Stephen Olaribigbe Majekodunmi
Department of Pharmaceutics and Pharmaceutical technology, Faculty of Pharmacy, University of Uyo, Uyo, Nigeria
Correspondence to: Stephen Olaribigbe Majekodunmi, Department of Pharmaceutics and Pharmaceutical technology, Faculty of Pharmacy, University of Uyo, Uyo, Nigeria.
Email: |  |
Copyright © 2015 Scientific & Academic Publishing. All Rights Reserved.
Abstract
The importance of centrifugation in the pharmaceutical industry has rarely been studied. Centrifugation is one of the most important and widely applied research techniques in biochemistry, cellular and molecular biology and in evaluation of suspensions and emulsions in pharmacy and medicine. This review focuses on the basics and principle of centrifugation, classes of centrifuges, types of centrifuge separations, different types of density gradient media, classification of industrial centrifuges, types of industrial centrifuges and applications of centrifugation in the pharmaceutical industry. Centrifugation, clearly, plays an important role in the pharmaceutical industry in the production of bulk drugs, biological products, determination of molecular weight of colloids and in the evaluation of suspensions and emulsions.
Keywords:
Centrifugation, Centrifuge, Gravitational force, Separation, Centrifugal force
Cite this paper: Stephen Olaribigbe Majekodunmi, A Review on Centrifugation in the Pharmaceutical Industry, American Journal of Biomedical Engineering, Vol. 5 No. 2, 2015, pp. 67-78. doi: 10.5923/j.ajbe.20150502.03.
1. Introduction
Centrifugation is one of the most important and widely applied research techniques in biochemistry, cellular and molecular biology, evaluation of suspensions and emulsions in pharmacy and in medicine. Current research and clinical applications rely on isolation of cells, subcellular organelles, and macromolecules, often in high yields. A centrifuge uses centrifugal force (g-force) to isolate suspended particles from their surrounding medium on either a batch or a continuous – flow basis [1].Many particles or cells in a liquid suspension, given time, will eventually settle at the bottom of a container due to gravity. However the length of time required for such separations is impractical. Other particles, extremely small in size, will not separate at all in solution, unless subjected to high centrifugal force. When a suspension is rotated at a certain speed or revolutions per minute (RPM), centrifugal force causes the particles to move radially away from the axis of rotation. The force on the particles (compared to gravity) is called Relative Centrifugal Force (RCF). For example, an RCF of 500 x g indicates that the centrifugal force is 500 times greater than earthly gravitational force.Centrifugation is a process which involves the use of the centrifugal force for the sedimentation of heterogeneous mixtures with a centrifuge, used in industry and in laboratory settings. This process is used to separate two immiscible liquids. More-dense components of the mixture migrate away from the axis of the centrifuge, while less-dense components of the mixture migrate towards the axis. Pharmacists, chemists and biologists may increase the effective gravitational force on a test tube so as to move rapidly and completely cause the precipitate ("pellet") to gather on the bottom of the tube. The remaining solution is properly called the "supernate" or "supernatant liquid". The supernatant liquid is then either quickly decanted from the tube without disturbing the precipitate, or withdrawn with a Pasteur pipette [2].As enunciated by Sir Isaac Newton in his first law of motion, a freely moving body (such as a ball) tends to travel in a straight line, and if directed along a curved path by some restraining force (such as would result were a hand-held string tied to it) it will exert a force against the directing or restraining force in its continual effort to fly off onto a straight tangential course. It is a familiar observation that an object revolving in a circle exerts a force away from the centre of rotation. This force, which is the outward pull of the ball on its string, is the centrifugal force. Also, there is general appreciation of the fact that the amount of this force can be increased by increasing either the angular velocity of rotation, the mass of the object, or the radius of the circle through which the object moves. Perhaps not so generally appreciated is the fact that whereas the centrifugal force is directly proportional to the radius and to the mass, it is proportional to the square of the angular velocity. For example, doubling the mass of the rotating object will increase the centrifugal force by a factor of 2, but doubling the number of revolutions per minute (rpm) will increase the centrifugal force by a factor of 4 (equals 2 times 2); similarly, increasing the speed by a factor of 10 will increase the force by a factor of 100 (equals 10 times 10). Centrifugal force is expressed by the basic relation F = mν2 / R = 4π2mn2R; F is the centrifugal force, m the mass, R the radius, v the speed, and n the number of revolutions per second [3].Centrifuge is any device that applies a sustained centrifugal force; that is, a force due to rotation. Effectively, the centrifuge substitutes a similar, stronger, force for that of gravity. Every centrifuge contains a spinning vessel; there are many configurations, depending on use. A perforated rotating drum in a laundry that throws off excess water from clothes, for example, is a type of centrifuge. A similar type is used in industry to separate fluids from solid matter after crushing.
2. Basics of Centrifugation
The earth's gravitational force is sufficient to separate many types of particles over time. A tube of anticoagulated whole blood left standing on a bench top will eventually separate into plasma, red blood cell and white blood cell fractions. However, the length of time required precludes this manner of separation for most applications. In practice, centrifugal force is necessary to separate most particles. In addition, the potential degradation of biological compounds during prolonged storage means faster separation techniques are needed.The rate of separation in a suspension of particles by way of gravitational force mainly depends on the particle size and density. Particles of higher density or larger size typically travel at a faster rate and at some point will be separated from particles less dense or smaller. This sedimentation of particles, including cells, can be explained by the Stokes equation, which describes the movement of a sphere in a gravitational field. Equation (1) calculates the velocity of sedimentation utilizing five parameters [4].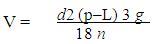 | (1) |
v = sedimentation rate or velocity of the sphered = diameter of the spherep = particle densityL = medium densityn = viscosity of mediumg = gravitational forceFrom the Stokes equation five important behaviors of particles can be explained:1. The rate of particle sedimentation is proportional to the particle size.2. The sedimentation rate is proportional to the difference in density between the particle and the medium.3. The sedimentation rate is zero when the particle density is the same as the medium density.4. The sedimentation rate decreases as the medium viscosity increases.5. The sedimentation rate increases as the gravitational force increases.Table 1. Classes of centrifuges and their applications 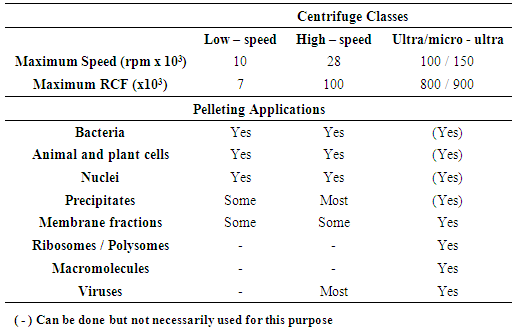 |
| |
|
RCF is dependent on the speed of rotation in rpm and the distance of the particles from the center of rotation. When the speed of rotation is given in rpm (Q) and the distance (r) is expressed in centimeters, RCF can be calculated by using the formula in Figure 2. | (2) |
Formula for relative centrifugal force (RCF).
