Nasiruddin1, Md. Kamruzzaman2, Md. Razu Ahmed1, Md. Anwaruzzaman2, Md. Lawshan Habib2, Md. Abdul Mannan3
1Department of Chemistry, Bangabandhu Sheikh Mujibur Rahman Science & Technology University, Bangladesh
2Department of Applied Chemistry & Chemical Engineering, Bangabandhu Sheikh Mujibur Rahman Science & Technology University, Bangladesh
3Department of Chemistry, University of Rajshahi, Rajshahi, Bangladesh
Correspondence to: Md. Abdul Mannan, Department of Chemistry, University of Rajshahi, Rajshahi, Bangladesh.
Email: |  |
Copyright © 2018 The Author(s). Published by Scientific & Academic Publishing.
This work is licensed under the Creative Commons Attribution International License (CC BY).
http://creativecommons.org/licenses/by/4.0/

Abstract
A simple, sensitive, and selective spectrofluorimetric method has been presented to determine bovine serum albumin (BSA) levels using enoxacin (ENX)-aluminium (Al3+) as a fluorescence probe. The fluorescence (FL) intensity of ENX was enhanced when Al3+ was added in to the ENX solution through charge transfer reaction and formed a binary ENX-Al3+ complex. The FL intensity of the ENX-Al3+ complex was further enhanced in the presence of BSA in Briton-Robinson buffer (pH=5.8). Under the optimum condition, the FL intensity was enhanced linearly by BSA concentrations in the range of 0.13 to 10.0 ng mL–1. The limit of detection was found to be 0.03 ng mL–1 with the relative standard deviation of 1.23% for 5 replicate determinations of 25 ng mL–1 of BSA. In comparison with most of the other reported methods, the proposed FL method provides high sensitivity, wide linear range with simple and less expensive instruments to determine BSA.
Keywords:
Fluorescence probe, Enoxacin, Aluminium (III), Bovine serum albumin, Charges transfer reaction, High sensitivity
Cite this paper: Nasiruddin, Md. Kamruzzaman, Md. Razu Ahmed, Md. Anwaruzzaman, Md. Lawshan Habib, Md. Abdul Mannan, Spectrofluorimetric Determination of Bovine Serum Albumin Using Enoxacin-Aluminium (III) as a Fluorescence Probe, American Journal of Biochemistry, Vol. 8 No. 5, 2018, pp. 100-105. doi: 10.5923/j.ajb.20180805.03.
1. Introduction
Bovine serum albumin (BSA) is a serum albumin protein which has abundant biological applications including ELISAs (Enzyme-Linked Immunosorbent Assay), immunoblots and immunohistochemistry. It is used as a nutrient in cell and microbial culture and to stabilize some enzymes during digestion of DNA and to prevent adhesion of the enzyme to reaction tubes and other vessels. BSA is also involved in the transport of a variety of endogenous and exogenous substances of the body that plays a vital role in the distribution and deposition of these substances [1]. The interaction between proteins and various kinds of drugs is very important for wide range of pharmacological, biological, and clinical applications. Because, when pharmaceutical compounds are absorbed, they enter into the circulatory system and widely and reversibly bind to serum albumin [2]. Therefore, it is becoming more and more important to analyze proteins qualitatively and quantitatively for the investigation of proteins and their interaction with drugs. The widely used chemical assays to determine protein levels are Lowry [2, 3], silver staining method [4], fluorescence resonance light scattering (RLS) [5-7], UV–vis absorption spectroscopy [8], Fourier transform infrared (FTIR) [9], circular dichroism spectroscopy (CD) [10,11], chemiluminescence [12, 13], electrochemistry [14, 15], and nuclear magnetic resonance (NMR) [16]. However, these methods have some limitations such as narrow linear range or slower reaction time, limited practical applications, complicated as multiple steps are involved and expensive instrumentation.In order to overcome the above limitations, ligand sensitized FL methods using covalent and non-covalent FL probes have been extensively examined to determine BSA because FL method provides high sensitivity and selectivity with simple and inexpensive instrumentation [17-22]. Covalent FL probes have many problems arising from inadequate chemistry, multiple derivatives and reaction conditions although they are suitable for labeling the N-terminus or other functional groups of the protein. In contrast, non-covalent FL probes are simple, fast, and less expensive which are frequently used to determine protein [23, 24]. In the presented FL method, enoxacin-Al3+ has been utilized as a FL probe for the investigation and detection of BSA levels. Enoxacin (ENX) is a fluoroquinolone antibacterial drug containing active group comprised of a –COOH, C=O, and a suitable ligand, and forms stable complexes with metal ions due to efficient energy transfer from the ligand to the metal. In this work, we selected ENX as a ligand for the Al3+ ion and investigated the enhancement of FL intensity by ENX and BSA. We found that the FL intensity of ENX was enhanced when Al3+ ion was added to ENX solution by charge transfer, and that this intensity was markedly enhanced when BSA was present in the ENX-Al3+ solution. Furthermore, FL intensities were enhanced by BSA over a wide concentration range in a linear manner with a limit of detection at the nanogram level. Based on the above, a novel FL method of high sensitivity and selectivity is proposed to determine BSA levels in human serum samples. In addition, interactions in the FL system are discussed.
