Yuri Mnyukh
76 Peggy Lane, Farmington, USA
Correspondence to: Yuri Mnyukh, 76 Peggy Lane, Farmington, USA.
Email: |  |
Copyright © 2015 Scientific & Academic Publishing. All Rights Reserved.
Abstract
This article is complementary to the article Ferromagnetic State and Phase Transitions [1]; it is about paramagnetism in solids. Magnetic elements of the periodic system are currently classified into ferromagnetic and paramagnetic types. All of them, however, can exist in one or the other crystal phase depending on the thermodynamic conditions. They actually constitute a single − magnetic − type by possessing of a spin. Evidence is presented that random orientation of spins in paramagnetic crystals results from thermal rotation of the spin-carrying particles (atoms, molecules), and not spins themselves. Connection of a paramagnetic crystal state to the phenomenon of orientation-disordered crystals is revealed and its significance for the theory of paramagnetism is emphasized.
Keywords:
Magnetic type, Paramagnetic, Ferromagnetic, Phase transitions, Orientation-disordered crystals
Cite this paper: Yuri Mnyukh, Paramagnetic State and Phase Transitions, American Journal of Condensed Matter Physics, Vol. 5 No. 2, 2015, pp. 56-59. doi: 10.5923/j.ajcmp.20150502.03.
1. Introduction
The remarkably well designed tables [2] by Wolfram Research, Inc. (WRI) on the periodic system of chemical elements sort out the elements by magnetic type. There 32 elements are classified as paramagnetic type, while Fe, Co, Ni and Gd as ferromagnetic type to which Cr − the only element marked "antiferromagnetic" − should be added as a particular case of ferromagnetism. It may appear that being of one or the other type is rooted in the elements themselves. Whether it is the case will now be considered with an emphasis on the physics of a crystal paramagnetic state.
2. Paramagnetic State of Ferromagnetic Elements
The elements of the two magnetic types feature an elementary magnetic moment ("spin") − against the remaining members of the periodic system. No other magnetic difference between the elements of that combined group is directly envisioned. Still, if it is real, it could be only between the crystals they form. At this point the phenomenon is to be invoked that ferromagnetic crystals of Fe, Co, Ni, Gd and Cr turn paramagnetic at elevated temperatures (called "Curie points"). For example, it is ~770°C in Fe, ~1121°C in Co and ~ 358°C in Ni. Different interpretation of that phenomenon stems from two disparate theories of ferromagnetic state and ferromagnetic ↔ paramagnetic phase transitions.
3. The Old Theory
According to the old theory (e.g., [3, 4]), the spontaneous magnetization (regular alignment of spin directions) of ferromagnetic crystals results from spin interaction in the "electron exchange field" that supposedly existed due to overlapping of the electron shells. Ordering effect of the exchange field is opposed by thermal agitation and, at elevated temperatures ("Curie points", Table 1), the ferromagnetic spin order is destroyed. The resulting crystal phase is identified as paramagnetic by its ability to be magnetized in the direction of the applied field H, falling to zero at H = 0. This theory views the spin system as a free entity bound to neither the atoms, nor the crystal it belongs. In contradiction with that view, the spin-carrying atoms emerge in the adjacent part of the theory dealings with a paramagnetic effect − predominance of orientations of atomic magnetic moments M in the direction of applied magnetic field H. The magnetic atom is regarded there a particle of a certain structure and direction of its magnetic moment M. The applied field H does not change the angle M^H, but makes the atomic magnetic axis (and, by implication, the atom) precessing around H under the same angle. Because this does not produce a paramagnetic effect, atomic collisions in a gas medium had to be invoked. The theory has not placed magnetic atoms into the crystal lattice where they are in a close contact with the surrounding atoms.Table 1. Data excerpted from the Wolfram Research Inc. tables "Magnetic Type", "Curie Point" and "Neel Point" 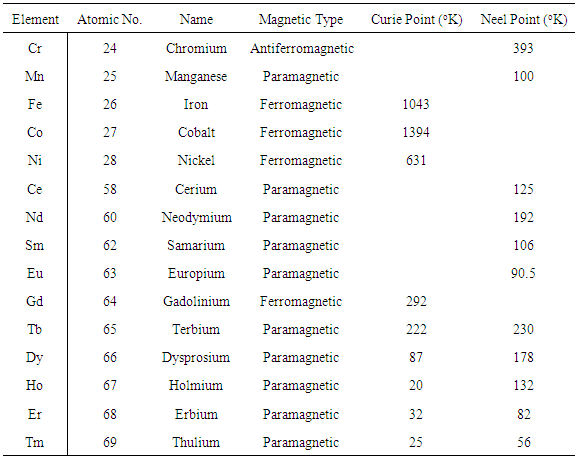 |
| |
|
4. The New Theory