3. Principles of Centrifugation
Particles having a size above 5m sediment at the bottom due to gravitation force. Such a suspension can be separated by simple filtration techniques. If the size of particles is less than 5m they undergo Brownian motion. In such suspension a stronger centrifugal force is applied to separate the particles.Considering a body of mass m rotating in a circular path of radius r at a velocity of v. The force acting on the body in a radial direction is given by:F = mv2/rWhere F = centrifugal force, m = mass of body, v = velocity of the body, r = radius of circle of rotation.The gravitational force acting upon the same body G = mgWhere, G = gravitational forceg = acceleration due to gravityThe centrifugal effect is the ratio of the centrifugal force and gravitational forces so that  | (3) |
Since, v = 2 r n where n = speed of rotation (r.p.m.) | (4) |
where, k = 22/g = constantD = maximum diameter of the centrifuge 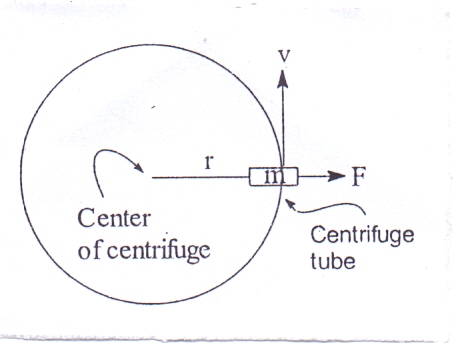 | Figure 1. Illustration of the principle of Centrifugation |
D can be measured from the center of the centrifuge to the free surface of the liquid or to the tip of the centrifuge tube.From the equation C = kDn2 it can be concluded that Centrifugal effect diameter of centrifugeCentrifugal effect (speed of rotation)2
3.1. Applications of Principle of Centrifugation
If the particles of suspensions are very small then high centrifugal effect will be required to separate the particles. To separate such suspensions the size of the centrifuge is kept smaller but it is rotated at very high speed (rpm). If a large amount of material is to be separated and a low centrifugal effect is sufficient to separate the suspension then the diameter (D) of the centrifuge is increased and speed (n) is kept low.
4. Types of Centrifugation Separations
There are two types of centrifugal techniques for separating particles, differential centrifugation and density gradient centrifugation. Density gradient centrifugation can further be divided into rate-zonal and isopycnic centrifugation.
4.1. Differential Centrifugation
The simplest form of separation by centrifugation is differential centrifugation, sometime called differential pelleting (Figure 1). Particles of different densities or sizes in a suspension will sediment at different rates, with the larger and denser particles sedimenting faster. These sedimentation rates can be increased by using centrifugal force. A suspension of cells subjected to a series of increasing centrifugal force cycles will yield a series of pellets containing cells of decreasing sedimentation rate.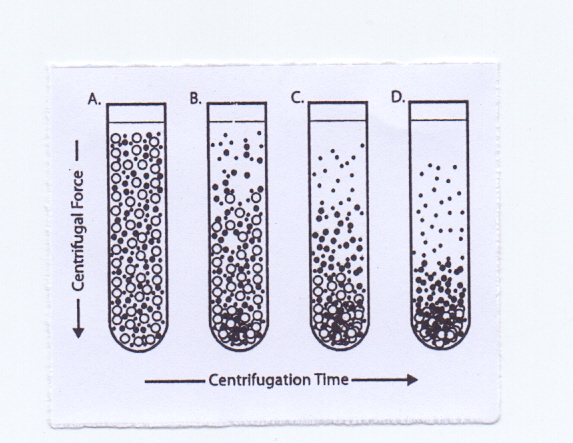 | Figure 2. Differential Centrifugation |
Particles of different densities or size will sediment at different rates with the largest and most dense particles sedimenting the fastest followed by less dense and smaller particles.Differential pelleting is commonly used for harvesting cells or producing crude subcellular fractions from tissue homogenate. For example, a rat liver homogenate containing nuclei, mitochondria, lysosomes, and membrane vesicles that is centrifuged at low speed for a short time will pellet mainly the larger and more dense nuclei. Subsequent centrifugation at a higher centrifugal force will pellet particles of the next lower order of size (e.g., mitochondria) and so on. It is unusual to use more than four differential centrifugation cycles for a normal tissue homogenate. Due to the heterogeneity in biological particles, differential centrifugation suffers from contamination and poor recoveries. Contamination by different particle types can be addressed by resuspension and repeating the centrifugation steps (i.e., washing the pellet).
4.2. Density Gradient Centrifugation
Density gradient centrifugation is the preferred method to purify subcellular organelles and macromolecules. Density gradients can be generated by placing layer after layer of gradient media such as sucrose in a tube with the heaviest layer at the bottom and the lightest at the top in either a discontinuous mode. The cell fraction to be separated is placed on top of the layer and centrifuged. Density gradient separation can be classified into two categories, rate – zonal (size) separation and isopycnic (density) separation.
