Constance Maglaras1, Antonio Valdevit1, Martin A. Posner2
1Department of Chemistry Chemical Biology and Biomedical Engineering, Stevens Institute of Technology, Hoboken, U.S.A
2Department of Orthopaedic Surgery, NYU – Hospital for Joint Diseases, New York, U.S.A
Correspondence to: Constance Maglaras, Department of Chemistry Chemical Biology and Biomedical Engineering, Stevens Institute of Technology, Hoboken, U.S.A.
Email: |  |
Copyright © 2015 Scientific & Academic Publishing. All Rights Reserved.
Abstract
The objectives of this study were to determine the energy forces on the padded surfaces of professional hockey gloves from pucks striking them at high speeds and the effectiveness of the gloves to dissipate those forces. Hockey gloves used by players in the National Hockey League (NHL) were studied. The impact force of a puck striking their padded dorsal surfaces at high speed was simulated using a gravity based drop system, and the forces measured using thin film flexible force sensors. One sensor was positioned on the outside of the glove on its dorsal surface directly over the distal portion of the index metacarpal, and two were positioned inside the glove, one over that same metacarpal and the other more distal over the proximal phalanx of the index finger. Impact forces on each glove were analyzed with respect to time lag; the time difference from the initial impact force at the exterior sensor and the peak force transmitted at each internal sensor location. No differences in time lag or force transmission were observed over the index finger metacarpal or proximal phalanx regardless of variations in the padding configurations that sometimes included thin polyethylene shell inserts. However, differences were noted when the types of padding material were studied. Single and dual density foam materials were significantly more effective than multi-density materials in reducing force transmission. More effective padding materials combined with alterations in the manner they are configured would likely reduce the incidence of hand injuries in hockey players. Our studies showed that of the padding materials currently used in NHL hockey gloves, single and dual padding materials were more effective than multi-density padding in dissipating force transmissions from pucks striking at high speeds. However, for many gloves, those forces still remain too high.
Keywords:
Hockey gloves, Impact forces, Metacarpal, Phalanx
Cite this paper: Constance Maglaras, Antonio Valdevit, Martin A. Posner, Evaluation of the Protective Padding in Professional Ice Hockey Gloves, American Journal of Biomedical Engineering, Vol. 5 No. 3, 2015, pp. 94-99. doi: 10.5923/j.ajbe.20150503.03.
1. Introduction
The incidence of serious injuries in ice hockey players is one of the highest among athletes participating in team sports and exceeds those that occur in soccer, basketball, volleyball, and baseball. [1] Hockey is a team sport characterized by high velocity impacts with players being struck by pucks and sticks, as well as collisions with other players and with the boards that surround hockey rinks. Impact forces up to 5560N have been reported for a 100 mph slapshot. [2] An investigation of the fracture load of porcine metacarpals revealed fracture loads of 482.8N (range 360N-779.2N). [3] Protective equipment which can effectively dissipate forces greater than the forces required for injury is essential. In one study, collisions with other players and with the boards accounted for the majority of injuries to the wrist and hand (45%) followed by slashes from hockey sticks (19%), and puck impacts (12%). [4] Lower rates of injury occur during practice (40%) as compared to game-time competition (60%) even though ice time for players is longer during practice. The likely reason is that player-to-player impacts are less forceful during practice sessions than during games when the intensity of competition is much greater. [5] In a 16-year study between 1990-2006 an average of 20,000 ice hockey injuries were treated yearly in hospital emergency departments in the United States, with the incidence of hand fractures ranging from 5.7% to 48.1% of all injuries. [6, 7, 4] In the NHL hand fractures are also common injuries. In the 5-year period from 2006 – 2011, The NHL Health Management Panel reported 148 players who sustained game-time fractures to either a metacarpal or phalanx. These injuries resulted in a total of 1,581 lost playing days that obviously had significant impacts on the respective teams. An analysis of injuries among adult recreational ice hockey players reported injuries to the upper extremity to account for 21% of total injuries, with 29% of those injuries occurring at the hand. Fracture accounted for 29% of upper extremity injury diagnoses, however, the instance of fracture to the hand was not specifically reported. [8] A 7-year study of injuries in the men’s International Ice Hockey Federation World Championship tournaments reported 528 total injuries with 21.8% involving the upper extremities. Of those injuries, 14.2% occurred in the fingers, 10.8% at the hand and 10.8% at the wrist. [9] Despite the high risk of injury, ice hockey is a popular sport in the United States with a reported 500,000 amateur players participating in 2010-2011. [10]Epidemiological studies on ice hockey injuries and understanding mechanisms of injury for different injuries have increased with the growing concern for reducing their incidence and, if possible, their prevention. This has led to improvements in equipment and rule changes, as well as a greater awareness among athletic trainers and coaches of the importance of proper conditioning and training. [4] Studies have been performed on the protection provided by hockey helmets and have resulted in improved material and design changes. [11] Helmets as well as face protectors are currently evaluated using impact testing under American Society for Testing and Materials (ASTM) standards and are certified by the National Operating Committee on Standards for Athletic Equipment (NOCSAE). [12] There are no similar standards or certifications for hockey gloves. Our study evaluated the efficacy of professional hockey gloves having different padding designs and compositions. Our objective was to establish a testing mechanism that would evaluate the ability of the gloves to dissipate sudden high impact forces in order to determine those gloves that were most effective and therefore more likely to protect the hand from significant injury.