2. Experimental
Reagents and chemicals: All reagents were of analytical reagent grade and were used without further purification. Distilled deionised (DI) water (Millipore, Milli Q Water System, USA) was used throughout. BSA and ENX were purchased from Sigma-Aldrich (St. Louis, USA) and used without further purification. Stock standard solution of BSA (0.5 mM) was prepared by dissolving BSA in DI water and stored at 4°C. Stock solution of ENX (1 mM) was prepared in DI water and stored in refrigerator before use. A stock solution of AlCl3 (5 mM) purchased from Duksan Pure Chemical Co. Ltd. (South Korea) was prepared using DI water. Britton-Robinson buffer (0.1 M) solution of pH=5.8 was used throughout and working solutions were prepared by diluting the stock solutions appropriately immediately before use.Apparatus: All the fluorescence spectra were recorded using a spectrofluorimeter (Model F- 4500, Hitachi, Japan) equipped with a 450-W Xenon lamp (Model XBO 450 W/1, Osram, Germany) as excitation light source and a photomultiplier tube (Model R928, Hamamatsu, Japan) powered at 950V as the detector. The excitation and emission slits were set at 10 nm to measure all the FL spectra. pH values were adjusted using a pH meter (Model Orion, 520A, USA). All the UV-visible spectra were measured using a UV-visible spectrophotometer (UV-1800, Shimadzu, Japan). Experimental procedure: All FL measurements were made using the following procedure. To a 10 mL volumetric flask, 1 mL of Al3+ solution, 1 mL buffer (pH=5.8) solution, and 1 mL ENX solution were added and diluted with 5 mL of doubly distilled DI water. The solution was mixed thoroughly and allowed to stand for several minutes. Then, 1 mL of BSA was added to the solution and diluted to mark with distilled DI water. The mixture was then allowed to stand for about 20 minutes. The solution was then added to 1 cm quartz cells to measure FL spectra using an emission and excitation wavelengths of 409 nm and 274 nm, respectively. Excitation and emission slits were set at 5 nm and the photomultiplier tube voltage was set at 900 V.
3. Results and Discussion
Fluorescence spectral characteristicsThe FL emission spectra of (a) Al3+, (b) BSA, (c) ENX, (d) ENX-Al3+, and (e) ENX-Al3+-BSA are shown in Figure 1. Al3+ had nearly no emission peak whereas ENX exhibited emission at ~409 nm [Figure 1, curve (a, c)]. When Al3+ was added to ENX solution, a charge transfer reaction occurred, which enhanced the FL intensity of ENX and red shifted to 428 nm [Figure 1, curve (d)]. These results suggest that ENX forms a binary complex with Al3+ and this complex enhances the FL intensity of ENX [25]. Furthermore, the FL intensity of the ENX-Al3+ system was further enhanced markedly with a slight red shift (431 nm) by the addition of BSA [Fig. 1, curve (e)], presumably due to an intramolecular energy transfer from BSA to ENX-Al3+ [25]. Thus, the results imply an interaction between BSA and ENX-Al3+ to form a stable ENX-Al3+-BSA complex. 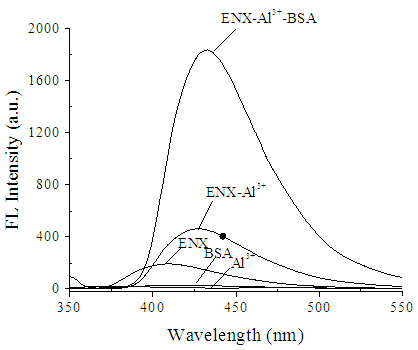 | Figure 1. Fluorescence emission spectra: a) Al3+; b) BSA; c) ENX; d) ENX-Al3+; e) ENX-Al3+-BSA. Conditions: [BSA] = 5 ng mL–1; [ENX] = 5 µM; [Al3+] = 0.06 mM; pH = 5.8; Briton-Robinson buffer=0.1 M; λex = 274 nm |
