A. Bentabet1, A. Azbouche2, A. Betka3, Y. Bouhadda4, N. Fenineche5
1Laboratoire de Caractérisation et Valorisation des Ressources Naturelles (LCVRN), université de Bordj Bou-Arreridj, 34000, Algeria
2Nuclear Research Center of Algiers, 2 Boulevard Frantz Fanon Alger, Algeria
3Département de physique, faculté des sciences, université de Bejaia, 6000, Algérie
4Unit of Applied Research in Renewable Energy, Ghardaïa, 47000, Algeria
5LERMPS, UTBM University, Belfort, France
Correspondence to: A. Bentabet, Laboratoire de Caractérisation et Valorisation des Ressources Naturelles (LCVRN), université de Bordj Bou-Arreridj, 34000, Algeria.
Email: |  |
Copyright © 2012 Scientific & Academic Publishing. All Rights Reserved.
Abstract
The transport of electron beam in the solids requires an accurate knowledge of their cross-sections with a target as functions of the projectile kinetic energy. Indeed, when we adjust a given analytical transport cross-section to tabulated data; the interpolation precision is considered as a validity criterion of the suggested model. In this commentary, we show that the transport cross section obtained by using the relativistic partial wave expansion method (RPWEM) could be not agreed with a simplified analytical expression of Born approximation and contains only one free parameter depending only on atomic number, which is the case of the work of Rouabah et al[Phys. Lett.A 373 (2009) 282] . Indeed, we prove that the corrections made by Rouabah et al have led to unfavorable results and their work must be reviewed and revised.
Keywords:
Key wordsFirst Order Born Approximation, Screened Cross-section, Interpolation
Cite this paper: A. Bentabet, A. Azbouche, A. Betka, Y. Bouhadda, N. Fenineche, “Improved Expression for Calculating Electron Transport Crosses Sections”: Comment on Z. Rouabah Et Al[Phys. Lett. A 373 (2009) 282], American Journal of Condensed Matter Physics, Vol. 3 No. 4, 2013, pp. 104-110. doi: 10.5923/j.ajcmp.20130304.02.
1. Introduction
Electron-transport calculations are usually performed by means of either analytical theory or Monte Carlo simulation. Both methods require an accurate knowledge of the cross section for elastic scattering of electrons as functions of the projectile kinetic energy E. Thus, the screened cross-section obtained via the first order Born approximation is extensively used in the literature. To model elastic scattering, Rouabah et al[1] reported a simplified adjustment of the electron transport cross section (TCS) for selected atomic targets ranging from H to U energies between 50 eV and 4000 eV. They proposed a correction to the approximate analytical TCS formula derived by Jablonski[2]. On other words, the principal idea of their work is based on interpolation of tabulated data via a known analytical transport cross-section. It is the reason that a large part of our work is focused on their suggested fitting. Indeed, a careful reexamination shows that their TCS formula is inadequate; therefore, the results should be examined. In our recent paper[3], we showed a short overview on some weak points of Rouabah et al paper[1]. So, the present work gives more detail on the majority of other Rouabah et al weak points especially the drastic deviation of their results.
2.
and the Screened Cross Sections (the Wentzel Model (1927)[4])
The work of Rouabah et al[1] is based on the analytical TCS
given by: 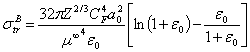 | (1) |
With CF=0.8853414,
and
.However, the authors of[1] attribute eq. (1) to Jablonski[2] saying the following words: “ The approximate analytical transport cross section as derived by Jablonsky, denoted
...”[1].Besides, Rouabah et al[1] thought that they have carried out an improvement on the Jablonski model when they said:“However, the accuracy of this formula, especially at low energies, is rather limited. An improved analytical expression was then derived by Jablonski[2] in order to provide reasonably accurate values of TCS in the energy range of interest for surface-sensitive electron spectroscopies, i.e.50–2000 eV. In the present work we suggest a simplified correction to the electron analytical transport cross section derived by Jablonski[2]. The analytical formula of Jablonski was proposed in order to provide needed values of transport cross section for energies between 50 and 4000 eV and for all elements”[1].Indeed, they have attributed the old screened cross section (which is not valid at lower energies, see table (1) of the present work) to Jablonski. In our recent paper[3] we have shown step by step that the first order Born approximation applied on the screened potential gives the same transport cross section
attributed by Rouabah et al to Jablonski [2]. Hence, the age of
is more than 60 years (since the works of Wentzel (1927)[4] and Nigam (1959)[5]). Consequently, their work must be reviewed.
