E. A. Gomaa , B. M. Al-Jahdalli
Chemistary Department; Faculty of Science; Mansoura University; 35516 Mansoura; Egypt
Correspondence to: E. A. Gomaa , Chemistary Department; Faculty of Science; Mansoura University; 35516 Mansoura; Egypt.
Email: |  |
Copyright © 2012 Scientific & Academic Publishing. All Rights Reserved.
Abstract
On using conductometric technique, the apparent association constant (KA) of Cu (NO3)2 were measured in mixed MeOH-DMF mixed solvents at 0, 20, 40, and 100% MeOH (by volume) and different temperatures (298.15, 303.15, 308.15 and 313.15K) in absence and presence of Kryptofix - 222[ 4, 7, 13, 16, 21, 24 – hexaoxa – 1.10 – diazo – bicycle – (8, 8, 8) Hexa cosane]. From the experimental results, the molar conductivities (∧) were calculated and limiting molar conductivites (∧0) were calculated by using Shedlovsky and Fouss – Kraus extrapolation methods. The molar solvated (∨), Van der Waals (∨w), electrostriction (∨e) and apparent molar () volumes were evaluated. The free energy of association (), free energy necessary for complexation (), enthalpy () and entropy () were determined. New equation for the calculation of association constant were used and all the results were discussed.
Keywords:
Kryptofix- 222 – Cu (NO3)2 – (MeOH - DMF) solvents, molar conductance, limiting molar conductance, molar solvated volume, Van der Waals volume, electrostriction volume, apparent molar volume, free en
1. Introduction
Although alkali and alkaline earth metal cations play an important role both in chemistry and in biology, the coordination chemistry of alkali and alkaline earth metals was completely ignored by chemists. However, the coordination chemistry of alkali and alkaline earth cations has mainly developed by the synthesis of crowns by Pedersen [Pedersen.., (1967)][1].Copper is an important trace element which plays an important role in human's body. It's essential for haemoglobin synthesis and iron metabolism. Copper is associated with number of metalloproteinase (Copper contain enzymes such as tyrosinase, Lysol oxidase, cytochrome oxidase and ceruloplasmin ). The major function of metalloproteinase involved in the oxidation-reduction reactions. When the activity of copper enzymes decrease, number of pathological conditions will appear such as decrease tyrosine activity leads to failure of pigmentation, decrease of Lysol oxidase activity leads to defects in connective tissue cross linking and also decrease of cytochrome oxidase activity leads to ataxia. The adult human body contain between 80-150 mg/day. Copper deficiency in infants has been observed in prematurity malnutrition, malabsorption, chronic diarhea and prolonged feeding with low total milk diets. The susceptibility of premature infants to copper deficiency is related to their lower stores of liver and spleen copper. Long term hyperlimentation produce copper deficiency in both infants and adults. The symptoms of cu deficiency infants are neutropenia, hypo chromic anaemia in the early stage which is responsive to oral copper. Also, osteoporosis, decrease the pigmentation of the skin, lowered plasma copper, ceruloplasmin, Anaemia, heart disease, abnormal electrocardiograms, hyper cholesterolemia and hyper tension caused due to cu-deficiency in adults and infants. The treatment using copper supplementation for adult between 0.5-1.5mg/day.The multidentate macromolecules (MMM) which have been studied as ligands for M+z, were included natural antibiotics and synthetic compounds such as crown and cryptands [Pornia..][2].The antibiotics could be cyclic or acyclic; where as the synthetic ligands could be acyclic, monocyclic or polycyclic. The macromolecular ligands had recently became more important to the chemistry of Mz+ than the conventional ligands, this was primarily because they binded Mz+ effectively and rendered the latter soluble in non polar solvents and secondly because they were relevant to chemistry of Mz+ in biological systems