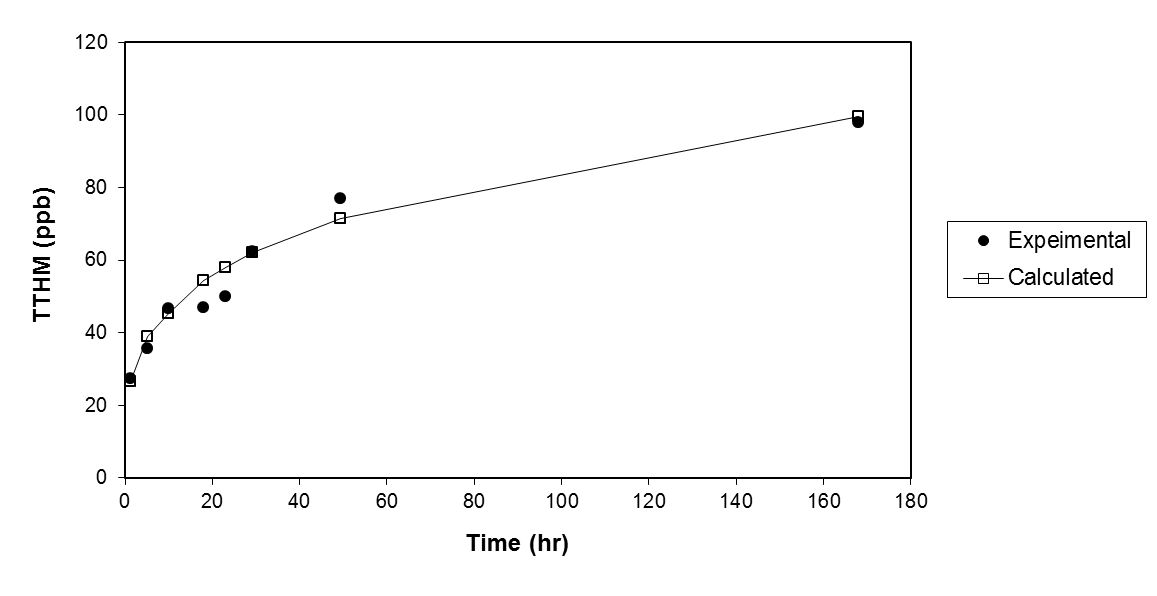 | Figure 2. Effect of reaction time on THMs formation |
This is a straight line equation passing through the origin and its slope is the rate constant k. A plot of the left hand side of this equation based on experimental data versus time is shown in Figure 3. It is very clear that the data obtained in this study follow first order kinetics since the plot gives a straight line. The rate constant k is found using standard regression methods to obtain the slope of this line. The rate obtained is 0.0022 hr-1.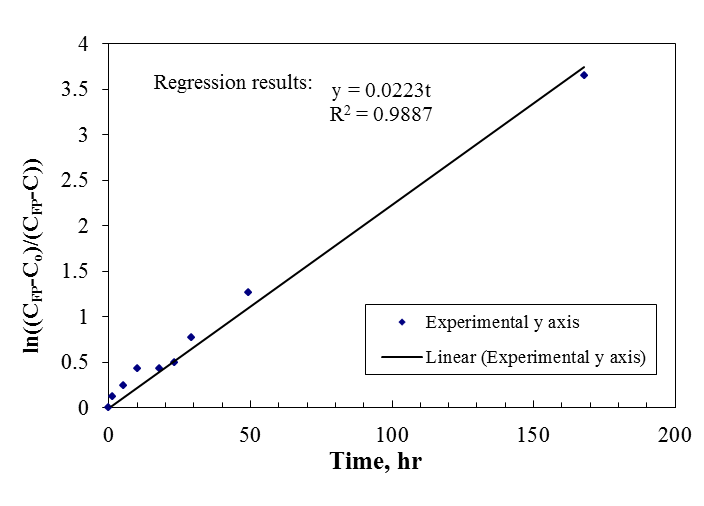 | Figure 3. First order behavior of THMs formation |
3.3. Effect of Temperature
Data collected by the Water Authority of Jordan[12] showed that THMs concentration varied seasonally and were lower in the winter and higher in summer due to the effect of temperature variation from winter to summer. With chlorination of natural water, approximately twice as much of chloroform can be formed during a given period of time at 25C as is formed at 3C[13]. Increasing the temperature has a positive effect on THM formation as shown in Figure 4. It is also seen that this trend is very well predicted by the correlation proposed in this study.