In order to investigate the interaction between ENX, Al3+, and BSA, the absorption spectra of Al3+, BSA, ENX, ENX-Al3+, and ENX-Al3+-BSA were recorded. The spectra are shown in Figure 2. ENX exhibited maximum absorbance at about 274 nm [Figure 2, curve (3)]. When Al3+ was added to ENX solution, the absorbency of ENX was enhanced and a blue shift was occurred to 261 nm [Figure 2, curve (4)], which suggested that ENX formed a binary complex with Al3+ via charge transfer. After the addition of BSA to the ENX-Al3+ system, absorbency was further enhanced and slightly blue shifted from 261 to 257 nm [Figure 2, curve (5)]. The above results indicate that BSA reacted with ENX-Al3+ to form an ENX-Al3+-BSA ternary complex. Furthermore, this resulted in an increase in FL intensity of several fold (Figure 1), and intensities were found to be linearly correlated with BSA concentration.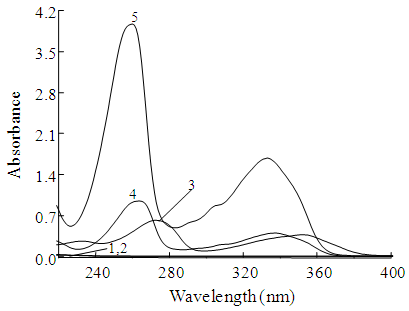 | Figure 2. Absorption of BSA in different system: 1) Al3+, 2) BSA, 3) ENX, 4) ENX-Al3+, 5) ENX-Al3+-BSA. Conditions: [BSA] = 25 ng mL–1; [ENX] = 5µM; [Al3+] = 0.06 mM; pH = 5.8; Briton-Robinson buffer = 0.1 M |
Energy transfer from BSA to ENXThe intramolecular energy transfer from BSA to ENX was examined using Föster’s non-radioactive energy transfer theory [26]. The energy transfer depends upon the extent of overlap between the emission spectrum of the donor with the absorption spectrum of the acceptor, the relative orientation of the donor-acceptor transition dipoles, and the distance between donor and acceptor. The overlap between the FL emission spectrum of BSA (donor) and the absorption spectrum of ENX (acceptor) is shown in Figure 3. The figure shows a large overlap between the FL spectrum of BSA and the absorption spectrum of ENX. Moreover, the maximum donor-acceptor distance was found to be less than 7. The result implies that ENX forms a ground complex with BSA by non-radioactive energy transfer from ENX to BSA. It can be concluded that ENX transfers energy to Al3+ and form an ENX-Al3+ complex exhibiting an enhanced FL peak at 428 nm. ENX can also transfer energy to BSA, and thus, BSA forms a stable ternary complex with the ENX-Al3+ system and enhances FL intensities.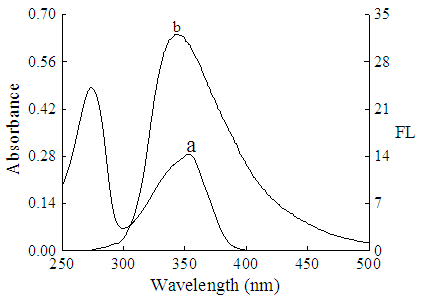 | Figure 3. The overlap of the fluorescence spectra of BSA (b) and absorption spectra of ENX (a) |
Effect of pH and buffer solutionThe pH of a solution plays an important role to enhance FL intensity. Thus, the effect of pH on the FL intensity of the ENX-Al3+-BSA system was investigated in the range of 4.5 to 6.5, and maximum FL intensity was obtained at pH 5.8 and thus, this value was selected for the presented experiment. The effect of pH on FL intensity is shown in Figure 4. At high pH values, Al3+ hydration results in the formation of soluble hydroxo complexes, polycations, and hydroxo polymers, which interfere with chelation between Al3+ and ENX [25]. Buffer solutions also had a substantial effect on FL intensity, and thus, we examined the effects of the following buffers: KH2PO4-Na2HPO4, borax-HCl, NH4Cl-NH3, Britton-Robinson, KH2PO4–NaOH, and tris-HCl. Britton-Robinson buffer at 0.1M exhibited maximum FL intensity, and thus, was used in this experiment.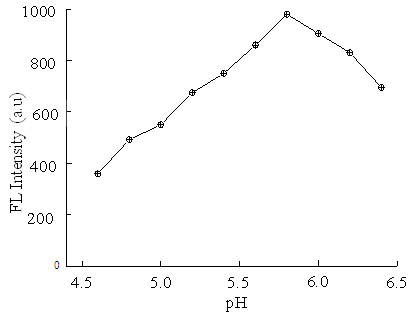 | Figure 4. Effect of pH on the FL intensity of ENX-Al3+-BSA system. Conditions: [BSA] = 5 ng mL–1; [ENX] = 5µM; [Al3+] = 0.06 mM; Briton-Robinson buffer = 0.1 M |