2. Main Body
2.1. Materials and Methods
A total of 18 pairs of new gloves from 6 different manufacturers whose gloves are currently used by players in the NHL were studied. The gloves tested had a variety of padding configurations on their dorsal surfaces, and the padding itself also varied and consisted of 3 types of foam: single, dual and multi-density. (Figure 1A) Each type sometimes included a thin, hard polyethylene shell that some manufacturers inserted in their gloves. With respect to the padding configurations, three groups were classified based on the their configurations on the dorsal surface of the fingers: gloves having three rolls over index finger and two rolls over the other fingers (designated as longitudinal geometry), gloves having three rolls over all four fingers (designated as small-faceted geometry) and gloves having two rolls over all four fingers (designated as large-faceted geometry). (Figure 1B)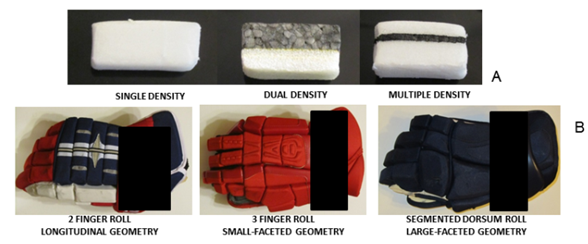 | Figure 1. A) Three types of padding used in professional hockey gloves. B) Dorsal padding configuration; three classifications |
To evaluate the protection provided by hockey gloves against pucks striking their dorsal surfaces at high speeds, a gravity drop-tube system was used. A regulation NHL puck securely attached to a specific weight was dropped from a pre-determined height in the tube to strike the dorsal surface of each glove at a specific site. (Figure 2) Our goal was to simulate game conditions when pucks often strike player’s gloves at high speeds in the range of 100mph. For the drop-tube, a PVC pipe (Pipelife Jet Stream Inc., Siloam Springs, AR), slightly larger than the diameter of the drop weight was used and was firmly attached to an aluminum frame. 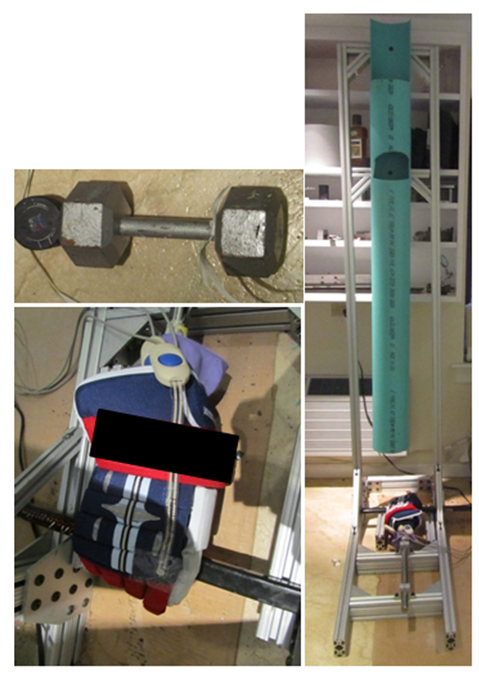 | Figure 2. A regulation NHL hockey puck secured to a 20 lb. weight was dropped from a pre-specified height to impact hockey gloves with the fingers positioned around a NHL hockey stick |
To simulate a player’s hand in the glove, a commercial rubber glove filled with a hardening mixture (Bondo, 3M Inc., St. Paul, MN) was inserted. A hollow cardboard cylinder, sealed at the distal end, was then inserted the palm portion of the rubber glove to facilitate aligning the dorsal side of the hockey glove with the test frame. Three thin film flexible sensors, each with a force capacity of 4450N (1000lbs), (FlexiForce B201, Tekscan, South Boston, MA) were used to measure impact forces. Sensor #1 was located on the outside of the glove over the distal end of the index metacarpal and the other two sensors on the inside of the glove, one over the distal part of the index metacarpal (sensor #2) directly beneath sensor #1, and the other over base of the proximal phalanx of the index finger (sensor #3). (Figure 3) Sensor locations were determined by considering the areas of the hand which are most exposed whilst gripping the hockey stick, as well as allowing reproducible locations between gloves. The 3 sensors were secured into their proper positions using double-sided adhesive tape. The sensors were preconditioned by cyclic loading from -100N to -1000N at 0.5Hz for 50 cycles using a materials ELF 3300 testing machine (Bose Corporation, Electroforce Systems Group, Eden Prairie, Minnesota) and calibrated according to the manufacturer’s instructions. A Multi-Handle Economical Force and Measurement (MELF) System (Tekscan, South Boston, MA) was used to record the output from all 3 sensors simultaneously. 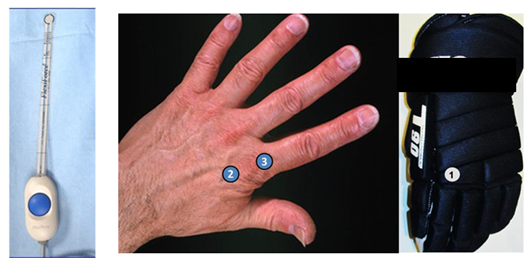 | Figure 3. Three thin film sensors were used; one on the outside of the glove over the distal end of the second metacarpal (1) and two on the inside the glove, one (2) at the same location as outside sensor and the other (3) over the proximal phalanx |