4.2.1. Rate-Zonal Centrifugation
In rate-zonal centrifugation the problem of cross-contamination of particles of different sedimentation rates may be avoided by layering the sample as a narrow zone on top of a density gradient. In this way the faster sedimenting particles are not contaminated by the slower particles as occurs in differential centrifugation. However, the narrow load zone limits the volume of sample (typically 10%) that can be accommodated on the density gradient. The gradient stabilizes the bands and provides a medium of increasing density and viscosity.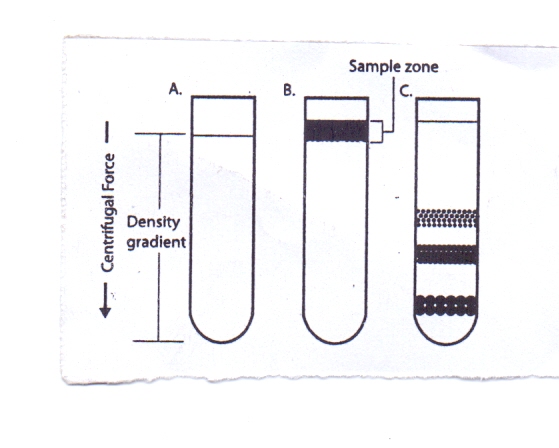 | Figure 3. Rate-Zonal Centrifugation |
Sample is layered as a narrow zone on the top of a density gradient (3B). Under centrifugal force, particles move at different rates depending on their mass (3C). The speed at which particles sediment depends primarily on their size and mass instead of density. As the particles in the band move down through the density medium, zones containing particles of similar size form as the faster sedimenting particles move ahead of the slower ones. Because the density of the particles is greater than the density of the gradient, all the particles will eventually form a pellet if centrifuged long enough.
4.2.2. Isopycnic Centrifugation
In isopycnic separation, also called buoyant or equilibrium separation, particles are separated solely on the basis of their density. Particle size only affects the rate at which particles move until their density is the same as the surrounding gradient medium. The density of the gradient medium must be greater than the density of the particles to be separated. By this method, the particles will never sediment to the bottom of the tube, no matter how long the centrifugation time.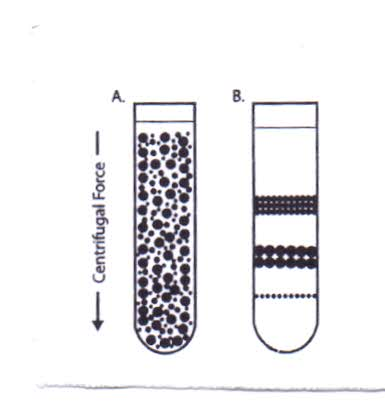 | Figure 4. Isopycnic Centrifugation |
Starting with a uniform mixture of sample and density gradient (4A) under centrifugal force, particles move until their density is the same as the surrounding medium (4B).Upon centrifugation, particles of a specific density sediment until they reach the point where their density is the same as the gradient media (i.e., the equilibrium position). The gradient is then said to be isopycnic and the particles are separated according to their buoyancy. Since the density of biological particles is sensitive to the osmotic pressure of the gradient, isopycnic separation may vary significantly depending on the gradient medium used. Although a continuous gradient may be more suited for analytical purposes, preparative techniques commonly use a discontinuous gradient in which the particles band at the interface between the density gradient layers. This makes harvesting certain biological particles (e.g., lymphocytes) easier.
4.2.3. Selection of Suitable Density Gradient Medium
The primary function of density gradient centrifugation is to separate particles, either on the basis of their buoyancy density or their rate of sedimentation. For rate-zonal separations, the function of the gradient is to provide a gradient of viscosity which improves particle resolution while stabilizing the column from convection currents. For isopycnic separations, the important feature is that the maximum density of the gradient media is higher than that of the particles.
4.2.3.1. Properties of Ideal Density Gradient Media
An ideal density gradient media should have sufficient solubility to produce the range of densities required; should not form solution of high viscosity in the desired density range; should not be hyperosmotic or hypoosmotic when the particles to be separated are osmotically sensitive; solutions of the gradient should be adjustable to the pH and the ionic strengths that are compatible with the articles being separated; should not affect the biological activity of the sample; should be nontoxic and not metabolized by cells; not interfere with assay procedures or react with the centrifuge tubes; should exhibits a property that can be used as a measure of concentration; should be easily removed from the purified product; autoclavable and possess reasonable cost. No single compound can satisfy all of the above criteria. As shown in Table 2, a wide range of gradient media are used for the different types of samples. Most media are capable of producing the range of densities required and being easily removed from the particles of interest. The effect of osmolality on biological particles requires special consideration. The osmolality of most mammalian fluids is 290-300 mOsm.4. This is the osmolality of balanced salt solutions (e.g., 0.85-0.9% NaCl) and most common media. High osmolality solutions not only remove water from the interior of membrane-bound particles, they also remove water bound to macromolecules like DNA. Loss of water from cells will reduce their size and increase their density, thereby affecting their buoyancy and rate of sedimentation. The osmotic effect on cells and macromolecules may be reversible, though it is a possible source of error that should be avoided.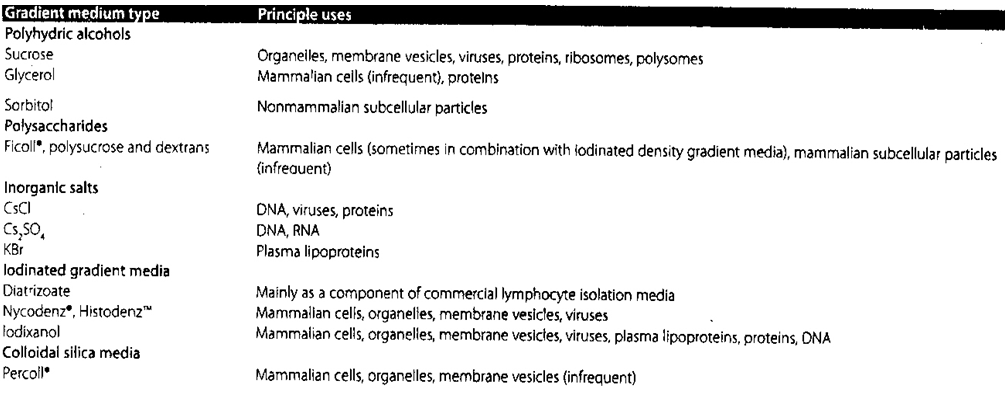 | Table 2. Density Gradient Media Types and their Principle Uses |
Over the years, a variety of different compounds have been developed as density gradient media in order to enhance the separation process and to overcome osmolality and viscosity problems. There are five main classes of density gradient medium. These are Polyhydric (sugar) alcohols, Polysaccharides, Inorganic salts, Iodinated compounds, Colloidal silica.