3.4. Effect of pH
The reaction sequence of THMs formation suggests that the pH would have a strong effect on THMs formation. This effect has been illustrated by a number of researchers[10, 11, 13].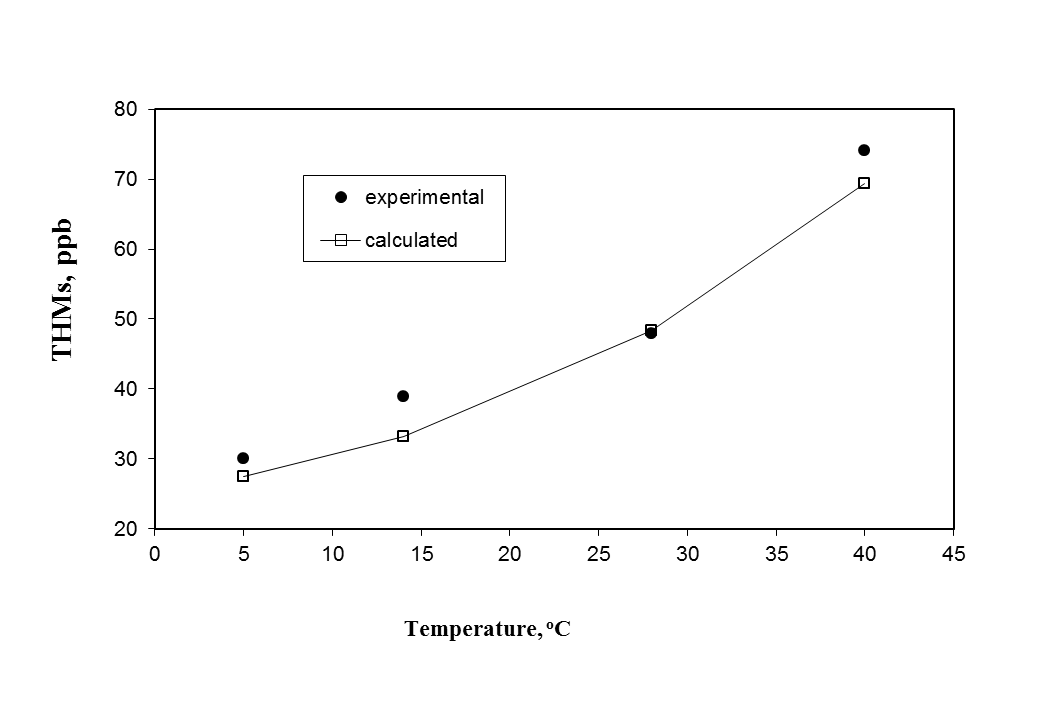 | Figure 4. Effect of temperature on THMs formation |
It is demonstrated that a large increase in the reaction rate occurred at pH values from 8-10; however, this effect was weakly pronounced in the range 6-8. He illustrated the increase at the high pH values was the result of increased formation of phenoxide ions[13]. According to the previous studies, the results also showed that the reaction rate of THMs progressively increased as the pH increased from 6 to 9[10].In the present study, it is observed that THMs concentrations increase with increasing pH as shown in Figure 5. There again the good agreement between predictions and experimental data is shown on the same figure.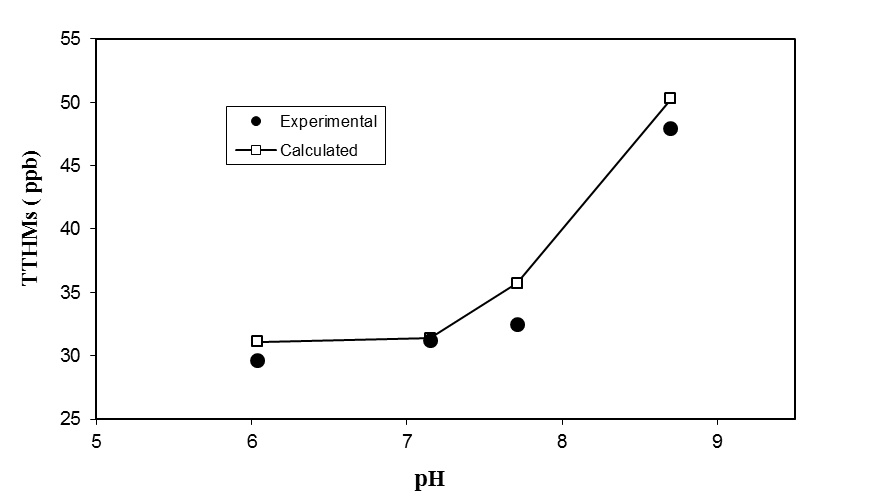 | Figure 5. Effect of pH on TTHMs formation |
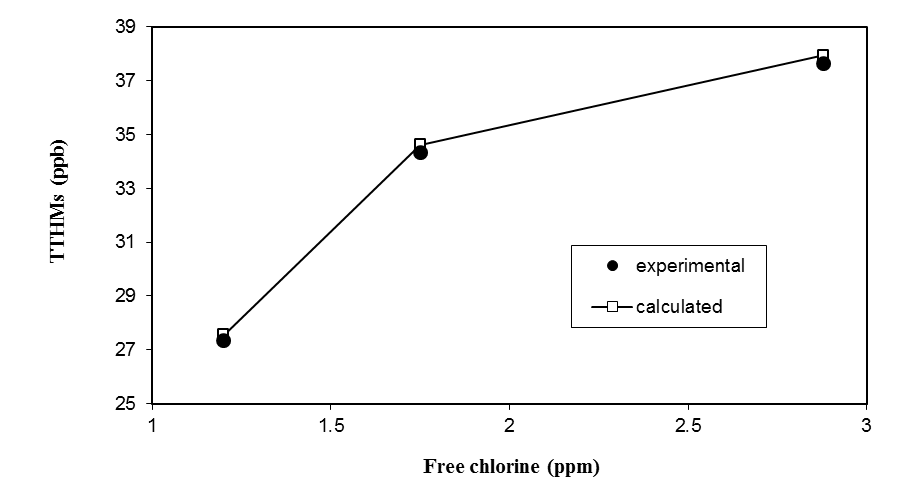 | Figure 6. Effect of free chlorine on TTHMs formation |
3.5. Effect of Chlorine Dose
The chlorine reaction with THMs precursors are described as a three-step reaction[14]. The first step was where immediate chlorine demand was exerted. Typically this is due to the reaction with an inorganic rather than an organic compound. Once the immediate demand is satisfied, the additional chlorine begins to react with any available organic material, including THM precursors. They showed that in this step there was a nearly linear relationship between chlorine dose and the concentration of THMs produced. When enough chlorine was consumed satisfying both the immediate and short-term organic chlorine demands, a long-term chlorine residual is obtained, and any further increase in THM formation with chlorine dosage is small. Moreover, according to the literature, the rate of THMs formation is strongly dependent on the applied chlorine does, i.e. it increases with increasing chlorine dose[11]. As shown in Figure 6, it is noticed that, as expected, the THMs concentration increased as a function of the applied free chlorine dose. Also, the figure shows the predicted results as compared to the experimental data.
