F. Caridi, S. Marguccio, A. Belvedere, G. Belmusto
Agency for Environmental Protection of Calabria, Italy (ARPACal), Department of Reggio Calabria, Via Troncovito SNC, Reggio Calabria, Italy
Correspondence to: F. Caridi, Agency for Environmental Protection of Calabria, Italy (ARPACal), Department of Reggio Calabria, Via Troncovito SNC, Reggio Calabria, Italy.
Email: |  |
Copyright © 2015 Scientific & Academic Publishing. All Rights Reserved.
Abstract
River sediment samples from the Mediterranean central basin, Italy, were analyzed for 226Ra, 232Th and 40K by HPGe gamma spectrometry. Activity concentration data were reported. River sediment depositions on the bottom of rivers most frequently consist of sand and gravel particles with different grain sizes, which make them particularly valuable for building construction. Knowledge of the radioactivity present in building materials enables one to assess any possible radiological hazard to humankind by the use of such materials. Data obtained in this article provide useful information on the background radioactivity of the studied area and can be further used for radiological mapping of the Mediterranean central basin rivers.
Keywords:
Natural radionuclide, River sediments, Gamma spectroscopy, Radiological implications, Dose rate, Background radioactivity
Cite this paper: F. Caridi, S. Marguccio, A. Belvedere, G. Belmusto, Measurements of Gamma Radioactivity in River Sediment Samples of the Mediterranean Central Basin, American Journal of Condensed Matter Physics, Vol. 5 No. 3, 2015, pp. 61-68. doi: 10.5923/j.ajcmp.20150503.01.
1. Introduction
Radioactivity naturally occurs in the environment. The radionuclide content of a river depends on mineralogical features in the catchment area and the chemistry of the river as a whole. Major sources of natural radionuclides in sediments have different possible origins, like weathering and recycling of terrestrial minerals and rocks (igneous or metamorphic) containing 40K and radionuclides of uranium and thorium radioactive series, rainfall and other depositional phenomena such as gravitational settling and precipitation [1]. Human exposure to ionizing radiations is one of the scientific subjects that attract public attention, since radiation of natural origin is responsible for most of the total radiation exposure of the human population [2]. Humans should be aware of their natural environment with regard to the radiation effects due to the naturally occurring and induced radioactive elements [3]. Long-term exposure to uranium and radium through inhalation has several health effects such as chronic lung diseases, acute leucopoenia, anemia, and necrosis of the mouth. Radium causes bone, cranial, and nasal tumors. Thorium exposure can cause lung, pancreas, hepatic, bone, and kidney cancers and leukemia [4]. In this article river sediment samples from the Mediterranean central basin (in particular the Reggio Calabria district), Italy, were analyzed by using HPGe gamma spectrometry to investigate about 226Ra, 232Th and 40K activity concentration and to evaluate radiological hazard effects.Experimental results reported in this article could serve as important baseline radiometric data for future epidemiological studies and monitoring initiatives in the Mediterranean central basin.