The hockey glove containing the hand model with the three sensors attached to their proper positions was secured to the mounting rod and properly aligned so that the impact would be directly over sensor #1. The sensors were then connected to the MELF data acquisition and their correct position was checked by applying digital pressure to the outside of the glove. To simulate game conditions, a NHL hockey stick was placed beneath the palm of the glove and the fingers of the glove wrapped around the stick. The puck and weight were secured to a rope that was then lowered to a predetermined height in the tube before it was released.If only the mass or weight of the hockey puck was considered, it would have to be dropped from a considerable height that would be impossible to achieve in most research facilities. However, by securing it to a much heavier object, the height requirement could be reduced. In order to determine a reasonable height, potential energy (PE) was calculated as;  | (1) |
with m representing mass, g gravity, and h the height from the impact surface. We determined that a 20lb weight dropped from a height of 1.35m, would produce a PE equal to 121 Joules of impact energy. At impact, potential energy (PE) equals kinetic energy (KE). Therefore;  | (2) |
with md representing the total mass of the drop weight, and v the velocity prior to impact. Since the change in kinetic energy of an object is equal to the net work on the object,  | (3) |
with W representing work, F the impact force and d the distance traveled during impact, or the deformation of the glove padding material at impact. We assumed a worst-case scenario that the entire padded surface of the glove material would be deformed. For most gloves the measured padding thickness was approximately 20mm. Therefore, F = 6095N, similar to the 5560N force reported by Sim and Chao who analyzed the injury potential in ice hockey in relation to impact forces using high-speed cinematography and achieved puck velocities up to 100 mph. [2]Gloves were grouped and analyzed according to three categories: the geometry of the padding on their dorsal surfaces, the location of thin polyethylene shell inserts in the padding when they were present, and the type of padding material. Data was recorded at 5000Hz with a trigger force of 5N used to initiate force versus time data recording from the sensors. The data was used to determine the peak force versus time obtained at each sensor site, the time lag to peak impact at the interior sensor sites and the maximum force transmission. Three successful impact tests were performed on each glove. Graphs of force versus time were generated and reduced using Prism 5.0 (GraphPad, Inc, San Diego, CA). Peaks less than 10% of the maximum force were ignored. For the peak immediately following external impact, the maximum force and associated time were extracted. The time lag was determined by subtracting the peak time at sensor #1 by the time for the first peaks at sensor #2 and sensor #3. The maximum force transmissions at sensors #2 and #3 were expressed as a percentage of the impact force and then averaged for each glove. Statistical comparisons for time lag and force transmission were performed using a One-way Analysis of Variance (ANOVA) with a Newman-Keuls post-hoc analysis (Prism 5.0, GraphPad, Inc., San Diego, CA) to determine differences between material groups (P<0.05).