3. The True Expression of the Jablonski Transport Cross Section
Actually the TCS derived by Jablonski lies in his paper[2]: “Thus, the accuracy of the first-order Born approximation for
is generally rather poor. To obtain a more accurate analytical expression for
, we need an additional analytical function
which correcting 
 | (2) |
With  | (3) |
where A0 , A1 , A2 , A3 , and A4 are fitted constants for each element.”. Therefore, the Jablonski’s TCS is that given by (3) which correspond to Eq. (21) of[2]. The accuracy of Jablonski model could be found in Jablonski paper itself[2]: “Calculations were made for atomic numbers between 1 and 96. The resulting analytical function provides values of
with a relatively high accuracy. For atomic numbers up to 30, the mean deviation between accurate values of
and values from the analytical formula reaches 0.5%. For several elements with larger atomic numbers, this deviation increases to 5%, although it is much lower for the majority of cases.”So why did Jablonski totally change the TCS expression? In other words, why didn’t Jablonski, who used five free parameters, replace one constant by a free parameter as Rouabah et al did (only one free parameter)? The answer is clear: it is impossible, with one free parameter depending only of atomic number to obtain accurate results. We shall discuss this below.
4. The Rouabah et al Parameter “k”
According to Rouabah et al[1], their TCS denoted
is given by: | (4) |
Consequently, Rouabah et al used the same TCS given by (1) by replacing the exponent 4 by an indicated free parameter “k” and the index B by “C”. They reported that[1]:”
represents the Corrected electron transport cross-section”. They assumed determine the parameter “k” by the adjustment of
to the results tabulated by Mayol and Salvat[6]. Thus they found: | (5) |
However, we asked questions about this fitting:a. Will the expression (5) well interpolate the results tabulated by Mayol et al[6]?Rouabah et al have adjusted their cross section given by (4) to that tabulated by Mayol et al[6]. So following an opposite reasoning, the authors of[1] deduced that the results of[6] agree with the eq. (4). Table (1). TCS (in A2) and the deviation of to . D’ is the percentage deviation. . : The electron transport cross section tabulated by Mayol and Salvat[6]. : TCS of Born approximation given by (1). and are tabulated here by using copy-past from tables (1) and (2) of[1] 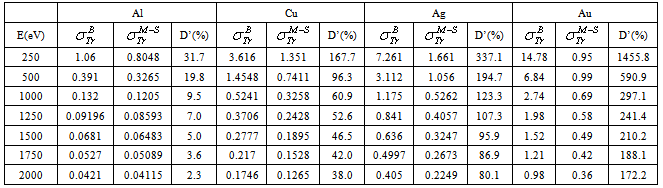 |
| |
|
Before evaluating this statement, we note that the authors of[1] have not given any explanation for their choice? We can see in fig.(2) of Jablonski’s paper[2] that the
behavior is totally different from that obtained by quantum methods, particularly in the case of heavy atoms. The right way will be to choose the interpolation function from the shape of the tabulated values and not the inverse. This is why we found in the literature analytical expressions TCS that take another form than that given by eqs. (1) or (4) (see for example[7]). Indeed Jablonski himself suggested an analytical TCS based on (1) but with another form (cf. see Equations.(2-3)).b. why did the authors of[1]choose the superscript "4" among others taken as free parameter?Number of authors has tried to modify the screened cross section based mainly on the screening parameter[3]. In other words why Rouabah et al did not choose the parameter