2. Materials and Methods
2.1. Sample Collection and Preparation
Surface sediment samples, around 1 kg each, were collected twice a year (2014), following the guidelines of the Italian institute for environmental protection and research (ISPRA) [5], in nine selected locations of the Mediterranean central basin (in particular the district of Reggio Calabria, Italy): Bonamico (Bovalino), Calopinace and Gallico (Reggio Calabria city), Laverde (Africo), Budello and Petrace (Gioia Tauro), Amendolea (Bova M.), Allaro (Caulonia) and Novito (Siderno) rivers (Fig. 1).Samples were collected with a metal sampler and stored into labeled plastic boxes. At the laboratory samples were homogenized, dried at 105°C temperature, crushed, sieved and fractions lower than 2 mm were transferred to polyethylene Marinelli beakers of 1 litre capacity used for analysis. 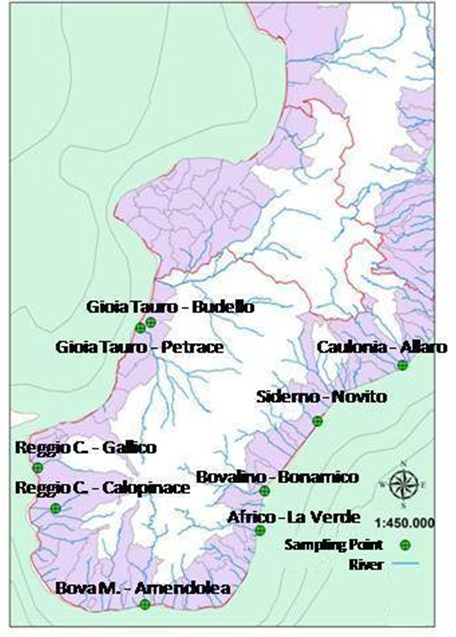 | Figure 1. Map of the Mediterranean central basin |
2.2. Gamma Spectrometry Analysis
Samples were counted for 70000 s. and analyzed to obtain the activity concentration of 226Ra, 232Th and 40K.In particular for 226Ra investigation the peak stripping method [6] was used because its γ-line at 185.99 keV interferes with that one of 235U at the close energy of 185.72 keV. The 232Th activity concentration was determined by using the 911.21 keV and 968.97 keV 228Ac γ-lines, while for 40K the evaluation was performed from its γ-line at 1460.8 keV.The experimental setup is composed by two Ortec HPGe detectors and integrated digital electronics. The first is a negative biased detector (GMX) with FWHM of 1.94 keV, peak to Compton ratio of 65:1 and relative efficiency of 37.5% at 1.33 MeV (60Co). The second one is a positive biased detector (GEM) with FWHM of 1.85 keV, peak to Compton ratio of 64:1 and relative efficiency of 40% at 1.33 MeV (60Co). Detectors were shielded from