4.2.4. Polyhydric Alcohols
Polyhydric alcohols are considered nonionic gradient media. Some of the first centrifugation techniques developed in the 1950s used sucrose in the purification of cell organelles. Sucrose gradients are widely used for the rate-zonal separation of macromolecules and for the isopycnic separation of viruses and cell organelles. The advantages are its stable nature, inertness, and low cost. The disadvantageslie in the fact that concentrated solutions are viscous and hypertonic. Reagent-grade sucrose may be contaminated with RNAses or heavy metals and therefore are unsuitable for DNA and RNA purification. Glycerol solutions are less dense than corresponding sucrose solutions. However, glycerol solutions of the same density of sucrose solutions are much more viscous. Glycerol helps to preserve the activity of certain enzymes and it can be removed through vacuum.Examples are: Sucrose Organelles, membrane vesicles, viruses, proteins, ribosomes, polysomes Glycerol Mammalian cells (infrequent), proteins Sorbitol Nonmammalian subcellular particles.
4.2.5. Polysaccharides
These include: Ficoll®, polysucrose and dextrans Mammalian cells (sometimes in combination with iodinated density gradient media), mammalian subcellular particles (infrequent).
4.2.6. Inorganic Salts (Ionic Metal Salts)
Ionic gradient media, comprised of concentrated heavy metal salts, are almost exclusively used for isopycnic separations of nucleic acids.6 Cesium chloride and cesium sulfate are the most widely used heavy metal salts with gradient densities of up to 1.91 g/cm3. Other useful salts include sodium iodide, sodium bromide and the rubidium salts. The steepness and shape of the ionic density gradient formed depends on the centrifugal force and type of rotor respectively. Ionic gradient media are highly ionic and non-viscous with high osmolarities. It should be kept in mind that the density of the sample is highly dependent on the sample's hydration, which in turn depends on the dehydration power of the ionic gradient media.Examples are: CsCl, DNA, viruses, proteins Cs2SO4 DNA, RNA, KBr and Plasma lipoproteins
4.2.7. Nonionic Iodinated Density Gradient Media
The iodinated aromatic compounds, originally devised for X-ray contrast applications, solve the more serious deficiencies of the other classes of gradient media. Iodinated gradient media have much lower osmolarities and viscosities than sucrose at any concentration. Polysaccharides such as Ficoll® are even more viscous than sucrose at all densities. Ionic gradient media, such as cesium chloride, have higher densities and lower viscosities than other density gradient media. However, their use is restricted due to the high osmolarities and ionic strength which affect the hydration of osmotically sensitive particles and can disrupt or otherwise modify the integrity of biological particles. For example, diatrizoate mainly is used as a component of commercial lymphocyte isolation media. Nycodenz®, Histodenz™ Mammalian cells, organelles, membrane vesicles, viruses Iodixanol Mammalian cells, organelles, membrane vesicles, viruses, plasma lipoproteins, proteins, DNA Colloidal silica media Percoll® Mammalian cells, organelles, membrane vesicles (infrequent).
5. Classification of Industrial Centrifuges
A. Perforated bowl or filter types1. Batch type(a) Top – driven(b) Under – driven2. Semicontinuous3. ContinuousB. Solid – bowl or sedimentation types1. Vertical(a) Simple bowl(b) Bowl with plates2. Horizontal(a) Continuous decanters
5.1. Batch Type Top Driven Centrifuge
5.1.1. Construction of Batch Type Top Driven Centrifuge
This consists of a rotating basket susp4ended on a vertical shaft and driven by a motor from top. The sides of the basket are perforated and are also covered with a screen on the side. Surrounding the basket is a stationary casing that collects the filtrate.
5.1.2. Working Batch Type Top Driven Centrifuge
This machine is a batch-type machine. The material (suspension) is put into the basket. Then power is applied. The basket accelerates to its maximum speed. The particles and liquid are thrown by centrifugal force to the wall of the basket. The liquid passes out through the screen and the solid particles retained on the screen as deposit. After a definite time the power is turned off, a brake brought to rest. The discharge valve at the bottom of the basket is raised, and the deposited solid is cut from the side of the basket into the opening.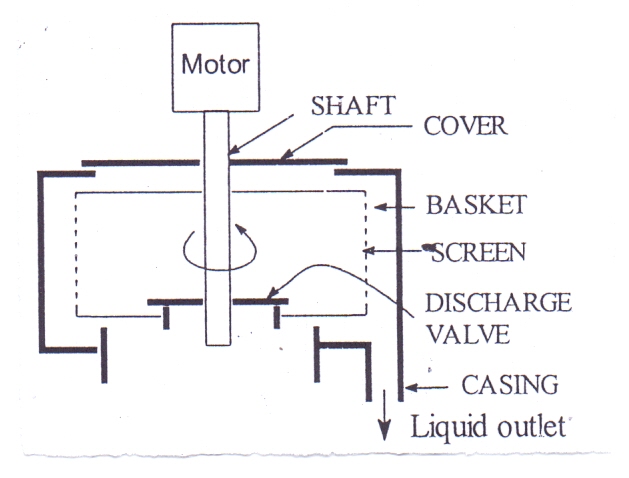 | Figure 5. Top-driven batch centrifuge |
Use:1. Crystal can be separated from mother-liquor2. Liquids can be clarified by removing unwanted solids dirt from oils.
5.2. Batch Type under Driven Centrifuge
5.2.1. Construction Batch Type under Driven Centrifuge
Batch type under driven centrifuge consists of a rotating basket place on a vertical shaft and driven by a motor from bottom. The sides of the basket are perforated and are also covered with a screen on the inside. Surrounding the basket is a stationary casing that collects the filtrate.