2.2. Results
A typical impact response was characterized by an initial rise in force on the exterior of each glove (sensor #1) followed by subsequent force increases on the inside of each glove at sensors #2 and #3, located over the index metacarpal and proximal phalanx of that finger respectively. (Figure 4)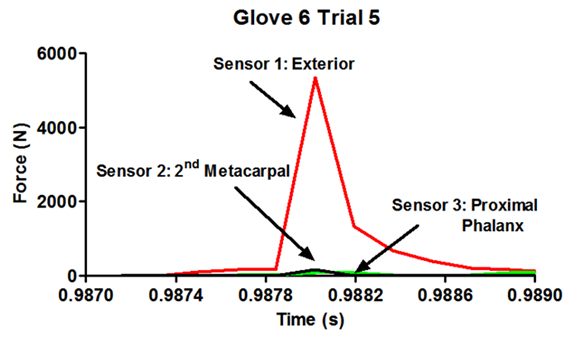 | Figure 4. Typical impact response showing the forces at all 3 sensors |
For the proximal phalanx, the percentage transmissions ranged from 4.7% to 109.5%, representing loads ranging from 160.5N to 558.4N (36.1lbs-125.5lbs). (Figure 5A) The mean percent of all 18 gloves was 46.0% and the mean load was 455.6N (102.4lbs). For the second metacarpal, the percentage transmissions ranged from 3.5% to 130.6%, representing loads ranging from 107.3N to 128.9N (24.1lbs-29.0lbs). (Figure 5B) The mean percent for all 18 gloves was 31.7% and the mean load, 363.2N (81.7lbs). Since each glove tested was wrapped around a hockey stick, the fingers of the glove cantilevered back on impact, producing a secondary recoil force.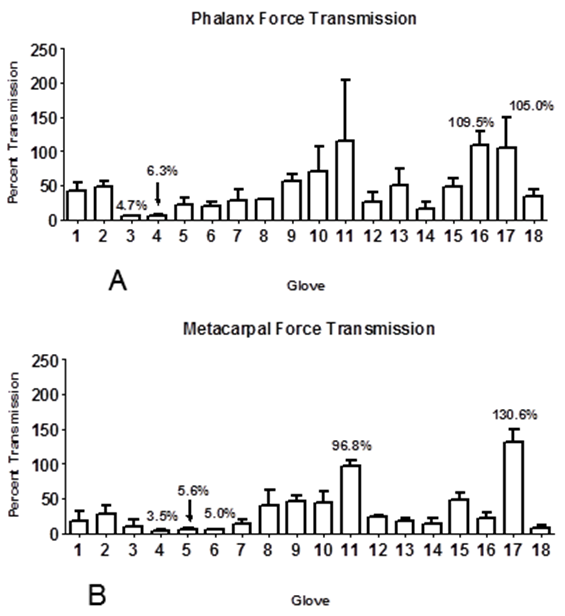 | Figure 5. Force transmissions for the 18 gloves tested expressed in percentages over A) the proximal phalanges and B) the second metacarpals |
When categorized by the geometry of their dorsal pads, force transmissions ranged from 4.8% to 203.6% at sensor #2 and 1.4% to 105% at sensor #3; when categorized by the padding material, they ranged from 8.2% to 57.2% at sensor #2 and 3.3% to 39.9% at sensor #3; and when categorized by the presence of polyethylene shells, they ranged from 4.0% to 203.6% at sensor #2 and 1.4% to 148.6% at sensor #3. Regardless of padding configuration, there were no statistically significant differences in time lag between the impact force and the percent transmission of that force to either the second metacarpal or proximal phalanx (ANOVA P>0.4). There were also no differences among the three configurations regarding the percentages of transmitted force (ANOVA P>0.3). (Figure 6A)Regardless of the location of polyethylene inserts on the surface or within the padding, there were no significant differences in time lag to either the proximal phalanx or the second metacarpal (ANOVA P>0.06), or force transmissions (ANOVA P>0.5). The inserts were statistically insignificant; they did not improve the effectiveness of the padding to dissipate forces. (Figure 6B)Single and multiple density foams showed a reduced time lag as compared to the dual density foam. However, the differences were not statistically significant at either the proximal phalanx or the second metacarpal (ANOVA P>0.06). Force transmission values for single and dual density foam materials were significantly reduced as compared to multi-density foam materials (ANOVA P<0.01). (Figure 6C) For the second metacarpal, the percentage force transmission for single and dual density materials were 30% as compared to 74% for the multi-density material, and for the proximal phalanx the values were 19% and 57% respectively. 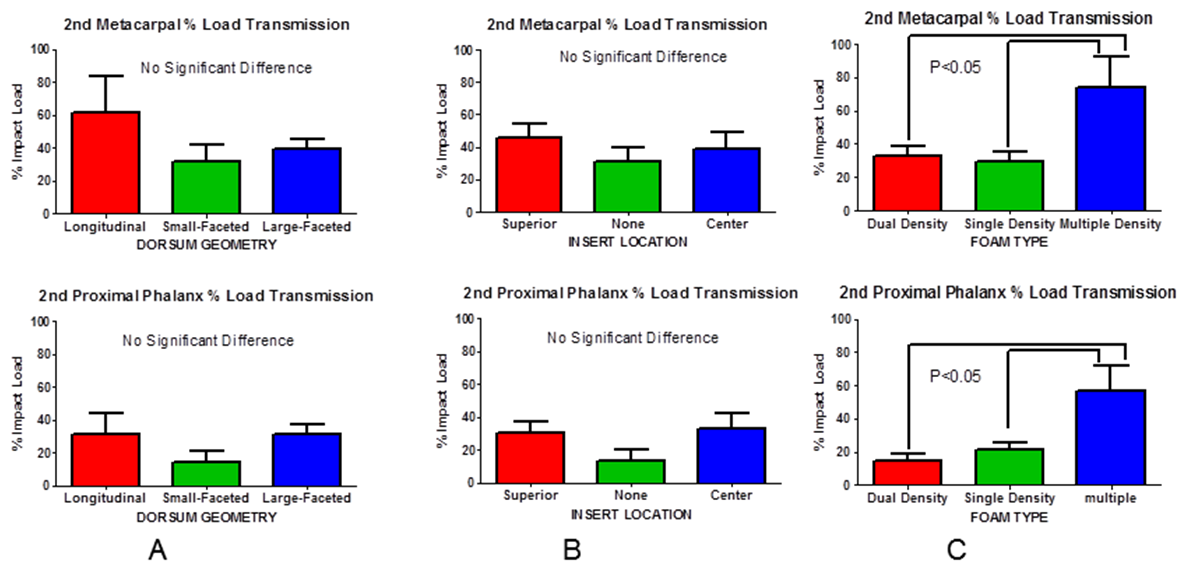 | Figure 6. Results of force transmissions according to A) dorsal geometry, B) polyethylene inserts (when present), and C) type of foam padding |
One tested glove modified by a NHL team, which added a thin honeycomb pad (Supracor, San Jose, CA) on its dorsal surface, significantly reduced transmitted forces compared to the original glove design. In the original glove, force transmissions to the metacarpal and proximal phalanx were 55.1% and 43.4% respectively compared to 4.7% and 10.4% respectively after the gloves were modified. The reduction in force transmission was statistically significant at the metacarpal (P<0.02) but not at the proximal phalanx (P<0.05).