environmental background, by using lead shields with copper and tin lining. The efficiency and energy calibrations were performed using Eckert and Zigler Nuclitec GmgH traceable multinuclide radioactive standards, number SV 277, covering the energy range 59.54 keV-1836 keV, customized to reproduce the exact geometries of samples in a water-equivalent epoxy resin matrix. The Gamma Vision (Ortec) software was used for data acquisition and analysis [7]. In particular an appropriate library containing key information (energy, half-life, etc.) about the radionuclides present in the investigated samples was employed to identify them in the spectrum and then to perform correction and activity calculations. The stability of the system (activity, FWHM, centroid) was monitored at least once a week with a 60Co point source. The quality of the measurements was also demonstrated through the participation in intercomparison exercises organized by international organizations [8].A photo of the experimental setup is reported in Fig. 2. 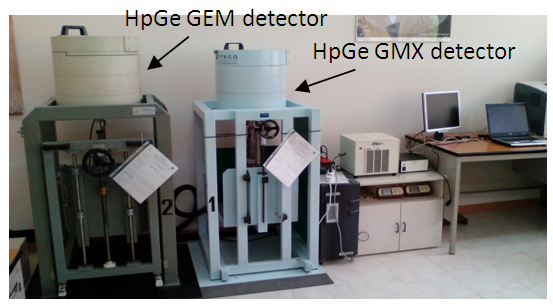 | Figure 2. A photo of the experimental setup |
3. Results and Discussion
3.1. Radioactivity Analysis
Fig. 3 reports typical gamma spectra detected with GMX (a) and GEM (b) detectors on sediment samples. 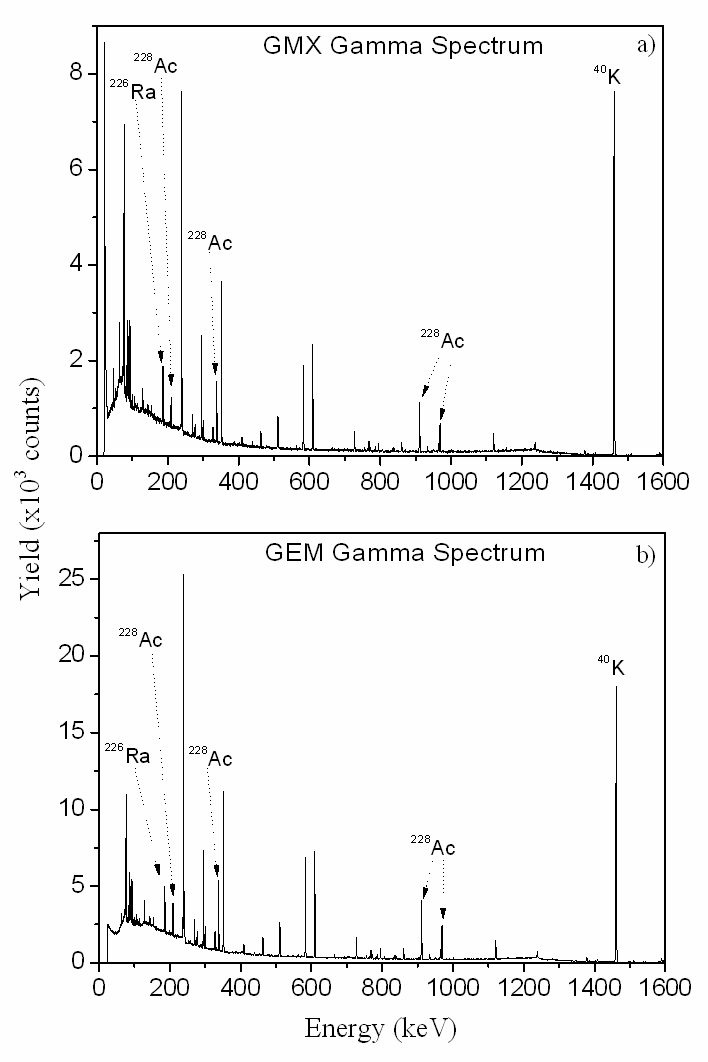 | Figure 3. Typical gamma spectra detected with GMX (a) and GEM (b) detectors on sediment samples |
The γ-lines of detected radionuclides are indicated. The activity concentration (Bq/kg) is calculated by the Gamma Vision software through the formula [7]:  | (1) |
where NE indicates net counts of the energy photopeak E, εE and γd are the efficiency and the yield of the photopeak at the energy E, respectively, M is the mass sample (kg) and t is the live time (s.). The activity concentration was corrected for the internal absorption by using the mass attenuation