5.2.2. Working Batch Type under Driven Centrifuge
In this machine, the material (suspension) is put into the basket. Then power is applied. The basket accelerates to its maximum speed. The particles and liquid are thrown by centrifugal force to the wall of the basket. The liquid passes out through the screen and the solid particles retained on the screen as deposit. After a definite time the power is turned off, a brake applied, and the basket brought to rest. The cover at the top of the basket is raised, and the deposited solid is cut from the side of the basket and collected.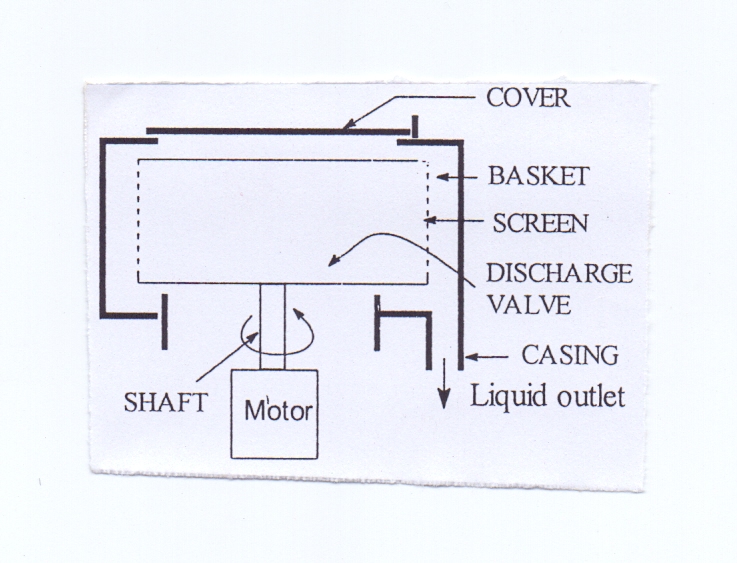 | Figure 6. Bottom-Driven Batch Centrifuge |
6. Types of Centrifuges
Centrifuges achieve separation by means of the accelerated gravitational force achieved by a rapid rotation. This can either replace normal gravity in the sedimentation of suspension or provide the driving force in the filtration through a filter medium of some kind.The most common application is separation of solid substances from high concentrated suspensions. Used in this way for the treatment of sewage sludge it enables the dewatering with the production of more or less consistent sediment depending on the nature of the sludge to be treated, and the accelerated thickening of low concentration sludge.
6.1. Principle
The separation is similar in principle to that achieved in a gravity separation process. The driving force is higher because is resulting from the rotation of the liquid: in the case of sedimentation, where the driving force is resulting from the difference in density between the solids particles and the liquid, the separation is achieved with a force from 1000 to 20000 times that of gravity.
6.2. Types of Centrifuges
Most centrifuges rotate thanks to some kind of motor drive. The types of centrifuge used for sedimentation include: hydrocyclone, tubular bowl, chamber bowl, imperforate basket, disk stack separator and decanter. Sedimenting centrifuges were invented for liquid solid separation and not for handling solids. It soon became apparent that these machines had wider applications, which would involve the presence of solid impurities, leading to use for separating solids from liquids.
6.2.1. Hydrocyclone
The simplest device to use centrifugal force to achieve separation is the hydrocyclone. It not really a centrifuge: the centrifugal separation is produced by the motion of the slurry, induced by the tangential introduction of the feed material. Its principle of operation is based on the concept of the terminal settling velocity of a solid particle in a centrifugal field. The following picture describes the conditions in an operating hydrocyclone.The feed enters tangentially into the cylindrical section of the hydrocyclone and follows a circulating path with a net inward flow of fluid from the outside to the vortex finder on the axis. The centrifugal field generated by the high circulating velocities creates an air core on the axis that usually extends on the spigot opening at the bottom of the conical section through the vortex finder to the overflow at the top. In order for this to occur the centrifugal force field must be several times larger than the gravitational one. Particles that experience this centrifugal field will tend to