2.3. Discussion
Protective designs for hockey gloves have traditionally been based on player experience, and trial-and-error engineering and manufacturing. We evaluated 18 different gloves, many with different designs that are currently used by players in the NHL using an impact testing apparatus and thin film force sensors that were located on the outside and inside of the gloves; a test configuration that has not been previously reported. Our studies determined the time lag from initial peak impact on the outside of each glove to peak impacts at two locations on the inside of each glove. The force transmission from outside to inside was determined as a percentage of the maximum impact force and was calculated to be approximately 6100N. While this computation is valid, it does not account for the lack of geometric conformation between the puck and force sensor. A decrease in the contact area would result in a reduced voltage output from the sensor and therefore, reduced impact force values. Within each glove, compression of the padding material will ensure a more uniform contact between the padding and the contact sites of the sensors. In order to account for slight variations in contact under impact with respect to the exterior sensor, the transmitted forces were expressed as a percentage of the impact force. We achieved a force of 4939N that was comparable to the 5560N that was recorded in a study using high-speed cinematography of a puck travelling at 100mph. [2]Ochman et.al. investigated a metacarpal fracture model under bending at a rate of 100mm/min. using porcine metacarpals. [3] Intact second metacarpal specimens displayed a mean yield load of 303N (range 200N-500N) and a mean fracture load of 482.8N (range 360N-779.2N). In comparison to the absolute values obtained in this study, most of the gloves examined would provide protection as in this study the maximum load transmitted was 558.4N (in middle of the range for fracture as reported by Ochman), while the low-end transmission was less than 110N. When evaluating protective characteristics of hockey gloves, it would be unrealistic to assume that injuries can be completely eliminated. Regardless of the configuration of the padding or the composition of that padding, injuries will still occur. However, their incidence can be reduced if impact forces on gloves are dissipated below levels that result in significant soft tissue and bony injuries. The key is the dissipative qualities of the padding material. An improvement in that padding would be manifested through an increase in the time between initial impact and force transmission to the hand, namely the lag time. In our study, we expressed that parameter as a percentage of the impact force. With respect to the polyethylene inserts, no statistical differences were observed in time lag or force transmission to either proximal phalanx or second metacarpal. These results implicate the ineffectiveness of polyethylene insert inclusion to improve force dissipation. A study which examined the impact absorption and dissipation of ethylene vinyl acetate mouth guards with and without a thin titanium layer produced comparable results. No statistical differences were observed between the mouth guard with and without the titanium layer, suggesting no beneficial effect of the added layer on the impact absorption and dissipation. [13]Differences in glove geometry had no statistical differences in time lag or force transmission for both the proximal phalanx and second metacarpal, however, a trend of reduced force transmission is observed for small-faceted geometry. Though not statistically significant, this observation may be attributed to a better resistance of force transmission in smaller sized foam segments than larger. The smaller segments may be restricting the parameters associated Poisson’s ratio, thereby limiting expansion of the material upon impact. The effect of material properties on energy dissipation in helmets has been widely documented, showing that an increase in foam density and stiffness yields an increase in energy dissipation capabilities. [14] However, analyses of protective equipment employing multiple density foams compared to use of a single density foam is lacking. In this study, single and dual density materials were comparable with respect to the percent of the transmitted force. However, multi-density materials significantly increased the percent of transmitted force over both the second metacarpal and proximal phalanx of that index finger as compared to single and dual density foams on an order of 2.5 to 3 times. That increase is probably because multi-density materials permit only a gradual change in modulus over the thickness of the material when subjected to an impact force. As a consequence of this gradual change in modulus, there is little diminution of the impact force and it is simply transmitted to the inside of the glove. In contrast, a significant change in modulus at the interface between materials that comprise the padding will more likely dissipate and scatter an impact force, and decrease its transmission. This also accounted for the lack of statistical differences with respect to the lag time data. The transmission of the impact wave may not necessarily decrease in velocity but may simply decrease in amplitude. The initiation for data acquisition in our study was a force of 5N or 0.5% of the sensor capacity and the lag time data was computed based upon the differences in the peak forces. It may be appropriate to consider time differences with respect to the initiation of the force profiles although this method may be more subjective in establishing exact time points for force initiation.Based upon the findings of our study, manufacturers of protective equipment should avoid using multi-density foam materials for padding that were not nearly as effective as single and dual density foams in reducing force transmissions. The challenge for future glove designs is to achieve better energy dissipation using materials that are durable, lightweight and comfortable, and perhaps also altering the manner in which they are configured.
