Mark Pitney 1, Elizabeth van Niekerk 1, Socrates Dokos 2, Matthew Pelletier 3, William R. Walsh 3
1Eastern Heart Clinic, Prince of Wales Hospital, Sydney, 2031, Australia
2Graduate School of Biomedical Engineering, University of NSW, Sydney, 2052, Australia
3Surgical and Orthopaedic Research Laboratories, Prince of Wales Clinical School, University of NSW, Prince of Wales Hospital, Sydney, 2031, Australia
Correspondence to: Mark Pitney , Eastern Heart Clinic, Prince of Wales Hospital, Sydney, 2031, Australia.
Email: |  |
Copyright © 2012 Scientific & Academic Publishing. All Rights Reserved.
Abstract
Information on stent longitudinal strength is limited to benchtop models using unconstrained stents, consequently having uncertain clinical significance. This study investigated the effects of stent apposition and tube (artery) compliance on stent stability. Multilink Vision, Multilink 8, Promus Element, Driver and Integrity were examined. Stent size was 3/18 mm or closest length. Four scenarios were tested: in three the stents were well deployed and in the final test, stents were purposely severely malapposed. In the well-deployed models, three tubes with different compliance were tested. Stents were compressed by applying a single point force. Malapposed stents compressed extensively at 50 and 100 gm., especially Element, which deformed double the amount of the other stents. At higher forces (150 gm.), the magnitude of difference was reduced. Stent apposition had a profound protective effect and limited differences between stents. Stiffer tubes provided more protection to stent deformation. All stents are vulnerable to deformation when severely malapposed but the Element is significantly more so. Well-apposed stents are considerably more resistant to longitudinal compression such that differences between stents become minimal. More rigid tubes offered greater protection. Clinical implications regarding stent sizing and postdilation strategy are significant.
Keywords:
Coronary Stents, Longitudinal Compression, Apposition, Vessel Compliance
Cite this paper: Mark Pitney , Elizabeth van Niekerk , Socrates Dokos , Matthew Pelletier , William R. Walsh , Longitudinal Stent Deformation: Importance of Stent Type and Stent Apposition, American Journal of Biomedical Engineering, Vol. 3 No. 3, 2013, pp. 63-69. doi: 10.5923/j.ajbe.20130303.03.
1. Introduction
To maximize the efficacy and deliverability of coronary stents, their design has traditionally focused on features such as radial strength, recoil, shortening, deliverability, strut thickness and resistance to stent thrombosis. The concept of stent longitudinal strength is relatively new, and though rare, longitudinal stent compression may have devastating effects (1). When stents are initially deployed, the lumen often remains narrowed at the site of severest stenosis while elsewhere, the stent may not be fully apposed to the vessel wall. This increases the feared complication of stent thrombosis and consequently, it is common for operators to pass a new balloon through a recently deployed stent to further expand it.Clinical experience with and consequences of longitudinal deformation have been reviewed elsewhere (2, 3). Recently, case reports have been published of stents that severely deformed longitudinally when operators attempted to pass new balloons through the stents (1, 4, 5). The likely cause was that the balloon catheter tips snagged and exerted a longitudinal force on the stent. Certain stent designs may be more vulnerable than others. Although the original clinical report involved stent testing within tubes (5), recent studies have comprised benchtop models using completely unconstrained stents (6, 7). While elegant and establishing that stents have intrinsic differences, the clinical applicability of these data is arguable. The magnitude of this effect has not been previously studied. This study attempted to mimic clinically relevant scenarios by testing well deployed stents in tubes of various compliances and comparing these to a purposely malapposed model using undersized stents. It was expected that the results would be of clinical interest to all operators, especially those treating increasingly complex lesions, where this phenomenon is more likely (5). Arterial compliance has the potential to greatly influence the apposition, stability and resistance to deformation of the stents placed within them. This study also attempted to quantify this influence.