Effect of Al3+ ion concentrationThe effect of Al3+ ion concentration on FL intensity was examined in the range of 0.01–0.12 mM. The effect is shown in Figure 5. The FL intensity was found to increase with Al3+ concentration up to 0.06 mM, and thus, this concentration was used to obtain maximum intensity.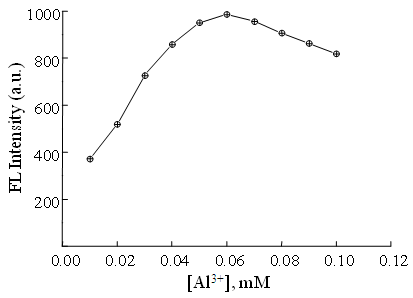 | Figure 5. Effect of Al3+ concentration on the FL intensity of ENX-Al3+-BSA system. Conditions: [BSA] = 5 ng mL–1; [ENX] = 5µM; pH = 5.8; Briton-Robinson buffer = 0.1 M |
Effect of ENX concentrationENX solution might also influence the sensitivity of the presented method. Thus, the effect of ENX concentration on FL intensity was investigated as shown in Figure 6. Maximum FL intensity was obtained at an ENX concentration of 5 µM. At concentrations above this level, intensities declined due to maximum complex formation of Al3+ with ENX at this concentration leads to fluorescence quenching due to electron transfer [25]. Thus, 5 µM ENX was chosen for this study. 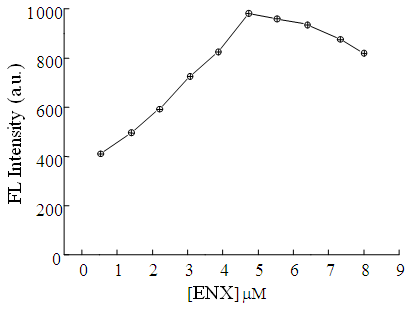 | Figure 6. Effect of ENX concentration on the FL intensity of ENX-Al3+-BSA system. Conditions: [BSA] = 5 ng mL–1; [Al3+] = 0.06 mM; pH = 5.8; Briton-Robinson buffer = 0.1 M |
Effect of the addition order of reagentsThe addition order of reagents also influenced the FL intensity of the system. A series of solutions with different addition orders of reagents was investigated and Al3+, Britton-Robinson buffer, ENX, BSA order of addition produced maximum FL intensities. The chelation reaction of this ENX-Al3+-BSA system took ~20 min. at room temperature. This meant that in practice FL intensity reached a maximum at 20 min. after all reagents had been added and then remained stable for at least 2h.Effect of interfering substancesIn order to investigate the selectivity of the proposed method, the effects of species known to coexist with BSA on FL intensity were examined. Accordingly, a set of solutions of these species was prepared at different concentrations in 3.5 ng mL–1 BSA. A foreign species was considered an interfering component if it produced a BSA quantitation error of > ±5%. Results are summarized in Table 1. However, none of the substances examined produce an error of this magnitude.Table 1. Tolerance limit of co-existing substances on the determination of BSA 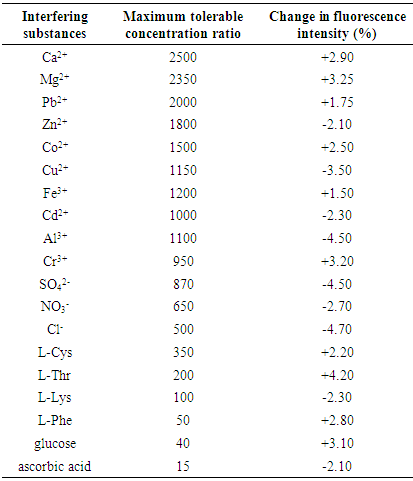 |
| |
|
4. Analytical Application
Linear range and detection limit to determine BSAUsing the above-mentioned optimal conditions, a calibration curve of FL intensity vs. BSA concentration in the range of 0.13 to 10.0 ng mL–1 (the correlation coefficient (r) between the two variables was 0.9981) was plotted. The limit of detection (LOD) as defined as recommended by IUPAC, LOD=3Sb/m (where Sb is the standard deviation of blank signals and m is the slope of the calibration curve) was found to be 0.03 ng mL–1 for BSA. The standard deviation (RSD) of the proposed method was 1.15% for 5 measurements at a BSA concentration of 5.0 ng mL–1. Determination of BSA in serum sampleIn order to demonstrate the applicability of the proposed method, it was applied to determine BSA concentrations in serum sample. The