move outwards relative to the carrier fluid because of their relatively greater density. The larger, heavier pqarticles will migrate rapidly to the outside walls of the cylindrical section and will then be forced to move downward to the inside of the conical wall. Small particles will, on the other hand, be dragged inward by the fluid as it moves towards the vortex finder. The solid separation occurs in the passage of the suspension along the barrel of the hydrocyclone, to form thickened slurry at the outer wall, which than leaves the hydrocyclone as a continuous stream from its discharge nozzle.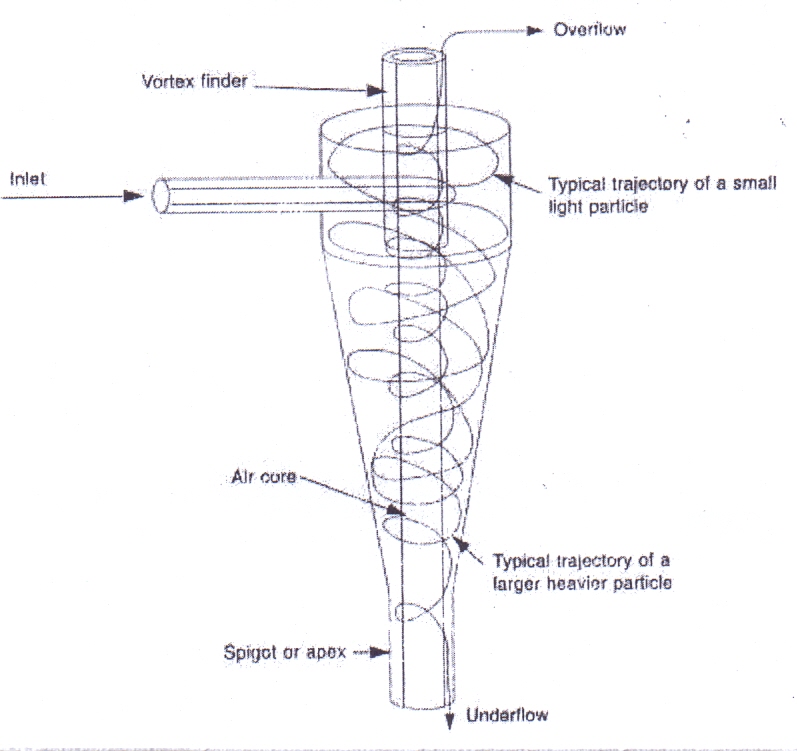 | Figure 7. Hydrocylone |
6.2.2. Tubular Bowl Centrifuge
The tubular bowl centrifuge has been used for longer than most other designs of centrifuge. It is based on a very simple geometry: It is formed by a tube, of length several times its diameter, rotating between bearings at each end. The process stream enters at the bottom of the centrifuge and high centrifugal forces (let to separate out the solids. The bulk of the solids will adhere on the walls of the bowl, while the liquid phase exits at the top of the centrifuge. As this type of system lacks a provision of solids rejection, the solids can only be removed by stopping the machine, dismantling it and scraping or flushing the solids out manually. Tubular bowl centrifuges have dewatering capacity, but limited solids capacity. Foaming can be a problem unless the system includes special skimming or centripetal pumps.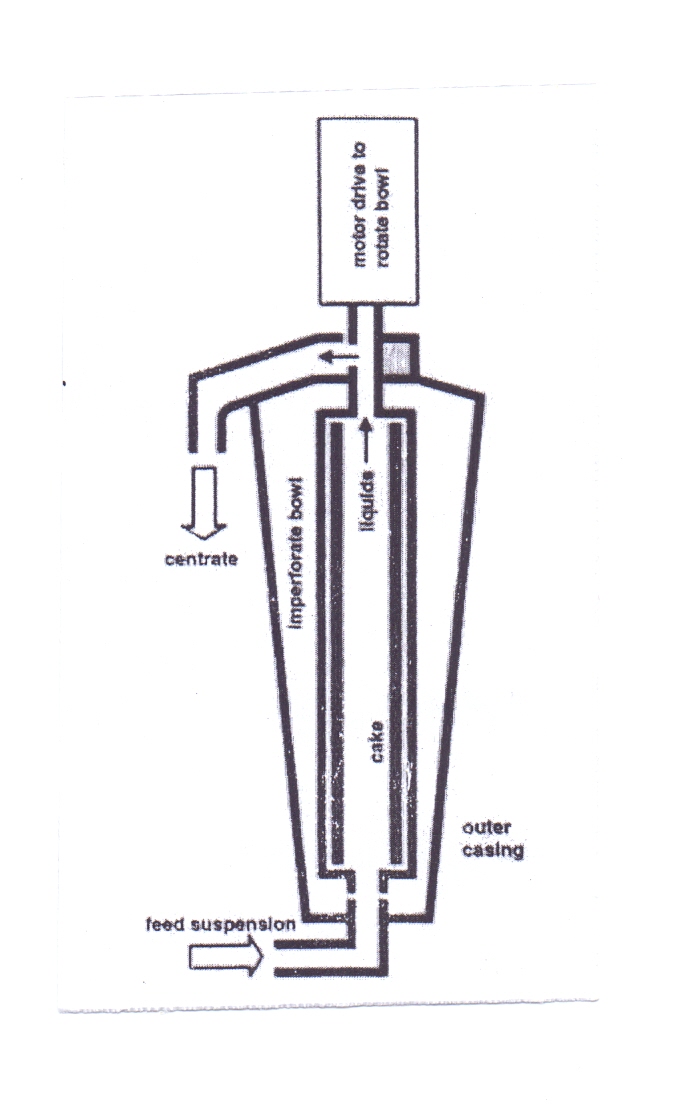 | Figure 8. Tubular Bowl Centrifuge |
6.2.3. Chamber Bowl Centrifuge
The chamber bowl centrifuge is a number of tubular bowl arranged co-axially. It has a main bowl containing cylindrical inserts that dived the volume of the bowl into a series of annular chambers, which operate in series. Feed enters the center of the bowl and the suspension passes through each chamber in turn, at increasing distances from the axis. The solids settle onto the outer wall of each chamber and the clarified liquid emerges as an overflow from the largest diameter chamber. This device provides also a classification of the suspended solids: the coarse particles deposit in the inner chamber and the increasingly fine particle deposit on the subsequent chambers. The removal of sedimented solids requires the stopping of rotation for manual cleaning.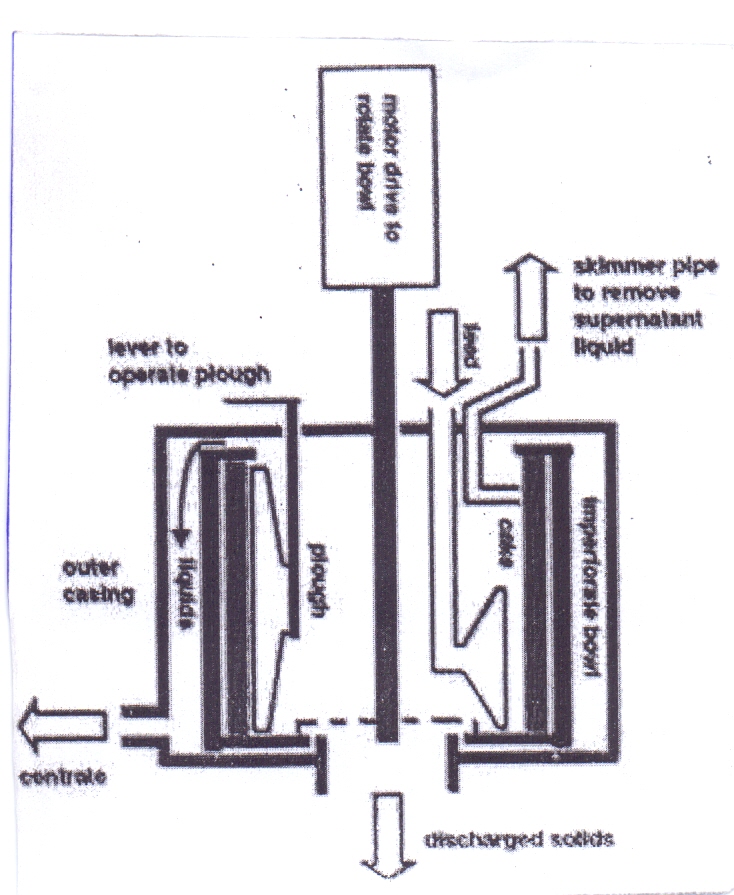 | Figure 9. Chamber Bowl Centrifuge |
6.2.4. Disk Stack Separator
The simplest design is a closed bowl, containing the disk stack, with any solids present collecting at the outer part of the bowl, from which they have to be removed manually after stopping rotation. The solids are discharged from the bowl by a number of methods, including the basic use of nozzles, which are open continuously, allowing thick slurry to discharge. In the more complicated design valved nozzles open automatically when the solid depth in the bowl reaches a certain value, and then close again when most of the solids have been discharged. In the most complicated design the bowl is opened: its shell splits circumferentially for a short period, with the opening also controlled by solids depth in the bowl.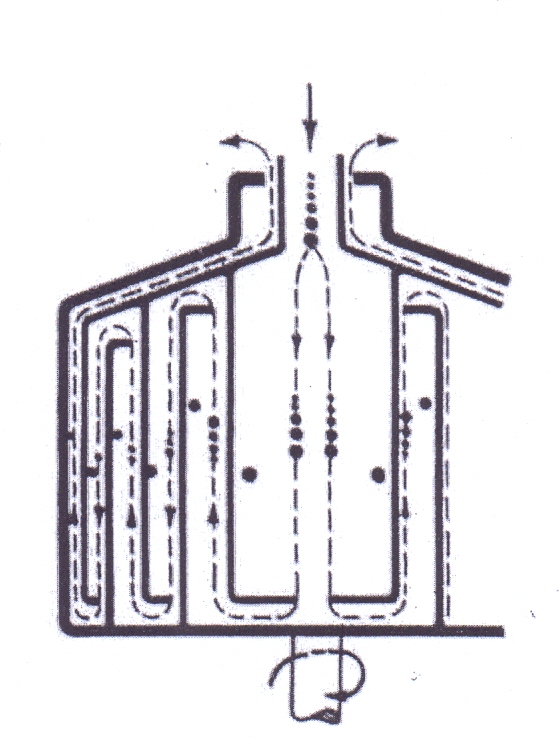 | Figure 10. Disk Stack Separator |