2. Materials and Methods
The 5 stent platforms most frequently used in our institute over the last 3 years were tested (Table 1). As stent platforms have different names around the world, stents are referred to as listed in Table 1 hereafter. When available, the bare metal versions were tested to reduce costs. Stent compression was tested by deploying stents in silastic tubes as detailed below. A single point of force model was employed to mimic the most common clinical scenarios of a postdilation balloon or IVUS catheter catching on to a stent (8). In vivo, stents can be compressed by an anterograde force (e.g. post dilation balloon) or retrograde force (e.g. withdrawal of an IVUS catheter or filter). This study used a retrograde force by deploying stents with a 3-0 monofilament nylon 45cm long suture (Ethicon, J&J, New Jersey, U.S.A.) between the stent and the tube. A free end of the suture was brought through the lumen of the stent such that a loop was formed, which subsequently applied force to a single point on the most distal strut. This model was found to be highly reliable in the design phase as opposed to hooks or rods. The connecting suture was attached to the load cell on a MACH 1 Micromechanical Testing Machine (BIOSYNTECH, Laval, Quebec, Canada). The load cell was configured such that it would move upwards at a constant velocity of 0.1mm/s. Load (grams, gm.) and time (milliseconds) data were recorded at 20 Hz. In the first case, the intrinsic strength of the stents was tested using deployment in an oversized tube such that severe malapposition occurred. The 3.0 mm stents were deployed just under the Rated Burst Pressure of the balloons in an oversized 4.0mm lumen diameter tube. One end of the stent was fixed to prevent migration while force was applied to the other end of the stent. Table 1. Tested Stents 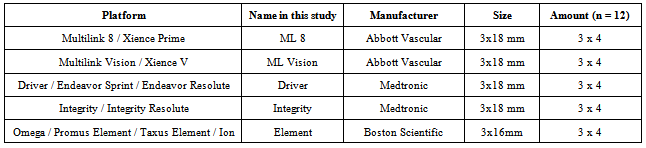 |
| |
|
Table 2. Models Tested 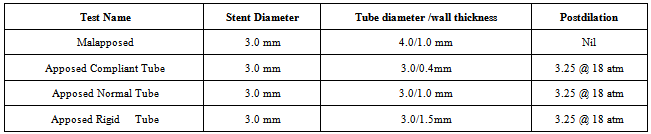 |
| |
|
To model the three other scenarios, stent apposition was ensured by deploying 3.0mm stents in a 3.0mm tube at nominal pressure but then postdilating at 18 atm with a 3.25mm non-compliant balloon (3.25mm NC Hiryu, Terumo, Tokyo, Japan) to standardize apposition. At 18 atm, the postdilation balloon was approximately 0.4 mm larger than the tube lumen. Each stent type was tested three times for each of the four apposition scenarios; severely malapposed, and three scenarios in which the stents were apposed to tubes of three different compliances. Figure 1 shows an example of deformation of well-apposed and severely malapposed stents. Table 2 summarizes the testing methodology. 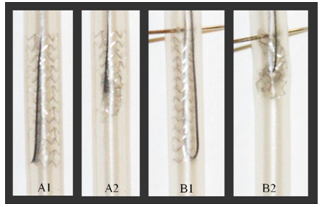 | Figure 1. Examples of well-apposed stents prior to (A1) and after (A2) deformation, and severely malapposed stents prior to (B1) and after (B2) deformation. The needle in the B-panels acted as an obstruction to deformation. All stents showed a difference between the apposed version of the stent (panels A1 and A2) and the severely malapposed version of the stent (B1 and B2) |
Silicone tubes of 3mm lumen diameter (GeckoOptical, Joondalup, WA, Australia) were selected to represent a range of possible coronary arterial compliances. Concurrently with stent testing, it was confirmed that the tubes had differences in compliances by using IVUS and a haemodynamic monitoring system and measuring tube lumen from 80 to 200 mmHg, increasing pressure in increments of 20 mmHg. The 0.4mm thick tube had a diametric compliance of 12.5%/100 mmHg; the 1.0mm thick tube had compliance of 5.3%/100 mmHg and the 1.5mm thick tube had a compliance of 1.05%/100 mmHg. This range in compliance attempted to reflect normal, highly compliant and stiff ateries respectively. Tubes were compared to each other by measuring compression of all stents at 50, 100 and 150 gm. and calculating a “global average” quantity for each tube. Serial photographs were taken at 5 second intervals with a Canon EOS 500D with a macro lens (Canon Inc., Ohta-ku, Tokyo, Japan). Image analysis was performed using ImageJ (Rasband, W.S., ImageJ, U. S. National Institutes of Health, Maryland, U.S.A.), to visually determine changes in length. The linear distance between the