as a free parameter which is more reasonable than an exponent? We note also that the authors of[1] have not given any justification for their choice (i.e. the exponent “4” has been replaced by a free parameter)? Consequently, this one is another inaccuracy of their work.c. How they get through Z and E dependency of k to solely Z?Rouabah et al[1] have adjusted
to that
tabulated by[6]. So, from eq. (4) one can easily conclude that k is given by: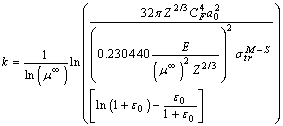 | (6) |
which is a function of Z and E. Rouabah et al[1] expressed it by eq. (5) which depends only on Z. This is yet another inaccuracy in Rouabah's paper. We note that their approach is justified only if k is set constant over a wide range of energy or varies in a non-significant manner around an average value for a fixed Z (otherwise the proposed function holds only representative for particular energies). Table (2) shows that k varies significantly with the energy for a given element. This clearly invalidates their proposal. Moreover, in Table (3) we show kexact (exact value) obtained using equation (6), the kR of Rouabah et al (fitted value) obtained using equation (5) and the deviation between them. Let’s take as an example (just to prove that our calculations are true): for Au (Z=79) at E=100 eV, | (7) |
Generally, we remark that the deviation reached drastic values for some elements especially at lower energies. This clearly invalidates their proposal. Table (2). the k parameter (equation (6)). D : the deviation between k value and the average value 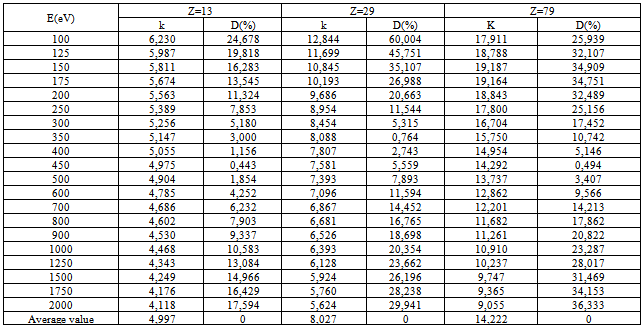 |
| |
|
Important remark: it is worth noting that the parameters k of Rouabah et al (i.e. given by equation (5)) do not correspond to the average values (see tables (2-3)). This one is too another weak point of their work.Table (3). k parameter. d: the deviation between kexact given by (6) (exact value) and kR (Rouabah et al fitted values given by equation (5) for Z=13, 29 and 79 respectively. 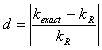 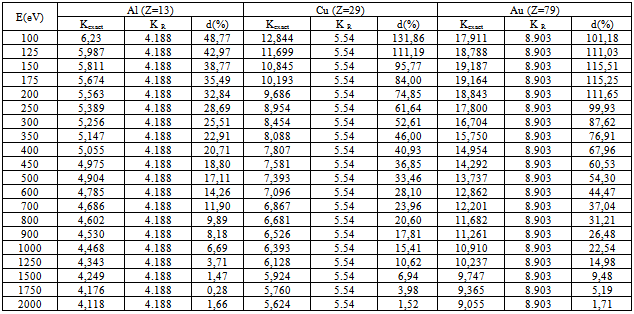 |
| |
|
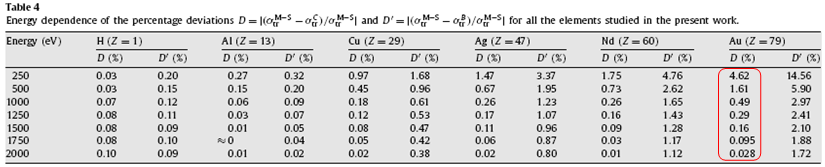 | Figure (1). the table (4) of Rouabah et al (using copy-past from[1]) |
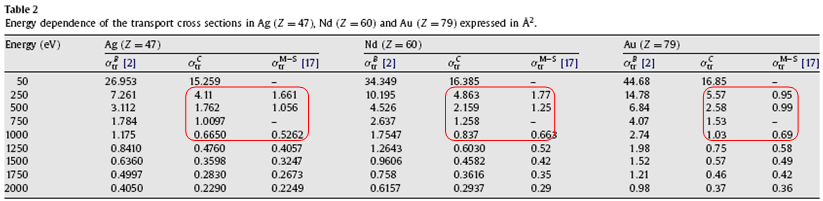 | Figure (2). the table (2) of Rouabah et al (using copy-past from their paper[1]) |
5. The Wide Deviations of Rouabah et al TCS
It is worth noting that among the most successful methods in determining the electron differential cross-sections (
) is the RPWEM (Relativistic Partial Wave Expansion Method)[8]. The differential cross-section obtained using this latter (RPWEM) presents a good agreement when compared with experimental data (see[9-10] and references therein). On other words the scattering cross section which could be measured experimentally is the differential cross-section. The total and the transport cross-section accuracy could be deduced using the next definitions: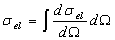 | (8) |
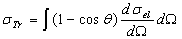 | (9) |
where
and