which there in involved essentially the macrobiomolecules.The strong (Mz+-MMM) interaction was due basically to the multichelate effect. The discovery of the crown ethers soon followed by synthesis of macrobicycilc polyethers containing three polyether strands joined by two bridgehead nitrogens [Lehn et. al][3]. These compounds have three-dimentional cavities which can accommodate a metal ion of suitable size and form an inclusion complex. These ligands which developed by Lehn and his- coworkers [Dietrich et al], [4] Were called (2)- cryptands where (2) indicates the bicyclic ligand such as Kryptofix- 222 which its structure is given in Fig(1).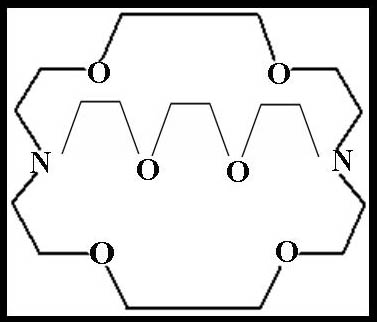 | Figure 1. Kryptofix – 222 [4, 7, 13, 16, 21, 24,- hexa–oxa–1,1o–diazo– bicyclo–(8 ,8 ,8) Hexacosane] |
The crown compounds and their thia- and aza- derivatives have a considerable interest in terms and their complexation properties in solutions with univalent and bivalent metals [Miolgley……][5, 6]. It is important to mention that the macrocyclic crown ethers have many applications [Izatt et al][7]. in biological activity, corrosion chemistry, analytical chemistry, phase-transfer catalysis and industrial production such as nuclear energy, electronics and electrochemical photosensitive materials [El-Dossouki][8].On understanding the interactions between macrocyclic crown ether such as Kryptofix-222 and metal cations in solution, it requires the study of various parameters governing these interaction. A conductance study of the interaction between CO2+,Ni2+,Cu2+Cd2+,Zn2+and Pb2+ ions with cryptand-211, C-221and C-222in different mixtures was carried out at various temperatures by [Shamsipur][9]. The formation constants were determined from the molar conductance-mole date. The conductometric measurement is one of the most suitable methods for the study of ion associations in the solutions of low dielectric constants. The observed association constant values are known to be composite quantities depending on specific and non-specific solute- solvent interactions. The separation of various interaction contributions is often very difficult process, beside that using mixed solvents it add another dimension to the problem [Mukhopadhyay et al][10]. The thermodynamic studies of these interactions give important information about their complexation reactions and the selectivity of these legends towards different metal cations [Rouraghi][11]. The enthalpy and entropy data of the cryptate formation reactions were determined from the temperature dependence of the formation constants.Sway and his co-workers [12] studied the molar conductance of the complexes between ligand with Mg2+,Ca2+,Sr2+ and Ba2+ ions in MeOH-DMF. The observed molar conductance (∧) was decreased for mole ratios less than one. The conductance date was analyzed using a model involving (1:1) complexation stoichiometry to give the stability constant (K) and limiting molar conductance (∧o) for each (1:1) complex by using a nonlinear least squares analysis. Wenz and his co-workers[13]studied the thermodynamics of the complexation of some monovalent and bivalent cations with crown ethers and with cryptands in N,N-dimethyl formamide (DMF).The aim of the present work is to study the conductivity of Cu (NO3) the absence and in the presence of Kryptofix- 222 using different molar ratios MeOH-DMF solvent at different temperatures (298.15, 303.15,308.15 and 313.15K). By applying Shedlovsky, Fouss- Kraus extrapolations methods, we were able to evaluate the values of (∧o), (KA) and ( ΔGA ) and to make an acceptable discussion. Finally, the crystal and molecular structures of about 200 metal halide complexes with oxygen-containing crown ethers were investigated by Bel'sky[14]. The systems considered were contained actinide halides and halides of the (IV) row elements. The characteristic features of the formation of these complexes and their coordination fragments were discussed.