upper end of the stent and the point where force was applied was measured by one observer for all image stacks. Care was taken to ensure repeatability by measuring a distance parallel to the sides of the stent. Subsequently, stent compression was calculated as the change in length divided by the original length, and was expressed as a percentage. Image resolution was approximately 25 pixels per mm. Changes in stent length were combined with time and force data as produced by the MACH 1 Micromechanical Testing Machine. As this was a single point of force experiment, the stents did not deform uniformly (as was the case with previous bench top studies which used rim compression), but instead, the distance of maximum deformation was noted. Linear interpolation was used to determine quantities of force and compression as required. Subsequent to testing, stent lumens were photographed with a Dyonics Xenon XL light source and cable receptacle (Smith and Nephew, London, UK) to determine the extent of lumen obstruction after stents were compressed. One-way ANOVA was performed with Minitab® Statistical Software1 (Minitab Inc., State College, Pennsylvania, U.S.A.) to determine the effects of apposition, tube compliance and stent design, and p < 0.05 was considered significant. One experimental outlier was deleted for apposed ML8s at 150 gm.
3. Results
3.1. General Observations
Well apposed stents displayed characteristic deformation of the stent ‘rim’ bending around into the lumen. Malapposed stents displayed significantly more deformation, with examples of well-apposed and malapposed stents shown in Figure 2. Apposition provided significant protection and tube compliance was an important variable.
3.2. Malapposed Stent Testing
This test assessed the intrinsic strength of malapposed stents. All stents suffered considerable deformation (Figure 2). At lower forces (50 and 100 gm.), the Element design was significantly more deformable whereas the remaining stents all behaved similarly. Compression at 50 gm. was 46.7±8.8% (mean ± SD, Element) vs. 18.6±2.8% (mean ± SD of other 4 stents, p = 0.00292) and at 100 gm. was 74.6±1.4% vs. 34.8±6.8% (p = 0.032). At 150 gm., however, the differences between the stents were considerably reduced.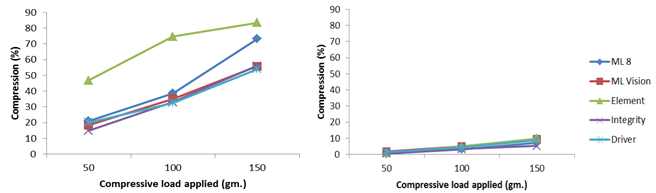 | Figure 2. Compression for malapposed (left) vs. well-apposed stents in tubes of 1.0mm thickness (right) shown to same scale. Note the profound protective effect of apposition such that any well deployed stent is much stronger than any poorly deployed stent regardless of stent type or compressive force |
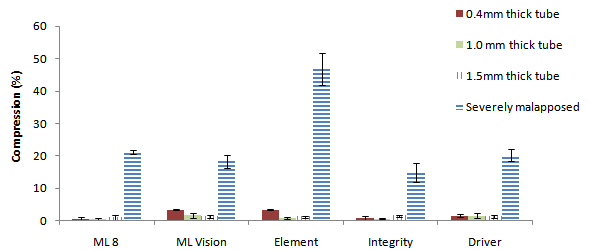 | Figure 3. Compression at 50gm. force applied. Severely malapposed stents are compared with stents apposed to vessel walls of different thicknesses (compliances). Error bars are standard error |
3.3. Apposed Stent Testing
Apposition had a dramatic effect on stent stability and longitudinal strength. All well apposed stents deformed by less than 5% at an applied load of 50 gm. regardless of the compliance of the tube in which they were deployed (Figure 2). Element (most vulnerable in unconstrained tests), showed an approximate tenfold improvement in longitudinal strength when apposed such that there was considerable equalising in its performance relative to other stents. A comparison of compression for different designs at 50 gm. load applied is displayed in Figure 3, showing the dependence of stent compression on stent type (particularly for malapposed stents), as well as vessel compliance in the three apposed models.In the most compliant tube, compression for Element continued to be greater than other stents but only at higher loads. At 150 gm., Element compressed by 35.4±10.9%, vs. 23.4±5.3%, for other stents (p =0.16).In the 1.0 and 1.5mm thick tube models, there was no statistical difference between stents at 50 gm. (p = 0.364 in 1.0mm thick tube, p = 0.973 in 1.5mm thick tube). At higher forces, stents behaved differently but absolute differences were small (2-4% only at 100gm regardless of tube) and there was no stent with clear superior or inferior performance to other stents (p > 0.09 for all stents in 1.0 or 1.5mm thick tubes at 100 or 150gm.)