3. Conclusions
This study showed that of the padding materials currently used in NHL hockey gloves, single and dual padding materials were more effective than multi-density padding in dissipating force transmissions from pucks striking at high speeds. However, for many gloves, those forces still remain too high. More effective padding materials combined with alterations in the manner they are configured would likely reduce the incidence of hand injuries in hockey players.
ACKNOWLEDGEMENTS
The authors appreciate the assistance provided by the National Hockey League Health Management Panel for providing hand injury statistics in the league and for providing the gloves we tested. The authors also thank Glen Sather, President and General Manger of the New York Rangers; Jim Ramsay, the Head Athletic Trainer; and Acasio Marques, the Head Equipment Manager, for their encouragement in carrying out this study and their insight into hockey injuries based on their many years of experience in the sport.
References
[1] | Kujala UM, Taimela S, Antti-Poika I, Orava S, Tuominen R, Myllynen P. Acute injuries in soccer, ice hockey, volleyball, basketball, judo, and karate: analysis of national registry data. BMJ. 1995;311:1465-1468. |
[2] | Sim FH, Chao EY. Injury potential in modern ice hockey. Am J Sports Med. 1978;6(6):378-384. |
[3] | Ochman S, Vordemvenne T, Paletta J, Raschke MJ, Meffert RH and Doht S. Experimental Fracture Model versus Osteotomy Model in Metacarpal Bone Plate Fixation. The Scientific World Journal,2011;11:1692–1698. |
[4] | Mölsä J, Kujala U, Näsman O, Lehtipuu TP, Airaksinen. Injury Profile in Ice Hockey from the 1970s through the 1990s in Finland. Am J Sports Med. 2000;28(3):322-327. |
[5] | Stuart MJ, Smith AM, Nieva JJ, Rock MG. Injuries in youth ice hockey: a pilot surveillance strategy. Mayo Clin Proc. 1995;70:350-356. |
[6] | Castaldi CR, Bishop PJ, Hoemer EF, Eds.: Safety in Ice Hockey: Second Volume, ASTM STP 1212. Philadelphia, PA, American Society for Testing and Materials. 1993; 1-16. |
[7] | Deits J, Yard EE, Collins CL, Fields SK, Comstock RD. Patients With Ice Hockey Injuries Presenting to US Emergency Departments, 1990-2006. J Athl Train. 2010;45(5):467-474. |
[8] | Caputo, P., Mattson, D., J. Recreational Ice Hockey Injuries in Adult Non-checking Leagues: A United States Perspective. Journal of Sports Science and Medicine. 2005; 4:58-65. |
[9] | Tuominen, M., Stuart, M., J., Aubry, M., Kannus, P., Parkkan, J. Injuries in men’s international ice hocley: a 7-year study of the International Ice Hockey Federation Adult World Championship Tournaments and Olympic Winter Games. |
[10] | USA Hockey. 2010/2011 USA Hockey Annual Report. Available at: http://www.usahockey.com. |
[11] | Bishop PJ, Arnold J. The Effectiveness of Ice Hockey Helmets in Limiting Localized Loading on the Head. Safety in Ice Hockey: Second Volume, ASTM STP 1212. Philadelphia, PA. American Society for Testing and Materials. 1993; 175-182. |
[12] | Norman, R.W. Biomechanical evaluation of sports protective equipment. Exercise and Sport Science Reviews. 1983; 11: 232-274. |
[13] | Kataoka, S.,H., Setzer .,FC.,Gondim Jr., E., Caldeira CL. Impact absorption and force dissipation of protective mouth guards with or without titanium reinforcement. J. Am. Dent. Assoc. 2014;145(9):956-9. |
[14] | Salimi Jazi, M., Rezaei, A., Karami, G., Azarmi, F., Ziejewski, M. A Computational Study of Influence of Helmet Padding Materials on the Human Brain under Ballistic Imapcts. Computer Methods in Biomechanics and Biomedical Engineering, 2014;17(12):1368-1382. |