2. Experimental
The aza-crown ether, Kryptofix-222 [4,7,13,16,21,24- hexaoxa-1.10-diazo-bicyclo-(8,8,8) Hexacosane] was supplied from Merck Co.Cu(NO3)2 of high grade was supplied from MeOH-MDF and it was used without any further purification. The water content of salt was determined by using (Mettler DL-18) Karl-Fisher titrator and it was found to be less than 0. 01% Methanol (98.8%) was a DMF supplement and the water used was secondly distilled one. All the conductometric titrations were manipulated and were done using 1x10-5 mol/L Cu(NO3)2 and 1x10-4 mo/L Kryptofix-222 as initial concentrations.In general, densities of the saturated solutions of Cu (NO3)2 were measured by using a weighing bottle (1ml) with a capillary cover. The viscosities of the solutions were measured by the flow of each solution in a viscometer of a very fine capillary recalibrated by means of water.The different saturated solutions of Cu (NO3) MeOH- DMF in different volume proportions were prepared by shaking 10ml of each of the mixed solution in a glass test tube containing excess solid Cu (NO3)2 and locating in a thermostatic water bath of the type (Assistant – 3193) for a period of one week. Dry nitrogen gas was passed through each of these solutions to ensure that these media were completely inert. The molal solubilities of Cu(NO3)2 in these different mixed solutions with its different volume proportions, were determined gravimetrically by using a precipitation titration methods (Mohr's and Fajan's methods) using back titration of excess EDTA with MgSO4 and EBT as indicator (buffer solution of a pH=10). The experimental values of the molal solubility (S) reported in this work were average of these separated determination.The specific conductivity (Ks) of Cu (NO3)2 solution in presence of Kryptofix- MeOH-DMF solvent were achieved at different temperatures (298.15, 303.15, 308. 15,313.15K) using conductometer of the type (Beckmann conductivity Bridge Model No (RE-18A). The conducto- meter was connected with an ultra- thermostat of the type Kattermann- 4130. Spectrophotometrical continuous variation study of Cu (NO3) the presence of Kryptofix-222 at different temperatures and in (0,20,40, 100%) MeOH was achieved using Unicum UV-2-100 UV/visible spectrometer v 3.32; at a wave length of
max (284n.m).
3. Results and Discussion
The solubilities (S) of Cu(NO3)2 in MeOH-DMF were determined from the experimental results in molal scale. From the density measurements of Cu(NO3)2 in (MeOH-DMF) mixed solvents, the molar volume (V) were calculated at different temperatures by using equation(1). | (1) |
when
x1, mole fraction of MeOHx2, mole fraction of DMFd, density of solutionsand their value are listed in Table (1). The packing density (P) as reported by Kim[15] i.e the relation between Van der Waals volume (Vw) and the molar volume (V) of relatively large molecules was found to be a constant value and equal to 0.661 i.e. | (2) |
The electrostriction volume (Ve) which is the volume compressed by the solvent can be calculated by using equation (3) as follows. | (3) |
The apparent molar volume
can be calculated at different temperatures by using equation. (4) [Grzybkowski...] [16]. | (4) |
Where ms, concentration =8x10-5The apparent molar volume at infinite dilution
was obtained by extrapolating the relation between
and
by using equ. (5) see fig (2).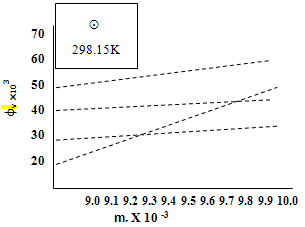 | Figure 2. Variation of the apparent molar volume фv and molarity ms at different temperature of Cu(NO3) the presence of Kryptofix-222 |
 | (5) |
Where,
is the intercept and Sv the slope.The solvated radii (rs) of Cu (NO3)2 were calculated by adding the crystal radius to the radii of solvent by using equ. (6) [Gomaa …..][17].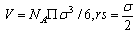 | (6) |
Where, NA is Avogadro's number (6.02x1023) and
is the solvated diameter of solute.The specific conductance (Ks) values at different concentrations of Cu (NO3) MeOH- DMF in the presence and in absence of Kryptofix-222 were measured experimentally and from which the molar conductance (∧) were calculated [Gomaa 18] by using equ. (7).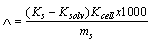 | (7) |
Where (Ks) and (Ksolw) are specific conductances of the solution and the solvent, respectively; (Kcell) is the cell constant and (
) is the molar concentration of Cu (NO3)2. The limiting molar conductance (∧o) of the solution were obtained by extrapolating the relations between (∧) and
of Cu (NO3)2 in the presence and in the absence of Kryptofix-222[16].The values of limiting molar conductances (∧o) for each stiochiometric complex were obtained by extrapolating experimental (∧) values vs.