3.6. Effect of Total Organic Carbon
Trihalomethanes are formed according to the following generalized equation[15, 16, 17]:
The formation of THM is strongly dependent on the amount of naturally present humic substances (Humic and Fulvic acid); generally referred to as Total Organic Carbon (TOC), i.e. it increases with increasing TOC in presence of free chlorine residuals.As shown in Figure 7, it is seen that THMs concentration increases almost linearly with TOC concentration. This trend is very well followed by the predictive equation as shown in the figure.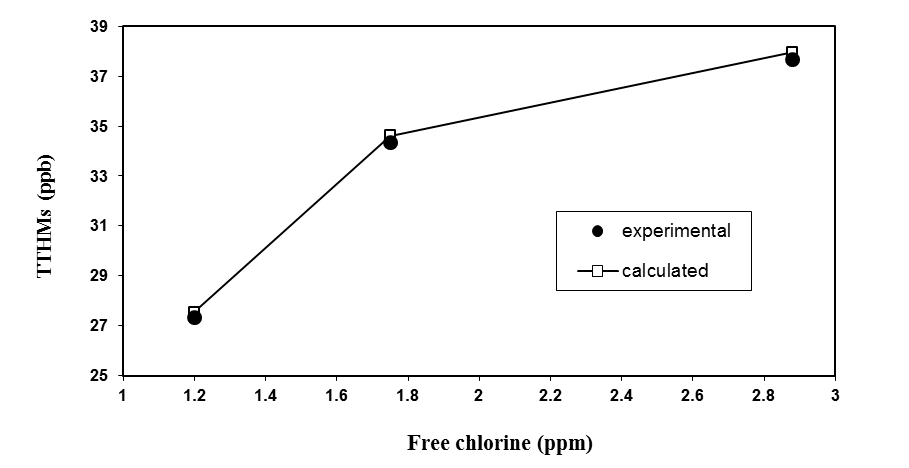 | Figure 7. Effect of TOC level on TTHMs formation |
3.7. Effect of Bromide Ions
Both chlorine and bromine are oxidants and substitution agents. Chlorine being a better oxidizing agent; while bromine being a better substitution agent. Moreover, the kinetics of bromine substitution is more rapid than those for chlorine substitution. This is why bromide ions were found to be good precursors in the formation of brominated THMs in the presence of chlorine (even with the presence of substantial amounts of it). Bromide ions are oxidized to bromine (hypobromous acid) which participate in the halogenation step in the THMs formation reaction sequence much more effectively than does chlorine.The results of the measurements made to reveal the effect of the presence of bromide ions on the THMs formation in the Zai water treatment plant are shown in Figure 8. They clearly indicate that the presence of bromide ions increased the formation of brominated THM species[18]. Bromoform is by large the dominating halogenated methane.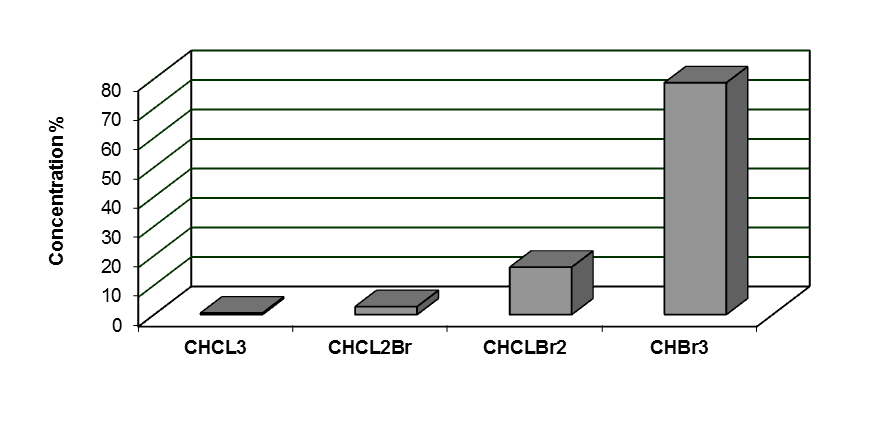 | Figure 8. Effect of bromide ion presence on THMs types formation |
4. Conclusions
The effect of several parameters on the formations of THMs from chlorination of water from Zai water treatment plant was investigated in this study. The results of this investigation indicated that increasing the residence time, temperature, pH, free chlorine, and TOC (humic substances) enhanced and increased the THMs formation. Specifically, the yield of brominated–THM species increased with the presence of bromide ions in the chlorinated drinking water, mainly the bromoform. The kinetics of THMs formation were investigated and found to follow first order kinetics with 0.0022 hr-1 rate constant.It is shown in this study that the factors affecting the formation of THMs can be related by simple regression correlation based on experimental data. The correlation proposed in this study satisfactorily predicted the THMs formation as function of the parameters under investigation. The correlation coefficient for the measured and predicted values was found to be 0.982.