coefficients reported in literature [9].The measurement result uncertainty is a combined standard one at coverage factor k=2, taking into account the following components: uncertainty of the counting estimation, of the calibration source, of the efficiency calibration, of the background subtraction, of the γ-branching ratio and of the internal absorption estimation [7]. The activity concentration of detected radionuclides, 226Ra, 232Th and 40K, in the investigated sediment samples, is reported in Table 1 for both sampling for each selected point and the average value is shown in Fig. 4. This value varies from location to location, because the river bottom can exhibit large variations in chemical and mineralogical properties and rare-earth elements [10]. Table 1. Activity concentration of 226Ra, 232Th, and 40 K in the river sediment samples 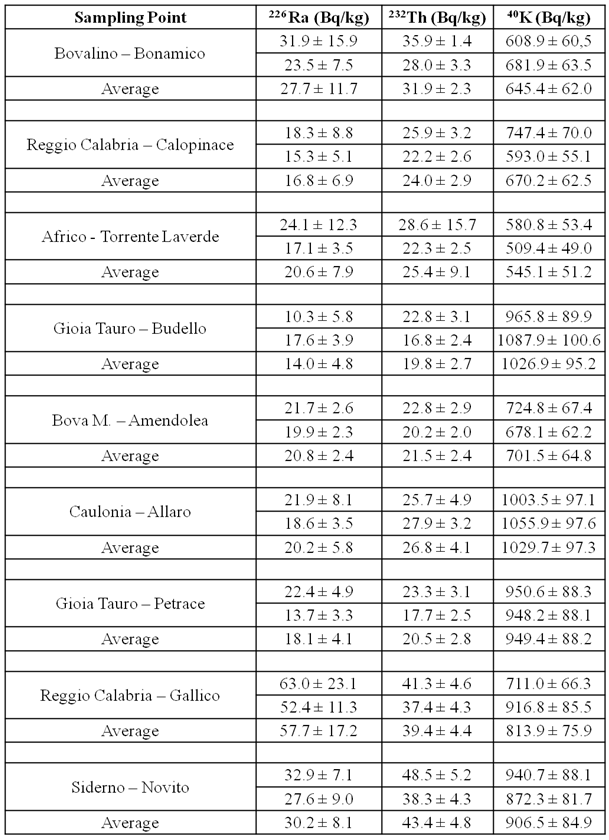 |
| |
|
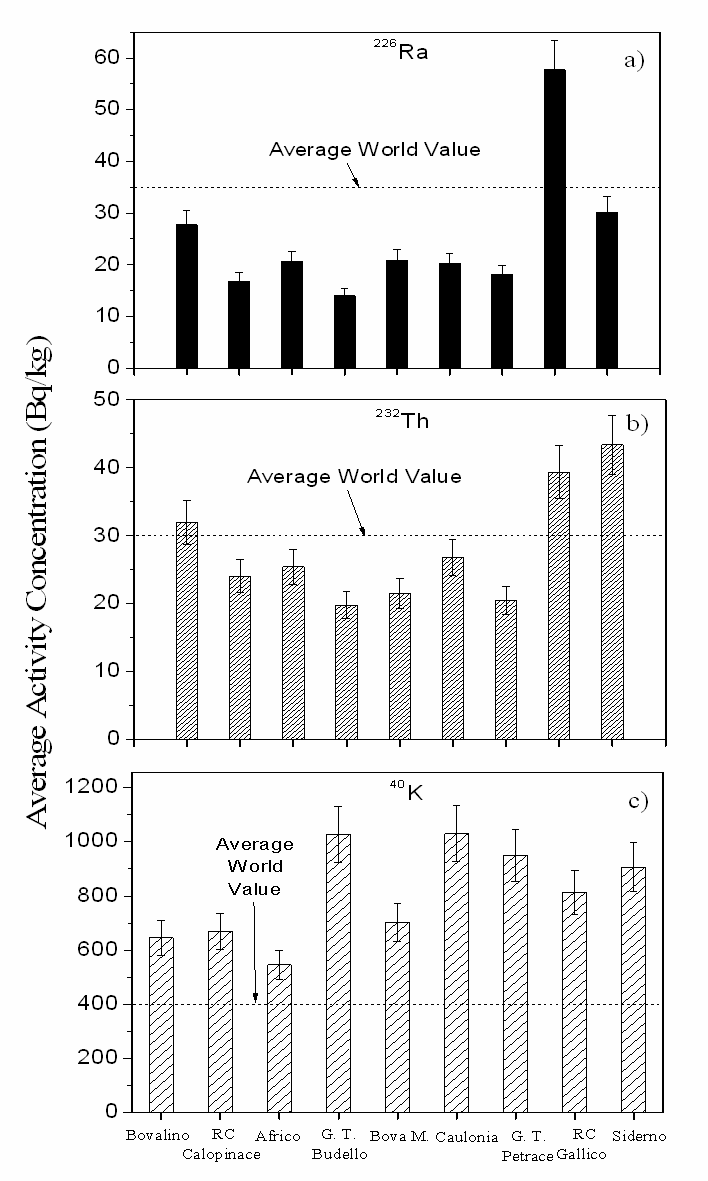 | Figure 4. The average activity concentration for 226Ra, 232Th and 40K in all investigated samples |
The 40K activity concentration is more than one order of magnitude greater than that of the 226Ra and 232Th, as normally happens in soil. The highest average concentration of 226Ra, 232Th and 40K is found in Reggio Calabria-Gallico, in Siderno-Novito and in Caulonia-Allaro rivers, respectively. Worldwide average concentrations of 226Ra, 232Th and 40K, reported by [2], are 35 Bq/kg, 30 Bq/kg and 400 Bq/kg, respectively. Our results show that the average activity concentration of 226Ra in our samples is lower than the average world value, except for the Reggio Calabria-Gallico river. The average value for 232Th is higher than the worldwide one only for Bovalino-Bonamico, Reggio Calabria-Gallico and Siderno-Novito rivers. The average activity concentration of 40K is higher than the average world value for all investigated samples.