of Cu (NO3)2 to zero concentration for each line. The experimental data of (∧) and (∧o) were analyzed firstly by using Shedovsky and Fuoss-Kraus extrapolation method [Kappenstein …….][19] to estimate (KA) of Cu (NO3) the presence and absence of Kryptofix-222. The above mentioned method has been mentioned earlier in Dash's publication [20].It is given by the equations from (8). to (10).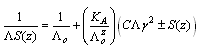 | (8) |
Where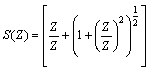
 | (9) |
Where S = a∧o+b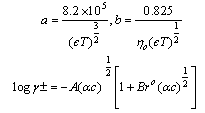 | (10) |
Where
and (A) , (B) are the Debye- Huckel constants, (rº) is the ion size parameter,
are the viscosity and the dielectric constants of MeOH-DMF respectively. The new equation for calculation of association constant (KA) at different concentration of Cu (NO3)2 in MeOH-DMF in the presence and in the absence of Kryptofix -222 were derived as equ. (11).
Where, 
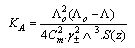 | (11) |
The linear plot of log
o vs 1/T of Cu (NO3) the presence and in the absence of Kryptofix-222; activation energy (Ea) values could be evaluated where the slope =
by using equ. (12). See Fig (3) and Table (2).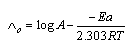 | (12) |
Table 1. The values of d,do,ms, , V,VW Ve, , ,rs of Cu (NO3)2 in (MeOH-DMF) at different temperatures 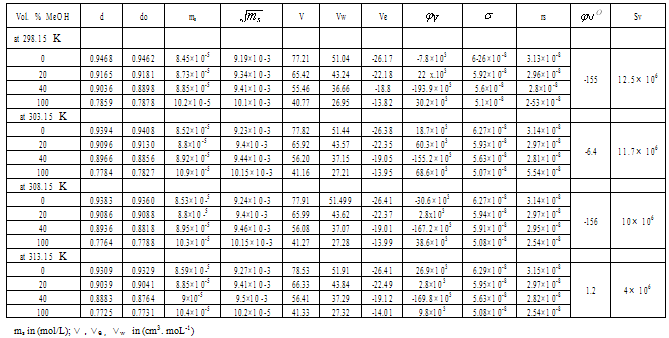 |
| |
|
Table 2a. The values of cm, ^, ^∘ , ɤ + , S(Z) , α of Cu (NO3)2 in MeOH /DMF in presence of Kryptfix-222 at different temperatures 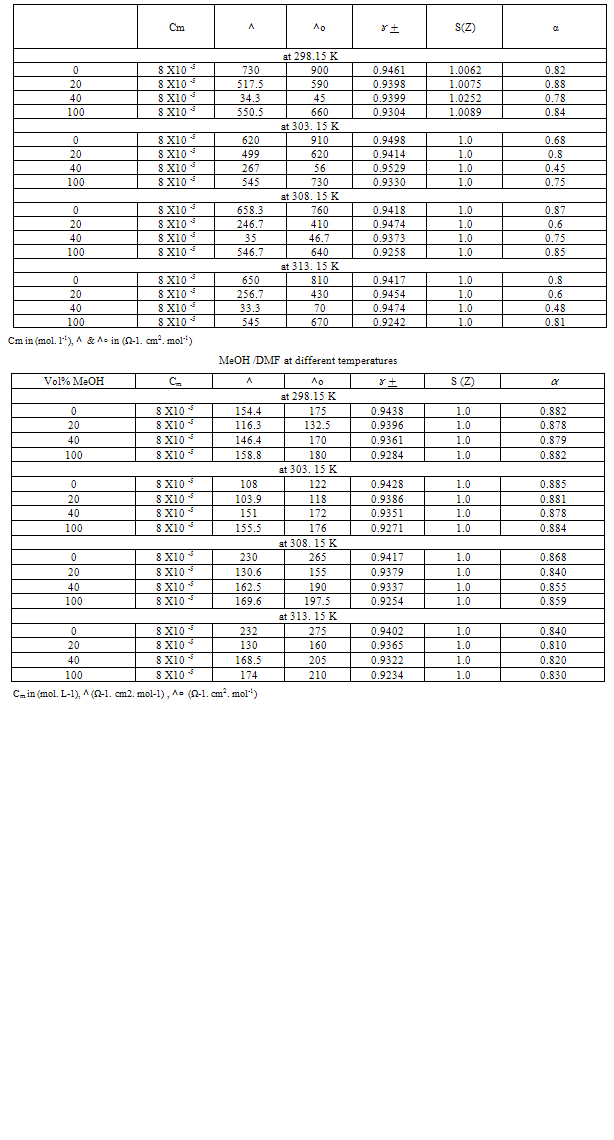 |