The new theory [1, 5] was put forward since it became evident that the standard theory was inadequate and contradictory. Thus, the old theory was only about parallel and antiparallel spin alignments, but was unable to explain the actual unlimited diversity of spin patterns ("magnetic structures"); its predictions vastly disagreed with experiment; magnetization by interface propagation was foreign to it; the "electron exchange field" produced a wrong sign of spin interaction; the "Curie points" revealed hysteresis… and much more. The new theory maintains that ferromagnetic spin order is established by crystal forces. Indeed, it is the essence of a crystal matter to arrange both positions and orientations of its constituent particles in the long-range order according to minimum free energy of their chemical bonding. A simple fact is stressed that every spin belongs to its atomic carrier. Atoms of atomic crystal lattice function as particles of a certain geometrical shape. The chemical bonding orients atomic spin carriers and in doing so it creates a ferromagnetic spin order. Crystallographic orientation of the spin carriers (atoms, ions, molecules) in ferromagnetic crystals of any kind gives rise to the observed diversity of spin patterns (magnetic structures): parallel, antiparallel, ferrimagnetic, spiral, etc.; antiferromagnetism is not a phenomenon opposite to ferromagnetism, but a particular case of it.Still, a ferromagnetic crystal state can only be explained in the frames of the new theory if spins are invariably oriented in the structure of their atoms. But there is no physical reason to doubt it is not so. As said above, it was used by the classical theory of paramagnetic effect without arguments. More importantly, it has led to the new comprehensive account both for the stability of a ferromagnetic state and for its all manifestations [1, 5]. Finally, the actual mechanism of the ferromagnetic ↔ paramagnetic transitions indicates whether spins alone, or their carriers are rotating in paramagnetic crystals. If the former, the crystal structure remains intact. If the latter, the crystal must be reconstructed. Experimentally, the latter is found to be true. The ferromagnetic ↔ paramagnetic transitions, including those in Fe, Ni and Co [5, 6], materialize by nucleation and growth, meaning that they are structural phase transitions. (According to the now obsolete, but still used terminology, they are "first order").
5. Paramagnetic Orientation-Disordered Crystals
The novel explanation of a ferromagnetic state throws different light on the physical nature of paramagnetic crystals: it is the atomic spin carriers, and not spins themselves, are engaged in the thermal rotation. Paramagnetic crystals are simply a case of the currently well-known phenomenon called orientation-disordered crystals (ODC), not related to their magnetic properties [7-9]. The constituent particles in the ODC are engaged in a thermal rotation, hindered to one or another degree − from jumping between a number of fixed orientation positions to almost smooth rotation. The phenomenon is observed in different kind materials and is more common than meets the eye. Phase transitions CRYSTAL → ODC materialize by the universal nucleation-and-growth mechanism of crystal rearrangements [5, 10] and accompanied by increase in the specific volume (2-3% in molecular crystals, less in other cases), allowing the closely packed system of particles to go into a rotating mode. Paramagnetic state of the compounds containing magnetic atoms could be due to: (a) rotation of the whole molecules, (b) rotation of the molecular branches containing those atoms, (c) rotation of only those atoms, (d) combination of some or all those rotations. Representation of paramagnetic crystals as ODC raises question whether the classical theory of a paramagnetic effect is applicable. Considering that a close molecular packing in the ODC state is basically preserved, molecular rotation there is hindered. Within a short range it is reminiscent to interconnected rotating gears. Precession of atoms and molecules in that environment is hardly possible. On the other hand, existence of the orientational effect M → H under these conditions seems qualitatively self-evident. As for a quantitative theory, its development will be impeded by the multitude of the types and freedom degrees of the molecular rotation.