3.2. Evaluation of Radiological Hazard Effects
3.2.1. Absorbed Dose Rate
The absorbed dose rate calculation is the first major step to evaluate the health risk. With regard to biological effects, the radiological and clinical effects are directly related to the absorbed dose rate [11]. Measured activity concentrations of detected radionuclides are converted into doses by the following equation [2]: | (2) |
where CRa, CTh, and CK are the mean activity concentrations (Bq/kg) of 226Ra, 232Th, and 40K in the river sediments, respectively. Calculated values (nGy/h) are reported in Table 2 and plotted in Fig. 5a. The average absorbed dose rate for the investigated samples is lower than the world average value (57 nGy/h) [2] only for Reggio Calabria-Calopinace, Africo-Laverde and Bova M.-Amendolea rivers.Table 2. Absorbed dose rate in air, AEDE (outdoor and indoor) and Raeq in all investigated samples 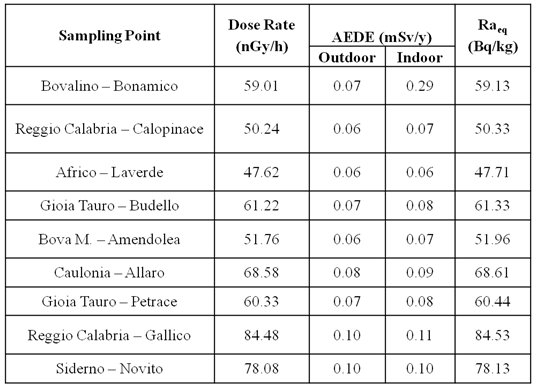 |
| |
|
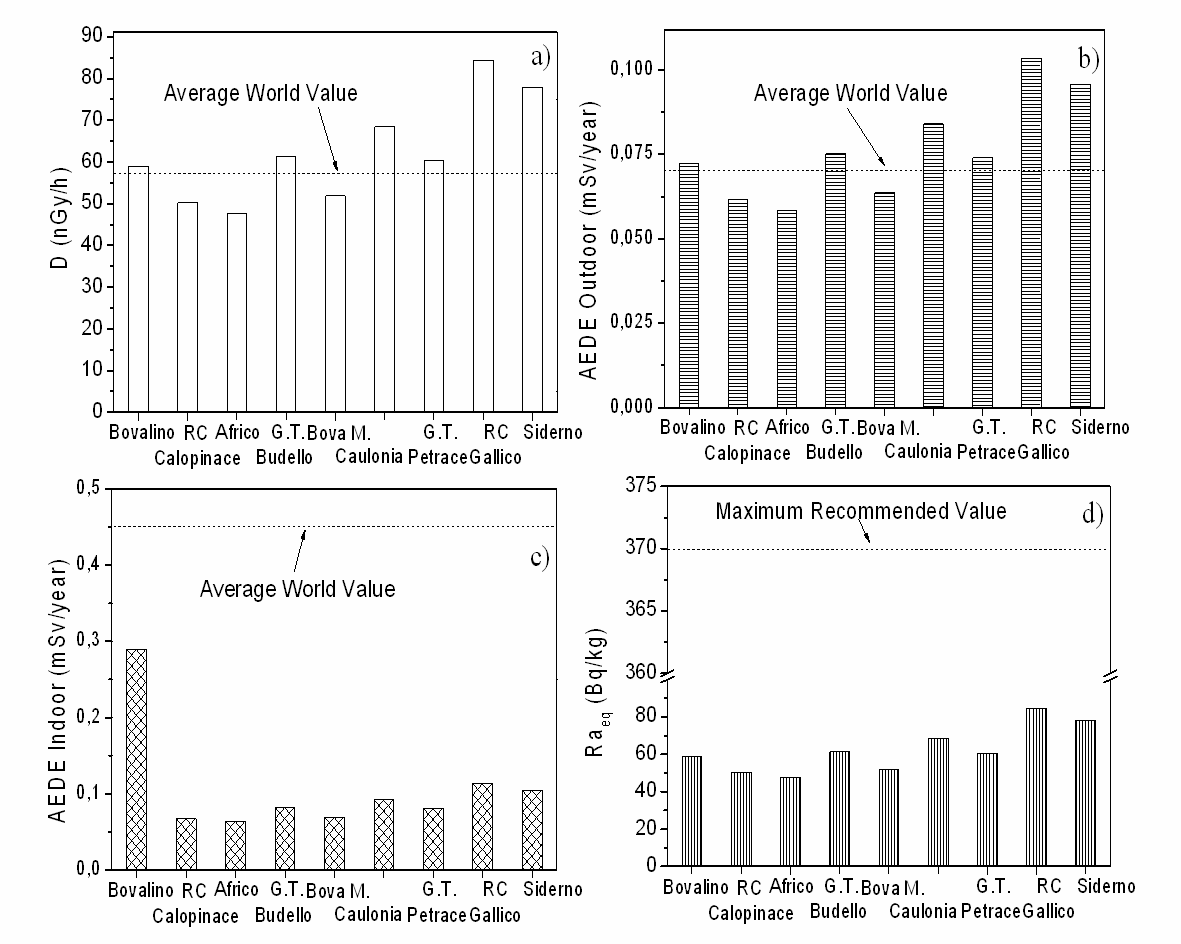 | Figure 5. Absorbed dose rate in air (a), AEDE (outdoor and indoor) (b) and (c) and Raeq (d) in all investigated samples |
3.2.2. The Annual Effective Dose Equivalent
Annual estimated average effective dose equivalent (AEDE) received by an individual was calculated using the following equations with an outdoor occupancy of 20% and 80% for indoors [12]: | (3) |
 | (4) |
Values of the AEDE for investigated locations are reported in Table 2 and plotted in Fig. 5b and 5c. The average outdoor AEDE values for sediment samples in Bovalino-Bonamico, Gioia Tauro-Budello, Caulonia-Allaro, Gioia Tauro-Petrace, Reggio Calabria-Gallico and Siderno-Novito rivers are higher than the world average value of 70 µSv/year [13]. The average indoor AEDE values are lower than the world average value of 450 µSv/year [1] for all investigated samples.