| |
|
Table 3a. The values of K A.cal , KA(F-Sh) =KA (Fuoss – Shedlovsky), KA (F-K) = KA (Fuoss – Kraus), ∆G ligand, ∆G complex, ∆HA, ∆SA, Ea of Cu (NO3)2 in MeOH /DMF 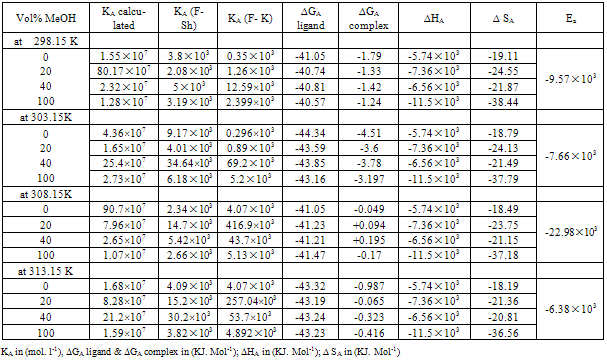 |
| |
|
Table 3b. The values of KA calculated, ∆GA nonligand, ∆HA, ∆SA, Ea of Cu (NO3)2 in MeOH /DMF at different temperatures. 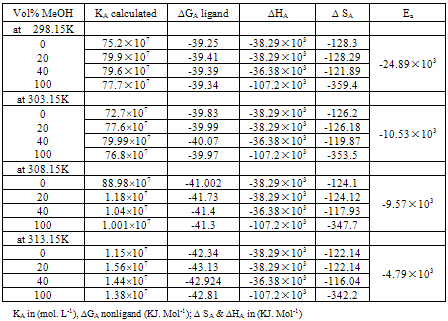 |
| |
|
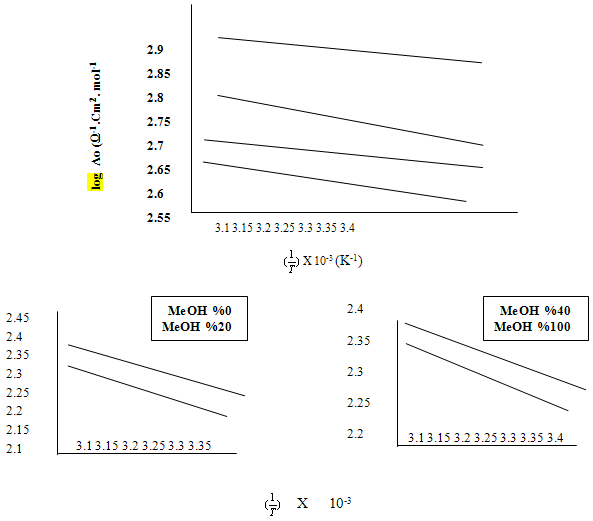 | Figure 3b. Variation of log limiting molar conductance in mixed (MeOH-DMF) of Cu(NO3)2 in the presence of Kryptofix-222 |
The Gibbs free energies of association (ΔGA)values of Cu (NO3) the presence and in the absence of Kryptofix-222 can be calculated at different temperatures by using equ. (13),(14).respectively.  | (13) |
 | (14) |
The Gibbs free energies of necessary for complexation (ΔGA complex) values of Cu(NO3)2 in MeOH/DMF with Kryptofix -222 at different temperatures can be calculated by using equation (15) [Kappenstein…..][19]. See Table (3). | (15) |
The linear plot of log KA vs. 1/T of Cu(NO3) the presence and in the absence of Kryptofix-222, the enthalpy change (ΔGA) could be obtained, where the slope equal
We can also determine the entropy change (Δs) of Cu(NO3)2 in the presence and in the absence of Kryptofix-222 at different temperatures [Gomaa...][20,21].By using eg. (16), (17) respectively. | (16) |
 | (17) |
The plot of Gibbs free energies association (ΔGA) in the presence of Kryptofix-222 vs mole fraction of MeOH (Xs) at different temperature see Fig (5) and Table (3).