3.4. Tube Compliance
Global average stent compression (as defined in methods) was 4% for the tube with usual compliance (1.0 mm wall thickness). Increasing the compliance of the tube (e.g. reduction in stiffness) resulted in greater vulnerability with the 0.4mm wall thickness tube having an average stent compression of 12% (p < 0.0001). However, making the tube stiffer than normal (less compliant, wall thickness 1.5mm) offered no additional protection with average stent compression of 5% (p = 0.218). Results are displayed in Figure 4.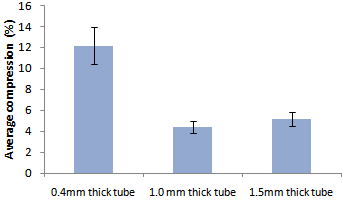 | Figure 4. Compression averaged over all stents and all loads for well-apposed stents in tubes of different thickness (compliance). Error bars are standard error |
4. Discussion
This study confirmed that intrinsic differences exist between stents with the Element being approximately twice as vulnerable to deformation as other stents when malapposed. It is noteworthy that all stents are vulnerable to stent deformation when malapposed if sufficient force is applied. Although it is unlikely that stents would be entirely malapposed in vivo, long stents sized to the distal vessel in arteries that taper are likely to be poorly apposed. Additionally, sometimes when GTN is administered post stent insertion it becomes apparent that the stent is undersized. Operators should recognise these situations as being of higher risk for deformation and should a balloon not pass easily, operators are recommended to assume malapposition and change to a lower profile semi compliant balloon and ensure apposition at moderate pressure then return with a high pressure non compliant balloon if required.The intrinsic differences between stent types became less important once stents were well deployed as the associated embedding and frictional forces dominated stent intrinsic strength in this study. A limitation to this assumption is that this study used silastic tubes which may have different frictional and embedding properties to diseased human arteries. Nevertheless, apposition is clearly protective; although the degree of protection may be over or understated by this study. If vulnerability to deformation affects an operator’s stent choice (other factors such as deliverability and conformability may be equally important), then there should be equal focus on ensuring apposition. In terms of tested applied load, the region of interest has been extended from the previously deemed ‘clinically relevant force’ of 50 gm. to include 100 gm. and 150 gm. This was done both to evaluate stents under circumstances with more extreme loads, and to include a margin of error around the applied load previously estimated at 50 gm., the clinical relevance of which is potentially limited by subjectivity.The potential effect of vessel compliance was a secondary aim of this study, and was tested by using 3 silicone tubes of varying compliance (1-12%/100mmH). It was hypothesised that stiffer tubes would allow more embedding of stents and thus offer greater protection. Generally speaking, the results support this hypothesis insomuch as the most compliant tube offered the least protection. However, there was little difference between the 1.0 and 1.5mm thick tubes, suggesting that the effect of arterial compliance may plateau. Arteries with coronary artery disease are usually less compliant than healthy arteries. There are many factors affecting arterial compliance, including age and sex, applied pressure, diabetes, obesity, plaque type, area and eccentricity, type of coronary artery disease (stable/unstable), and others (9-13). Published studies report compliance for normal human coronary arteries to be between 6 and 9%/100mmHg (6.2%, (14), 8·0% (15), 8.85% (16)). The compliances of our tubes thus reflect normal arterial compliance, lower than normal and greater than normal. A reasonable assumption from this study is that arteries with higher than normal compliance (e.g. soft plaque rupture in young arteries, perhaps myocardial infarction in arteries with positive remodelling) may be more vulnerable to longitudinal stent deformation and may need to be postdilated more carefully.Stent compression was not uniform around the ‘rim’ of the stent, because a single point of force was applied. The maximal compression was measured, as only the shortest length of stent visible would provide radial support to an artery. These observations suggest that significant stent deformation may occur before it is fluoroscopically evident. Moreover, the amount of compression likely to be determined fluoroscopically is deemed minimal compression, since the longest length of stent visible would be measured, which may even be larger than the original stent length (Figure 5).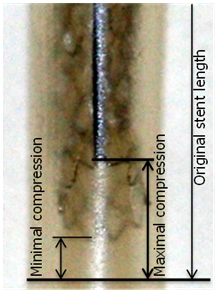 | Figure 5. Eccentric deformation characteristic of compression under a single point of force. There is a clear difference between the maximal and minimal compression |