3.2.3. Radium Equivalent Activity
The Ra equivalent activity (Raeq) is a widely used hazard index calculated through the relation given by Beretka and Mathew [14]: | (5) |
where is assumed that 370 Bq/kg of 226Ra, 259 Bq/kg of 232Th and 4810 Bq/kg of 40K produce the same gamma-ray dose rate. CRa, CTh and CK are the mean activity concentrations of 226Ra, 232Th and 40K, respectively.The range of Raeq estimated for the collected samples is given in Table 2. Estimated average values are lower than the maximum recommended value of 370 Bq/kg for the safe use of materials in the construction of buildings [2]. This is due to the leaching of heavy minerals by the continuous flow of water in the river [11].
3.2.4. Hazard Indices
Beretka and Mathew [14] defined two indices (external and internal radiation hazards) to limit the radiation dose to 1 mSv/year. The external hazard index (Hex) is calculated using the given equation: | (6) |
where CRa, CTh and CK are the mean activity concentrations of 226Ra, 232Th and 40K in Bq/kg, respectively. The Hex must not exceed the limit of unity for the radiation hazard to be negligible.The internal hazard index (Hin) gives the internal exposure to carcinogenic radon and its short-lived progeny and it is given by the following formula: | (7) |
where CRa, CTh and CK are the mean activity concentrations of 226Ra, 232Th and 40K in Bq/kg, respectively. The value of Hin must also be less than unity to have negligible hazardous effects of radon and its short-lived progeny to the respiratory organs [2].Table 3 reports calculated average values of hazard indices. They are shown in Fig. 6a and 6b, respectively, and we can notice that they are less than unity for all investigated samples. Table 3. Hex, Hin, I and AGDE in all investigated samples 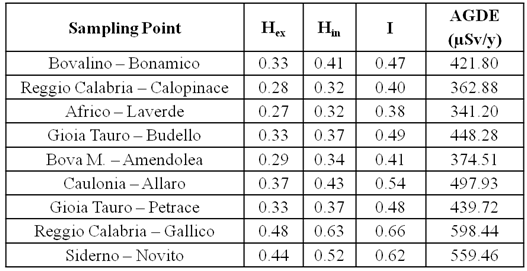 |
| |
|
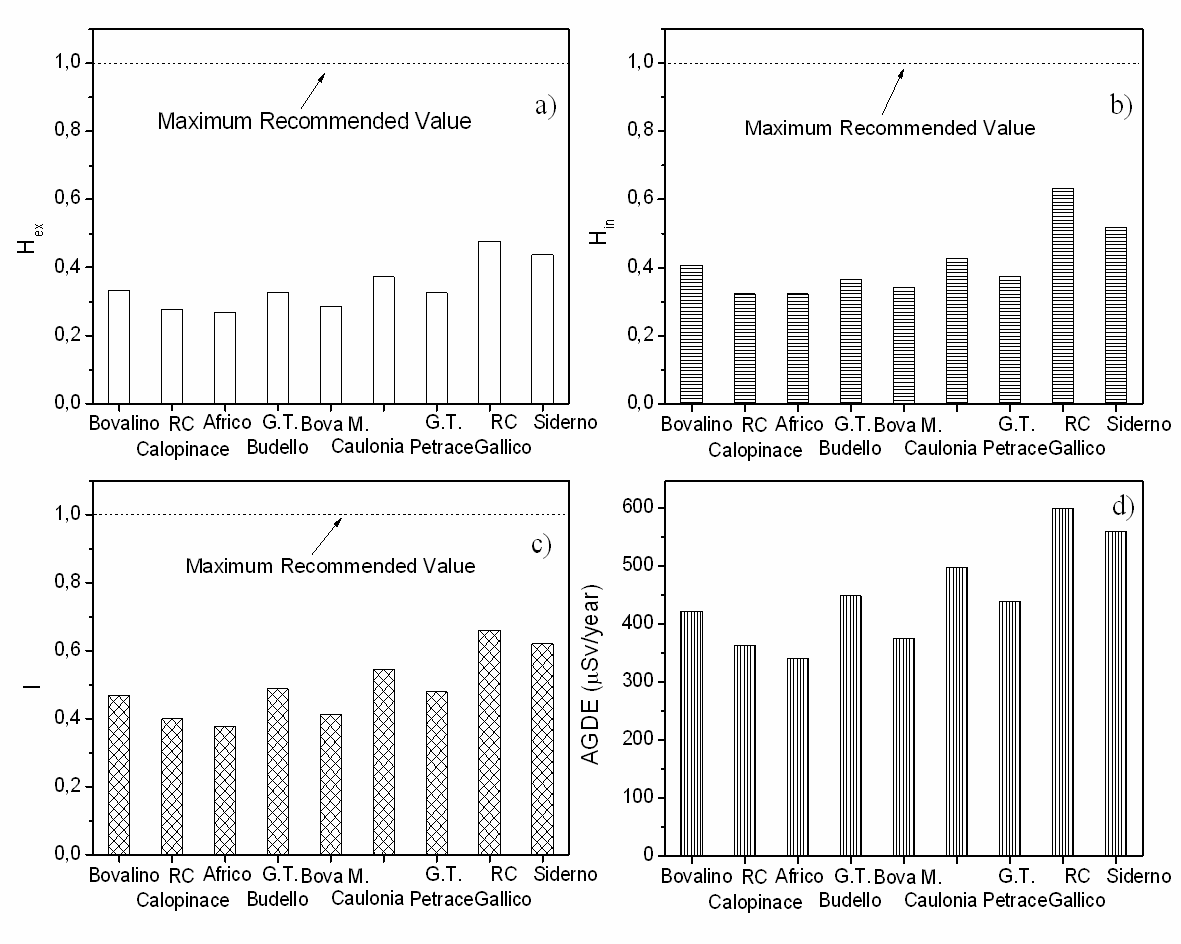 | Figure 6. Hex (a), Hin (b), I (c) and AGDE (d) in all investigated samples |
3.2.5. Activity Concentration Index
Another radiation hazard, called the activity concentration index (I), has been defined by the European Commission [15] and it is given below: | (8) |
where CRa, CTh and CK are the mean activity concentrations of 226Ra, 232Th and 40K in Bq/kg, respectively. The I is correlated with the annual dose rate due to the excess external gamma radiation caused by superficial material. Values of I ≤ 1 correspond to a criterion of 1 mSv/year [15]. Thus, I should be used only as a screening tool to identify materials that might be of concern to be used as construction materials. Those with I > 1 should be avoided since these values correspond to dose rates higher than 1 mSv/year, the highest value of the dose rate recommended for humans [16]. The distribution of the values of I for the district of Reggio Calabria sediments is presented in Table 3 and plotted in Fig. 6c. The average I in the sediment samples varies between 0.38 and 0.66 and all values are < 1. Therefore, the annual effective dose delivered by these sediment samples is smaller than the annual effective dose constraint of 1 mSv/year.