6.2.5. Imperforate Basket Centrifuge
The imperforate basket centrifuge is used when if the solid content of the suspension is higher. It consists of a simple drum-shaped basket or bowl, usually rotating around a vertical axis. The solids accumulate and compress as effect of the centrifugal force, but they are not dewatered. The residual liquid is drained out when the rotation of the bowl is stopped. The layer of solids is removed manually by scraping or shoveling. Unloading can be achieved semi automatically first by use of a skimmer pipe to remove the residual liquid and then by lowering a knife blade into the solid and so cutting it out from the bowl. This allows avoiding the switching off of the machine.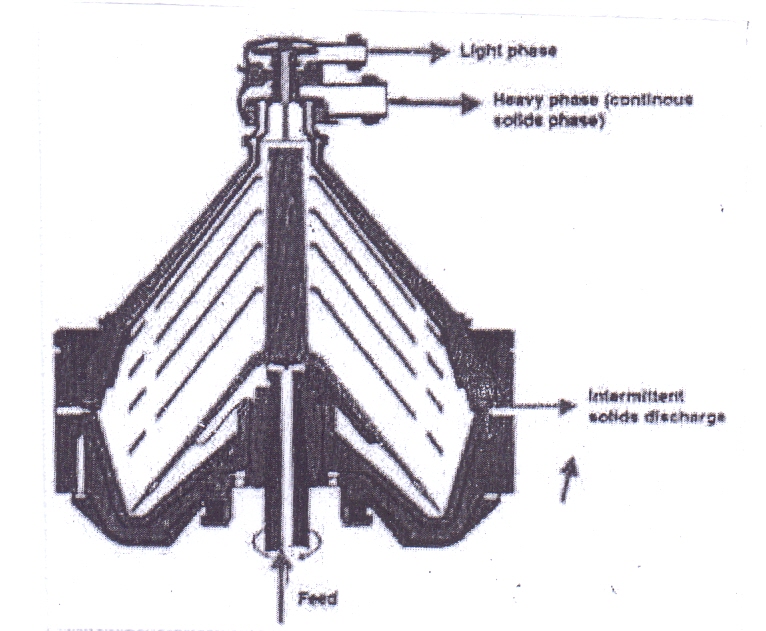 | Figure 11. Imperforate Basket Centrifuge |
6.2.6. Decanter
The decanter centrifuge is the only sedimentation centrifuge designed from the start to handle significant solid concentration in the feed suspension. At the same time it can achieve quite good degrees of clarification of the liquid concentrate. Although a complicated piece of machinery it embodies a simple principle. They consist basically of a horizontal cylindrical bowl (1) rotating at a high speed, with a helical extraction screw (2) placed coaxially. The screw perfectly fits the internal contour of the bowl, only allowing clearance between the bowl and the scroll. The differential speed between screw and scroll provides the conveying motion to collect and remove the solids, which accumulate at the 0bowl wall.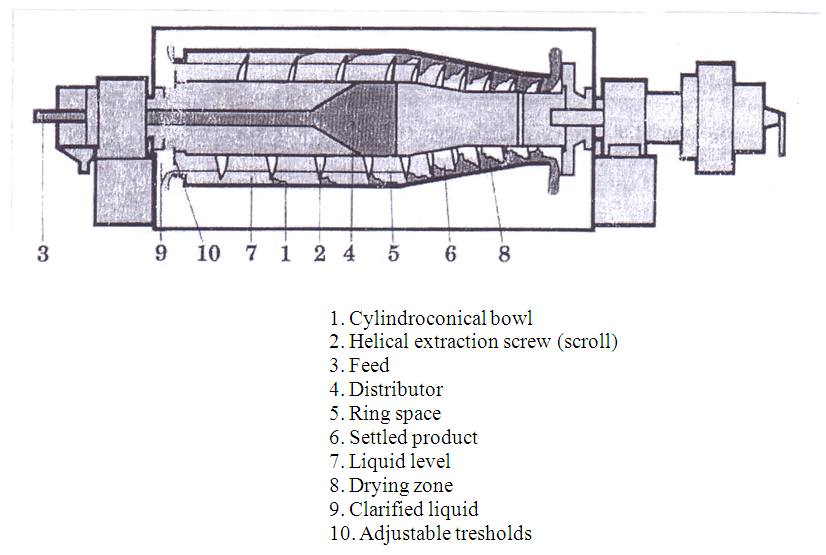 | Figure 12. Decanter centrifuge |
The product to be treated (3) is introduced axially into the unit by appropriate distributor (4). It is propelled into the ring space (5) formed by' the internal surface of the bowl and the body of the scroll. The separation process basically takes place inside the cylindrical section of the bowl. The relative velocity of the scroll pushes the settled product (6) along into the bowl. The conveyance of the solids into the length of the cone enables the sediment to pass out of the clarified liquid phase. As the feed is continuous a liquid level (7) is established in the unit following a cylindrical surface that constitutes the internal surface of the liquid ring. Once the solids have passed out of the liquid ring the remaining section of the cone all the way up to the ejector provide the final draining: this section is known as the drying zone (8). The clarified liquid (9) is collected at the other end of the bowl by flowing through the adjustable threshold (10), which restricts the liquid ring of the unit. A cover that enables the clarified liquid as well as the sediments to be collected protects the rotor.The decanter operates mainly by sedimentation a process causing the separation of suspended solids by virtue of their higher density than the liquid in which they are suspended. If the density difference is high than gravity may provide sufficient driving force for the separation to occur in a reasonable time. If the density difference is small, or the particle size is very small, than gravity separation would take too long and the separation force must be increased by the imposition of centrifugal forces many times that of gravity alone.