References
[1] | Richardson, S. D. (2011) Disinfection by-products: formation and occurrence of drinking water. In: Nriagu, J.O. (Ed.), the Encyclopedia of Environmental Health, vol. 2. Elsevier, Burlington, pp. 110-136. |
[2] | Brunet, R., Berne, F., De Laat, J. (2010) Disinfection by-products in chlorinated swimming pool waters (Sous-produits de chloration dans les eaux de piscines publiques). L’Eau, L’Industrie, Les Nuisances 333, 83-88 (in French). |
[3] | Steve E. H. (2009) Chlorination disinfection by-products, public health risk tradeoffs and me, Water Research, 43, 2057 – 2092. |
[4] | Richardson, S.D. (2005) New disinfection by-product issues: emerging DBPs and alternative routes of exposure, Global Nest J., 7 (1) 43–60. |
[5] | Grayman, W.M., Clark, R.M., and Males, R.M. (1988) Modeling Distribution-System Water Quality: Dynamic Approach. Jour. Water Resour. Plng and Mgmt. ASCE, 114(3): 295-312. |
[6] | Liou, C.P. and Kroon, J. R (1987) Modeling the Propagation of Waterborne Substances in Distribution Networks. Jour. AWWA, 79(11) : 54-58. |
[7] | Garcia-Villanova, R.J. et al. (1997) Formation, Evolution and Modeling of THMs in the Drinking Water of a Town: I. at the Municipal Treatment Utilities. Wat, Res., 31(6): 1299-1308. |
[8] | Golfinopoulos, S. K., et al. (1998) Use of a multiple Regression Model for Predicting THM formation. Wat. Res., 32(9): 2821-2829. |
[9] | Clark, R.M. (1998) Chlorine Demand and TTHM Formation Kinetics: a Second-Order Model. Jour. Environ. Eng. ASCE, 124(1): 16-24. |
[10] | El-Dib, M.A., and Ali, R.K. (1995) THMs Formation During Chlorination of Raw Nile River Water. Wat. Res., 29 (1): 375-378. |
[11] | Kavanaugh, M.C. (1980) An Empirical Kinetic Model of THM Formation. Applications to Meet the Proposed THMs Standard. Jour. AWWA. , 72, 578, October. |
[12] | Garber, A. and Salameh, E. (1991) Jordan's Water Resources and Their Future Potential, Amman, Jordan. |
[13] | Rook, J. J. (1979) Haloforms in Drinking Water. Jour. AWWA, 68, 1680, |
[14] | Trussell, R.R., and Umphres, M.D. (1978) The Formation of Trihalomethane. Jour. AWWA, 70(11): 604-612 |
[15] | Edzwald, J.K. (1987) Coagulation-Sedimentation-Filtration processes for Removing Organic Substances from Drinking water. In: Control of organic Substances in water and wastewater, pollution Technology review No. 140, Noyes Data Corporation. USA, New Jersy,: p.p. 26-64. |
[16] | Vasconcelos, J.J., and Boulos, P.F. et al. (1996) Characterization and Modeling of Chlorine in Distribution Systems, AWWA Research Formation, Denever. |
[17] | Morris, J.C. and Baum, B. (1977) Precursors and Mechanisms of Haloform Formation in the Chlorination of Water supplies. Presented at the second Conference on Water Chlorination: Environmental Impact and Health Effects, Gatlinburg, Tenn., Oct.31- Nov.4. |
[18] | Toroz, I., and Uyak, V. (2005) Seasonal variations of trihalomethanes (THMs) within water distribution networks of Istanbul city, Desalination 176 , 127–141. |