results are listed in Table 2. Fresh serum samples were diluted appropriately (10 fold) to obtain a BSA concentration within the linear range. Aliquots (1.0 mL) of sample solutions were analyzed using the developed method according to the standard addition method. The results in Table 2 indicated that the developed method is reliable and suitable for practical applications with good precision and accuracy. Recoveries were found to be in the range 94.0–107.0% for BSA.Table 2. Determination of BSA in samples of serum 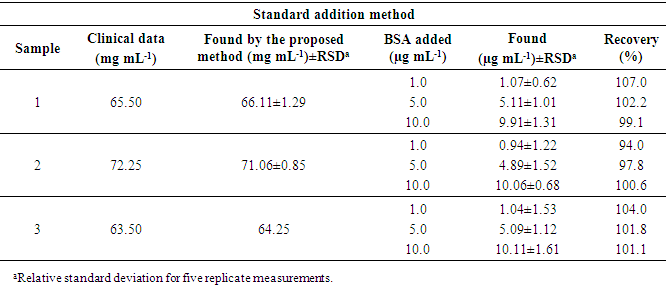 |
| |
|
5. Conclusions
A sensitive, straightforward, cost effective spectrofluorometric method was described based on the interaction between BSA and ENX-Al3+ complex for the determination of BSA levels in serum. It was found that ENX can form complexes with Al3+ and an energy transfer occurred from ENX to Al3+, which produced a strong FL signal. After the addition of BSA, it can combine with the Al3+-ENX binary complex and form the Al3+-ENX-BSA ternary complex. ENX can transfer the energy to BSA and the FL intensity of Al3+-ENX complex was markedly inhibited after the addition of BSA in a linear manner in the BSA concentration ranged from 0.13 to 10.0 ng mL–1. In particular, the proposed method offers a lower limit of detection (0.03 ng mL–1) than previously reported methods, and was successfully applied to the determination of BSA in serum samples revealing excellent reproducibility and accuracy. Furthermore, the described method offers the advantages of simplicity, rapidity, sensitivity, and selectivity for the determination of BSA.
ACKNOWLEDGEMENTS
This research was supported by Bangabandhu Sheikh Mujibur Rahman Science & Technology University Research Fund.
References
[1] | Kamat BP, Seetharamappa J. In vitro study on the interaction of mechanism of tricyclic compounds with bovine serum albumin. J Pharm Biomed Anal. 2008; 35: 655–664. |
[2] | Lowry OH, Rosebrough NJ, Farr AL Randall RJ. Protein measurement with the folin phenol reagent. J Biol Chem. 1951; 193: 265–275. |
[3] | Bradford MM. A rapid and sensitive method for the quantitation of microgram quantities of protein utilizing the principle of protein-dye binding. Anal Biochem. 1976; 72: 248–254. |
[4] | Ohsawa K, Ebata N. Silver stain for detecting 10-femtogram quantities of protein after polyacrylamide gel electrophoresis. Anal Biochem. 1983; 135: 409–415. |
[5] | Guo C, Wu X, Xu W, Yang J. Resonance light-scattering enhancement effect of the protein-Y3+-TTA-SLS system and its analytical application. Luminescence. 2008; 23: 404–409. |
[6] | Liu R, Yang J, Sun C, Wu X, Li L, Li Z. Resonance light-scattering method for the determination of BSA and HSA with sodium dodecyl benzene sulfonate or sodium lauryl sulfate. Anal Bioanal Chem. 2003; 377: 375–379. |
[7] | Chakraborty B, Basu S. Interaction of BSA with proflavin: A spectroscopic approach. J Luminesc. 2009; 129: 34 –39. |
[8] | Kathiravan A, Chandramohan M, Renganathan R, Sekar S. Spectroscopic studies on the interaction between phycocyanin and bovine serum albumin. J Mol Struct. 2009; 919: 210–214. |
[9] | Ferrer EG, Bosch A, Yantorno O, Baran EJ. A spectroscopy approach for the study of the interactions of bioactive vanadium species with bovine serum albumin. Bioorg Med Chem. 2008; 16: 3878–3886. |