3.2.6. Annual Gonadal Dose Equivalent
The annual gonadal dose equivalent (AGDE) due to the specific activities of 226Ra, 232Th and 40K is calculated using the following formula [17]: | (9) |
The obtained AGDE values are listed in Table 3 and shown in Fig. 6d. The average AGDE values varied from 341.20 to 598.44 µSv/year, with the highest one found for the Reggio Calabria-Gallico river sample.
4. Conclusions
The activity concentration and the distribution of natural terrestrial radionuclides 226Ra, 232Th and 40K are measured using HpGe gamma spectrometry for sediment samples collected from the district of Reggio Calabria, Italy. Reported values are comparable to the average worldwide ranges and they fell within the maximum recommended values. From the experimental results the average values of the absorbed dose rate in air, AEDE (outdoor and indoor), Raeq, Hex and Hin, I and AGDE are calculated. The Raeq, Hex, Hin and AGDE are used to assess the radiological hazard of sand mixed with the sediment, since sand is used as construction material in this region. This study can be used as a baseline for future investigations and data obtained in this article may be useful for natural radioactivity mapping.
ACKNOWLEDGEMENTS
Authors thank Dr.ssa L. Settineri, Dr. Ssa E. Pellegrini, Dr.ssa M.G. Aloi, Dr. G. Altimari and Ing. L. Minutolo for the useful technical support given to this work.
References
[1] | A. El-Gamal, S. Nasr, A. El-Taher, Radiat. Meas. 42 457-465 (2007). |
[2] | United Nations Scientific Committee on Effects of Atomic Radiation, “Exposures from Natural Radiation Sources", UNSCEAR Report, New York, (2000). |
[3] | H. A. Ghrefat, N. Yusuf, Chemosph. 65 2114–2121 (2006). |
[4] | H. Taskin, M. Karavus, P. Ay, A. Topuzoglu, S. Hindiroglu, G. Karahan, Journ. of Environm. Radioact., 100 49-53 (2009). |
[5] | Italian institute for environmental protection and research (ISPRA), “Guidelines for the environmental radioactivity monitoring”, 83 (2012). |
[6] | G. R. Gilmore, Practical gamma-ray spectrometry, John Wiley and Sons Ltd. (2008). |
[7] | Ortec Gamma vision-32 v. 6 (2010). |
[8] | IAEA-TEL-2014-03, ISPRA Task 01.05.01 2013, EC Interlaboratory comparison on Cs-137 measurements in air filters 2015. |
[9] | O. Sima, Health Phys. 62 (5) 445-449 (1992). |
[10] | O. Amaro, G. Neri, M. Tarnatora, Landscape in Progress, University of Reggio Calabria, Online Edition (2014). |
[11] | V. Ramasamy, G. Suresh, V. Meenakshisundaram, V. Ponnusamy, Appl. Rad. And Isot. 69 184-195 (2011). |
[12] | United Nations Scientific Committee on Effects of Atomic Radiation, “Sources and Effects of Ionizing Radiations”, UNSCEAR Report, New York, (1993). |
[13] | Y. Orgun et al., Appl. Rad. And Isot. 65 739-747 (2007). |
[14] | J. Beretka and P.J. Mathew, Health Physics 48 87-95 (1985). |
[15] | Council Directive 2013/59/Euratom. |
[16] | R. Ravisankar et al., Appl. Rad. And Isot. 70 699-704 (2012). |
[17] | K. Mamont-Ciesla et al., Natural Rad. Environm., Halsted press, New York 551 (1982). |