4. Conclusions
It was concluded that the KA for Cu (NO3)2 with Kryptofix-222 increase with increase of temperature and the mole fraction of MeOH in the mixed MeOH-DMF solvents due to the increase of ion-ion and ion-solvent interactions (ionic solvation).It was also concluded that all the volume values increase with increase of temperatures and MeOH content favouring also more association and interactions.
References
[1] | Pederson C.J , Am. Chem. Soc. 89(1967) 7017. |
[2] | Poonia N.S. and AV Baja, Coordination Chemistary of Alkali and Alkaline Earth cations, chem. Rev, 79 (1979) 389. |
[3] | Lehn J.M and Sauvage J.P , Chem. Comm., (1971) 440. |
[4] | Dietrich B; Lehn J.M and Sauvage J.P, Tetrahedron Lett., 2885(1969) 2889. |
[5] | Maiolgley D. Chem. Soc. Rev. 4(1975) 549. |
[6] | Gokel G.W. and Drust H.D. (1976) Synthesis, 168. |
[7] | Izatt R.M., Izatt N.E.; Rossiter B.E; Christensen J.J and Haymore B.L., Science 199(1978) 994. |
[8] | El Dossouki F.I (1998) Ph.D. Thesis; Mansoura University; Egypt. |
[9] | M Shamsipur and Ghasemi, J Inclusion Phenon Mol Recognit Chem 20 (2) (1995) 157. |
[10] | Mukhopadhyay A.; Chattopadhyay M.R. and Pal M. I nd. J. Chem. 36A(1997) 94. |
[11] | Rounaghi G; Nejad F.M. and Tahari K. , Ind.J.Chem. 38A, (1999)568. |
[12] | Sway I.M., and N Samara, J Chem Eng Data ( Amer Chem Soc.), 44 (2) (1999) 343. |
[13] | G Wenz, J.H Buschmann and E Schollmeyer, J Coord Chem, 48 (4) (1999) 465. |
[14] | K V Bel'sky, Chem Rev, 68(2) (1999) 119. |
[15] | I J Kim, Zeitschrift Fur Physikalische Chemie Neue Folge, 110 (1978) 197. |
[16] | Grzybkowski W. And Pastewski R. (1980) Electrochimica Acta 25,279. |
[17] | Gomaa,E.A ., Thermochim. Acta, 156(1989) 91. |
[18] | Gomaa, E.A., J.King Saud Univ.,vol.3 Science(1) (1991)69. |
[19] | Kappensteim CBull. Soc. Chim. Fr. 89. (1974) 101. |
[20] | Gomaa,E.A. and Al-Jahdali,B.M.,American Journal of Fluid Dynamics. 1(2011)4. |
[21] | El-Shishtawi,N.A.,Hamada,M.A. and Gomaa,E.A.,Physical Chemistry, 1(2011)14. |