[10] | Zhang YZ, Zhou B, Zhang XP, Huang P, Li CH, Liu Y. Interaction of malachite green with bovine serum albumin: determination of the binding mechanism and binding site by spectroscopic methods. J Hazard Mater. 2009; 163: 1345–1352. |
[11] | Ashoka S, Seetharamappa J, Kandagal PB, Shaikh SMT. Investigation of the interaction between trazodone hydrochloride and bovine serum albumin. J Luminesc. 2006; 121: 179–186. |
[12] | Hara T, Tsukagoshi K. Chemiluminescence analyses of biological constituents using metal-complex catalysts. Anal Sci. 1990; 6: 797–806. |
[13] | Yu X, Mou C. Determination of protein using copper(II)-Alizarin Red S complex as an efficient chemiluminescent probe. Anal Lett. 2011; 44: 137–145. |
[14] | Luo H, Du Y, Guo ZX. Electrochemistry of N-n-undecyl-N'-(sodium-p-aminobenzenesulfonate) thiourea and its interaction with bovine serum albumin. Bioelectrochem. 2009; 74: 232–235. |
[15] | Fotouhi L, Banafsheh S, Heravi MM. Electrochemistry of the interaction of furazolidone and bovine serum albumin. Bioelectrochem. 2009; 77: 26–30. |
[16] | Bojko B, Sułkowska A, Jurczyk MM, Równicka J, Sułkowski WW. Investigations of acetaminophen binding to bovine serum albumin in the presence of fatty acid: Fluorescence and 1H NMR studies. J Mol Struct. 2009; 924–926: 332–337. |
[17] | Guo XJ, Sun XD, Xu SK. Spectroscopic investigation of the interaction between riboflavin and bovine serum albumin. J Mol Struct. 2009; 931: 55–59. |
[18] | Anbazhagan V, Renganathan R. Study on the binding of 2,3-diazabicyclo[2.2.2]oct-2-ene with bovine serum albumin by fluorescence spectroscopy. J Luminesc. 2008; 128: 1454–1458. |
[19] | Wu X, Sun S, Guo C, Yang J, Sun C, Zhou C, Wu T. Resonance light scattering technique for the determination of proteins with Congo red and Triton X‐100. Luminescence. 2006; 21: 56–61. |
[20] | He L, Wang X, Liu B, Wang J, Sun Y, Gao E, Xu S. Study on the interaction between promethazine hydrochloride and bovine serum albumin by fluorescence spectroscopy. J Luminesc. 2011; 131: 285–290. |
[21] | Gong A, Zhu X, Hu Y, Yu S. A fluorescence spectroscopic study of the interaction between epristeride and bovin serum albumine and its analytical application. Talanta. 2007; 73: 668–673. |
[22] | Bani-Yaseen AD. Spectrofluorimetric study on the interaction between antimicrobial drug sulfamethazine and bovine serum albumin. J Luminesc. 2011; 131: 1042–1047. |
[23] | Jiang C, Luo L. Spectrofluorimetric determination of human serum albumin using a doxycycline–europium probe. Anal Chim Acta. 2004; 506: 171–175. |
[24] | Liu S, Yang J, Wu X, Wang F, Wang F, Jia Z, Ma L. Study on the interaction between protein and Eu(III)–chlorotetracycline complex and the determination of protein using the fluorimetric method. Luminescence. 2004; 19: 352–357. |
[25] | József G, Harangozóa, Zsombor M, László B, Véronique W, Cédric L. Effect of host-guest complex formation on the fluorescence of 6-methoxy-1- methyl-quinolinium cation with 4-sulfonatocalix [4] arene: Utilization as a fluorescent probe for the study of difenzoquat binding. J Incl Phenom Macrocycl Chem. 2015; 81: 377–384. |
[26] | Lakowicz JR. Principles of frequency-domain fluorescence spectroscopy and applications to cell membranes. Plenum Press, New York. (1983). |