are the total elastic and transport cross sections, respectively. We note that Rouabah et al adjusted their cross section given by (4) to that tabulated by Mayol and Salvat[6] who used the RPWEM. Moreover, Rouabah et al carried out an erratum[11] on their results of[1] in which they precise:“(i) in all the text, the statement percentage deviation has to be replaced by relative deviation. (ii) In table (4) and fig.1, the deviations D and D’ are expressed in absolute units and not as percentages.”[11]. We think that Table (4) and Fig. 1 of[1] would be expressed as percentage commonly used in literature (i.e. more appropriate than absolute units). To prove that the TCS of[1] is inaccurate, we can also combine their results published in[1] to their erratum[11]. Indeed, we will show that their erratum[11] itself invalidates their work[1]. So, figure (1) of the present work represents the table (4) of their work[1] (represented using copy-past from[1]). Let’s take as example the Au (z=79): in the colon D (which corresponds to the deviation of their results) we find the next results: 4.62, 1.61, 0.49, 0.29, 0.16, .. According to their erratum[11] “the deviations D are expressed in absolute units and not as percentages” so, these values (4.62, 1.61,…) are in absolute units; consequently, these deviations, according to their erratum, become in percentage as follows: 462%, 161%, 49%, 29%, 16% .. These deviations are totally unacceptable for a given fitting process (the factor error of their deviation was 10000 % “ten thousand percent”, see[3]). Consequently, we note and we reconfirm that their erratum itself invalidates their work[1]. We note that we have tested their model for all the next elements: Z=3, 6, 9, 13, 16, 19, 23, 26, 29, 33, 36, 39, 43, 46 49, 53, 56, 59, 63, 66, 69, 73, 76, 79,.., 92 and we have found that the deviation reached, for number of the above elements, unacceptable values (≈100%, ≈200%, ≈300%, ≈400%, .. ≈700%). To confirm that the above deviations are the real values of the work of Rouabah et al[1], we present in figure (2) the TCS dependence of energy, which corresponds to table (2) of their work[1] (using copy-past). So, let’s take the next examples (we recall that we have done copy-past from their table (2) of[1]; i.e. without any modification):At E=250 eV:Ag (Z=47),
Nd (Z=60),
Au (Z=79), 
Table (4). program (fortran77) calculates the transport cross section given by (4-5) in the Au (Z=79) case and its deviation by comparison to that of[6] 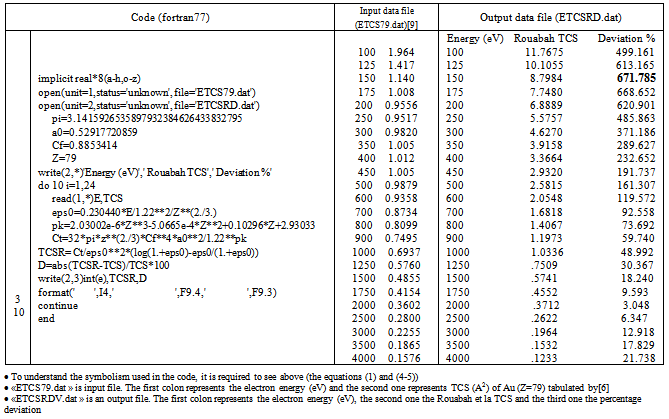 |
| |
|
At E=500 eV:Ag (Z=47),
Nd (Z=60),
Au (Z=79),
At E=1000 eV:Ag (Z=47),
Nd (Z=60),
Au (Z=79),
Let ask a question: is one of the above deviations is acceptable for the fitting process?To confirm that the deviation of their TCS model reached the value ≈700% we present in Table (4) (of the present work) a Fortran77 program calculating the percentage deviation of their TCS (
) in the case of Au (Z=79) where we remark that at E=150 eV the deviation equals to
So, since the fitted value can be considered as an approximate value to the exact one then, unfortunately, the above deviation is equivalent to say that the approximate value of 5+5 is about 77.1785 (i.e.
) because the deviation between the exact value (which is 10) and 77.1785 is
.The drastic deviation results could be observed also with other works based on quantum methods (see tables (5-6) and ref.[12]).