Comparison of this study to previous bench data is complicated. The severely malapposed stent compression results in this study were principally gathered to contrast against the study's apposed models’ results. Outcomes for malapposed stents do not closely mirror previously published results, but it should be noted that the testing methodologies differ. Rather than compressing the stent by pressing on the whole rim of the stent, we applied a single point of force which, we believe, better replicates catheters pushing on a single strut or IVUS catheter catching. Another difference was that stents were supported along the entire bottom strut in previous benchtop studies, compared to only two points in the severe malapposition model, where the stent encountered the needle. This prevented it from the migration that would otherwise follow for severely malapposed stents. Apposed stents did not require a needle to prevent migration since they were already firmly in contact with the tubes.The issue of whether to express the compression of a stent as a percentage of length or as an absolute displacement (mm), was briefly examined. Different lengths (18mm, 23mm, and 28mm) of ML Vision stents, all with the same nominal diameter of 3mm, were tested. It was found that for the same applied load, longer stents deformed more at the same applied load. Therefore, expressing deformation as a percentage of length gave more reproducible information and thus was employed. This concept of longer stents deforming more was not a specific aim of this study and would be an interesting future study with clinical implications.
5. Limitations
Sample size was small at 3 stents of each design per apposition group. However, in this study, a total of 60 stents were required in the final testing phase, and a further 10 were used in the initial design phase. Similarly, one could argue that an important addition to this study would be to apply force to the middle section of a stent and to consider testing in a curved tube in addition to a straight one. This is an area for future work as stent usage becomes a rate limiting factor; especially when all possible investigational variables are included in the study design. For repeatability, arterial compliance, surface properties, diameter and thickness were assumed uniform, and the material differences between arterial layers (17) have been ignored. The use of a silastic tube is a further simplification. Silastic tubes may have different frictional and embedding properties to diseased arteries which could change the degree of deformation. Nevertheless, the basic premise that apposition is protective and differing compliance can alter that protection is reasonably investigated by these testing methods.
6. Conclusions
The results of this study have significant clinical implications. All stents are deformable if enough force is applied, particularly when unsupported (severe malapposition). Under these circumstances, the Element is approximately twice as deformable as the other tested stents. If compressed by a single point of force (e.g. post dilation balloon) considerable deformation can occur before it becomes fluoroscopically evident.Apposition makes a dramatic difference and became the major determinant of longitudinal strength with stents being up to 10 times more resistant to deformation when well apposed. A well apposed Element stent became more resist to deformation than any poorly apposed stents, regardless of its intrinsic strength. Arterial compliance has an effect, with more compliant arteries providing less resistive force to counter longitudinal compression. Stents deployed in very compliant arteries may be more vulnerable to this phenomenon.When selecting a stent, in addition to established important features (deliverability, radial strength, visibility and conformability) operators may also need to consider stent intrinsic strength and risk of initial malapposition. Importantly, appropriate postdilation or other strategies ensuring correct apposition will counteract longitudinal stent deformation.In future, stent longitudinal deformation in tortuous vessels may be investigated, since this clinical situation presents an increased opportunity for catheters or other devices snagging on stents. Further elaborations on this work may include modeling the effect of varying other arterial variables such as friction or hardness on stent longitudinal deformation.
ACKNOWLEDGEMENTS
The authors would like to thank the contributions of all the manufacturers in terms of the provision of stents, cooperation and feedback.
Note
1. MINITAB® and all other trademarks and logos for the Company's products and services are the exclusive property of Minitab Inc. All other marks referenced remain the property of their respective owners.