6. Ferromagnetic State of Paramagnetic Elements
After the foregoing clarification of physical nature of the paramagnetic phase produced by the crystals of "ferromagnetic-type" elements at high temperatures, the question about specifics of the 32 "paramagnetic-type" elements remains. To answer, it should be first noticed that five of them − rare earth elements (atomic No. 65-69) − exhibit Curie points as well, but located at low temperatures. However, our human perception of low and high temperatures should not be applied. Below 20 K all of them will join their counterpart Gd to become ferromagnetic. Here we deal again with the "low-temperature" ferromagnetic and "high-temperature" paramagnetic phases. Their low-temperature Curie points are high enough for particles of the paramagnetic phases to be engaged in thermal rotation. The five "paramagnetic" rare-earth elements do not constitute a separate magnetic type.There are also 5 "paramagnetic" elements (atomic No. 25, 58, 60. 62, 63) with "Neel points". Physical meaning of these "points" is identical to the Curie points, namely, to indicate the temperature separating ferromagnetic and paramagnetic phases, the difference in the names being relates only to history of science. These 5 elements do not constitute a separate magnetic type either. Both "points", however, are identically incorrect: they are not points, but temperature ranges of structural phase transitions, a subject of hysteresis [5, 10-12]. According to the WRI tables, the rest 22 "paramagnetic" elements do not reveal ferromagnetic phase at any temperature. But that does not mean the ferromagnetic area is not present in their temperature T − pressure p phase diagrams. Considering that change to the rotation mode involves an increase in the specific volume, application of sufficiently high pressure would transfer them to ferromagnetic phase.
7. Conclusions
1. The elements currently classified as either ferromagnetic or paramagnetic type are actually constitute a single magnetic type that differs from all other elements by possession of the elementary magnetic moment ("spin"). They are ferromagnetic or paramagnetic in different parts of the T − p phase diagram. The classification shows only in which crystal phase they are found at room temperature and atmospheric pressure. 2. Magnetic properties of paramagnetic crystals result from random orientation of the magnetic particles (atoms and molecules) due to their thermal rotation in the crystal lattice, and not rotation of spins alone as usually implied.3. Paramagnetic crystals are a case of the orientation-disorder crystals (ODC) not specifically related to the magnetic properties. The classical theoretical considerations about precession of magnetic atoms in a gas phase when magnetic field is applied are not applicable to the paramagnetic crystals. 4. Ferromagnetic ↔ paramagnetic phase transitions materialize by the nucleation-and-growth mechanism general to all solid-state phase transitions. They are not of a "critical" ("second order" [10]) nature. 5. It is predicted that the "paramagnetic-type elements" will produce ferromagnetic phase if sufficiently high pressure applied to their crystals.
References
[1] | Y. Mnyukh, "Ferromagnetic state and phase transitions", Am. J. Cond. Mat. Phys. 2012, 2(5): 109-115;arxive.org/abs/1106.3795. |
[2] | http://periodictable.com/Properties/A/MagneticType.html. |
[3] | C. Kittel, Introduction to Solid State Physics, 4th Ed., Wiley, (1971). |
[4] | I.E. Tamm, Fundamentals of the Theory of Electricity, Mir Publications, Moscow (1979). |
[5] | Y. Mnyukh, Fundamentals of Solid-State Phase Transitions, Ferromagnetism and Ferroelectricity, Authorhouse, 2001 [or 2nd (2010) Edition]. |
[6] | Sen Yang et al., "Evidence for first-order nature of the ferromagnetic transition in Ni, Fe, Co and CoFe2O4" , Phys. Rev. B 8, 174427 (2008). |
[7] | N.G. Parsonage and L.A.K. Staveley, Disorder in Crystals, Clarendon Press (1978). |
[8] | N. Sherwood, Plastically Crystalline State: Orientationally Disordered Crystals (1979). |
[9] | Y. Mnyukh, "Order-disorder phase transitions as crystal growth", Section 2.7 in Ref. [5]. |
[10] | Y. Mnyukh, "Mechanism and kinetics of phase transitions and other reactions in solids", Am. J. Cond. Mat. Phys. 2013, 3(4): 89-103; arxiv.org/abs/1110.1654. |
[11] | Y. Mnyukh, "Hysteresis and nucleation in condensed matter", arxiv.org/abs/1103.2194. |
[12] | Y. Mnyukh, "Second-order phase transitions, L. Landau and his successors", Am. J. Cond. Mat. Phys. 2013, 3(2): 25-30; arxiv.org/abs/1102.1085. |