6. Conclusions
In summary, this work is a commentary on Rouabah et al papers[1, 11]. We presented a reminder on the screened transport cross-section
obtained using the first Born approximation. This latter (which is an old TCS) has been attributed wrongly by Rouabah et al to Jablonski. Rouabah et al who used the same mathematical expression of
by replacing only one number “4” by a free parameter depending only on atomic number without carrying out any justification. Actually Jablonski suggested his TCS as a function of
where the analytical expression is totally different from first order of Born approximation expression. Besides, Jablonski TCS expression contained five free parameters and its accuracy was an evident process. However, Rouabah et al. have used the same expression of
using only one free parameter. We have shown that their TCS is inaccurate and the weak point of their model has been hidden by deviation results leading to an error factor equal to10000 % (their deviation results should be multiplied by ten thousand percent i.e. 100). We have shown also that their erratum[11] cannot remove this weak point and actually was a proof of the invalidity of their work.Table (5). Transport cross –section (in A°2) of Al and Cu. : Rouabah et al TCS given by (4, 5)[1]. : The electron transport cross section tabulated by Mayol and Salvat[6]. : The electron transport cross section tabulated by Riley et al[13]. : The electron transport cross section tabulated by Dapor[9] 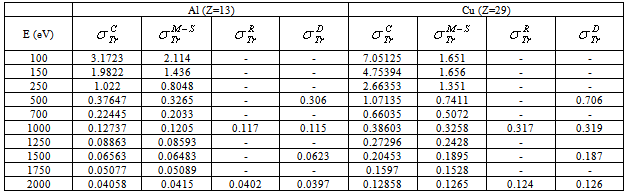 |
| |
|
Table (6). Transport cross –section (in A°2) of Ag and Au. : Rouabah et al TCS given by (4, 5)[1]. : The electron transport cross section tabulated by Mayol and Salvat[6]. : The electron transport cross section tabulated by Riley et al[13]. : The electron transport cross section tabulated by Dapor[9] 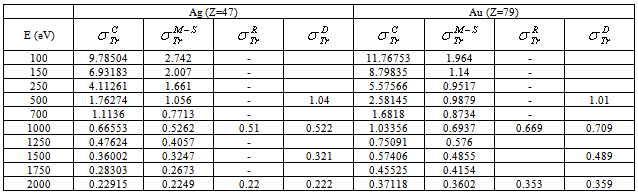 |
| |
|
References
[1] | Rouabah, Z., Bouarissa, N., Champion, C., 2009, Improved expression for calculating electron transport cross sections, Phys.Lett.A, 373, 282-284. |
[2] | Jablonski, A., 1998, Transport cross section for electrons at energies of surface-sensitive spectroscopies, Phys. Rev. B, 58 (24), 16470-16480. |
[3] | Bentabet, A., Betka, A., Azbouche, A., Fenineche, N., Bouhadda, Y., 2013, Study on Electron/Positron Scattering in Solid Targets Using Accurate Transport Cross-sections: Comment on Z. Rouabah et al Papers, American Journal of Condensed Matter Physics 3(3), 31-40. |
[4] | Wentzel, G., Z. Phys. 40 (1927) 590. |
[5] | Nigam, B. P., Sundaresan, M. K., Wu, T.-Y., 1959, Theory of Multiple Scattering: Second Born Approximation and Corrections to Molière's Work, Phys. Rev. 115, 491-502. |
[6] | Mayol, R., Salvat, F., 1997, At.Data Nucl. Data Tables, Total and transport cross sections for elastic scattering of electrons by atoms, 65, 55-154. |
[7] | Dapor, M., 1995, Analytical transport cross section of medium energy positrons elastically scattered by complex atoms (Z=1–92), J. Appl. Phys. 77, 2840-2842. |
[8] | Bentabet, A., Chaoui, Z., Aydin, A., Azbouche, A., 2010, Analytical differential cross section of electron elastically scattered by solid targets in the energy range up to 100 keV, Vacuum 85, 156-159 |
[9] | Dapor, M., 1996, Elastic scattering calculations for electrons and positrons in solid targets, J. Appl. Phys. 79 (11), 8406-8411. |
[10] | Jablonski, A., Salvat, F., Powell, C.J., 2004, Differential cross sections for elastic scattering of electrons by atoms and solids, Journal of Electron Spectroscopy and Related Phenomena vol.137–140, 299–303. |
[11] | Rouabah, Z., Bouarissa, N., Champion, C., 2009, Erratum to: “Improved expression for calculating electron transport cross sections”[Phys. Lett. A 373 (2009) 282], Phys.Lett.A 373, 1599. |
[12] | D. Liljequist, M. Ismail, F. Salvat, R. Mayol, and J. D. Martinez, 1990, Transport mean free path tabulated for the multiple elastic scattering of electrons and positrons at energies ≤20 MeV, J. Appl. Phys. 68, 3061-3065. |
[13] | Merle E. Riley, Crawford J. Maccallum, and Frank Biggs, 1975, Theoretical electron-atom elastic scattering cross sections, selected elements:1 keV to 256 kev, Atomic data and Nuclear data tables 15, 443-476.. |