References
[1] | Williams PD, Mamas MA, Morgan KP, El-Omar M, Clarke B, Bainbridge A, et al. Longitudinal stent deformation: a retrospective analysis of frequency and mechanisms. EuroIntervention. 2011. Epub Nov. 4. |
[2] | Abdel-Wahab M, Sulimov DS, Kassner G, Geist V, Toelg R, Richardt G. Longitudinal Deformation of Contemporary Coronary Stents: An Integrated Analysis of Clinical Experience and Observations from the Bench. Journal of Interventional Cardiology. 2012;25(6):576-85. |
[3] | Janakiraman E, Subban V, Victor SM, Mullasari AS. Longitudinal deformation – Price we pay for better deliverability of coronary stent platforms. Indian Heart Journal. 2012;64(5):518-20. |
[4] | Hanratty CG, Walsh SJ. Longitudinal compression: a "new" complication with modern coronary stent platforms - time to think beyond deliverability? EuroIntervention. 2011;7(7):872-7. |
[5] | Pitney M, Pitney K, Jepson N, Friedman D, Nguyen-Dang T, Matthews J, et al. Major stent deformation / pseudofracture of 7 Crown Endeavor / Micro Driver stent platform: incidence and causative factors. EuroIntervention. 2011;7(2):256-62. |
[6] | Ormiston JA, Webber B, Webster MWI. Stent Longitudinal Integrity: Bench Insights Into a Clinical Problem. JACC. 2011;4:1310-7. |
[7] | Prabhu S, Schikorr T, Mahmoud T, Jacobs J, Potgieter A, Simonton C. Engineering assessment of the longitudinal compression behaviour of contemporary coronary stents. EuroIntervention. 2011. Epub November 2011. |
[8] | Mamas MA, Williams PD. Longitudinal stent deformation: insights on mechanisms, treatments and outcomes from the Food and Drug Administration Manufacturer and User Facility Device Experience database. EuroIntervention. 2012;7:1-9. Epub March 2012. |
[9] | Alfonso F, Macaya C, Goicolea J, Hernandez R, Segovia J, Zamorano J, et al. Determinants of coronary compliance in patients with coronary artery disease: an intravascular ultrasound study. JACC. 1994;23(4):879-84. |
[10] | Cameron JD, Jennings GL, Dart AM. The relationship between arterial compliance, age, blood pressure and serum lipid levels. J Hypertens. 1995;13:1718-23. |
[11] | Jeremias A, Spies C, Herity NA, Pomerantsev E, Yock PG, Fitzgerald PJ, et al. Coronary artery compliance and adaptive vessel remodelling in patients with stable and unstable coronary artery disease. Heart. 2000;84(3):314-9. |
[12] | Mangoni AA, Giannattasio C, Brunani A, Failla M, Colombo M, Bolla G, et al. Radial Artery Compliance in Young, Obese, Normotensive Subjects. Hypertension. 1995;26(6):984-8. |
[13] | Shaw JA, Kingwell BA, Walton AS, Cameron JD. Determinants of Coronary Artery Compliance in Subjects With and Without Angiographic Coronary Artery Disease. JACC. 2002;39(10):1637–43. |
[14] | van Andel CJ, Pistecky PV, Borst C. Mechanical properties of porcine and human arteries: implications for coronary anastomotic connectors. Ann Thorac Surg. 2003;76(1):58-64. |
[15] | Tai NR, Salacinski HJ, Edwards A, Hamilton G, Seifalian AM. Compliance properties of conduits used in vascular reconstruction. Br J Surg. 2000;87(11):1516-24. |
[16] | Numao T, Ogawa K, Fujinuma H, Furuya N.[Pulsatile diameter change of coronary artery lumen estimated by intravascular ultrasound]. Journal of cardiology. 1997;30(1):1-8. Epub 1997/07/01. |
[17] | Azarnoush H, Lamouche G, Bisaillon C-E, Degrandpre C, Boulet B. Evaluation of a Technique to Estimate the Compliance of Atherosclerotic Intima In: Bello F, Cotin S, editors.: Springer Berlin / Heidelberg; 2010. p. 20-8. |