6.2.6.1. Advantages of Decanter
The prime beneficial characteristic of the decanter is its ability to remove separated solids from the separation zone on a fully continuous basis.By comparison with:● Gravity sedimentation: the decanter can achieve separations that would be very difficult in a clarifier or lamella separator. and it produces drier solids.● Hydrocyclones: the decanter has a much higher liquid capacity, can handle much higher slurry concentrations and producer much drier solids.● Tubular bowl centrifuges: the decanter offers higher capacities, the ability to handle concentrate slurries, and continuous operation.● Imperforate basket centrifuges: the decanter operates continuously, can handle much higher solids concentrations, and produces much drier solids.● Disk stack centrifuge: the decanter is truly continuous in operation, can handle much higher solids concentrations in the feed slurry and produces drier solids.The advantages of the decanter are its wide range of potential use, coupled with its continuous operation, its ability to accept a wide range of feed concentrations, and its availability in a wide range of feed capacities.
6.2.6.2. Applications of Decanter Centrifuge
The decanter centrifuge can be used for most types of liquid/solid separation. It can be used for the classification of solids in liquid suspension or for the clarification of liquids. It can also be used in the recovery of a valuable solid from its suspension in the liquid and it can wash the recovered solid from its mother liquor. The decanter can also dewater slurries to a high level of dryness and it can finally be operated so as to act as a thickener, producing clear liquid and more concentrated slurry.
7. Applications of Centrifugation in Pharmaceutical Industries
1. Production of bulk drugsAfter crystallization the drugs are separated from the mother liquor by centrifugation. For example traces of mother liquor is separated from aspirin crystals by centrifugation method.2. Production of biological productsMost of the biological products are either proteinaceous or macromolecules. During manufacturing they remain in colloidal dispersion in water. By normal methods of filtration it is difficult to separate the colloid particles. In those cases centrifugal methods are used. Insulin is purified from other precipitates of protein materials by centrifugation. Blood cells are separated from plasma by centrifugal method. Bacterial enzymes are separate from bacterial culture medium by sedimenting the bacterial cells by centrifugation. Dirt and water are separated from olive oil and fish-liver oils.3. Evaluation of suspensions and emulsionsOne of the problems of suspensions is sedimentation and one of the problems of emulsions is creaming. Immediately after the preparation of a suspension or emulsion this problems may not appear quickly. To enhance the rate of sedimentation and creaming the suspension or emulsion is introduced in a centrifuge and rotate at an rpm of 200 to 3000. If still the problems do not appear then the suspension or emulsion can be taken as stable formulation.4. Determination of molecular weight of colloidsPolymers, proteins and such macromolecules often form colloidal dispersions. The molecular weights of those molecules can be determined by ultracentrifugation. The larger molecules will be arranged at periphery and the lighter molecules near the center.
8. Conclusions
This review has provided insight into the importance of centrifugation in the pharmaceutical industry. Bulk drugs and biological products are produced through centrifugation. Purification of insulin and separation of blood cells are separated through the process of centrifugation. Centrifugation enhances the rate of sedimentation of suspensions and creaming of emulsions.
ACKNOWLEDGMENTS
I wish to acknowledge the immense contributions of Biofilesonline, Sigma – Aldrich for making their literatures available for this review. Special thanks go to their Technical Marketing Specialist, Mr. Mark Frei.
References
[1] | D. Rickwood and J. M. Graham (2001); Biological Centrifugation, Springer Verlag; ISBN: 0387915761. |
[2] | D. Rickwood, T. Ford, J. Steensgaard (1994): John Wiley & Son Ltd. ISBN: 0471942715. |
[3] | T. C. Ford and J. M. Graham (1991): An Introduction to Centrifugation, BIOS Scientific Publishers, Ltd. ISBN 1 872748 40 6. |
[4] | D. Rickwood (1992): Centrifugation: A Practical Approach ASIN: 090414755X.. |
[5] | D. Rickwood and J. M. Graham (1997): Subcellular Fractionation: A Practical Approach, Oxford University Press. ISBN: 0199634947. |
[6] | Biological Centrifugation, by D. Rickwood, I.M. Graham (2001). Springer Verlag; ISBN: 0387915761. |
[7] | Sub cellular Fractionation: A Practical Approach, by John M. Graham (Editor) and D. Rickwood (Editor) (1997). Oxford Univ Press. ISBN: 0199634947. |
[8] | Centrifugation: Essential Data, by David Rickwood, T. Ford, Jens Steensgaard (1994). 128 pages. John Wiley & Son Ltd. ISBN: 0471942715. |
[9] | Centrifugation: A Practical Approach, by David Rickwood, (Editor) (1992) ASIN: 090414755X. An Introduction to Centrifugation, by TC. Ford and lM. Graham (1991). 118 pages. BIOS Scientific Publishers, Ltd. ISBN I 872748406.. |