Ukpabi C. F1, Akubugwo E. I2, Ibiam U. A3, Agbafor K. N3, Ezeigbo R. O4, Wogu C5
1Department of Biochemistry Abia State Polytechnic, Aba
2Department of Biochemistry Abia State University, Uturu
3Department of Biochemistry Ebonyi State University, Abakiliki
4Department of Biology Abia State Polytechnic, Aba
5Department of Chemistry Abia State Polytechnic, Aba
Correspondence to: Ukpabi C. F, Department of Biochemistry Abia State Polytechnic, Aba.
Email: |  |
Copyright © 2012 Scientific & Academic Publishing. All Rights Reserved.
Abstract
We investigated the effect of inorganic and organic fertilizer application in soil at different concentrations on the pollution index per metal and exposure time on the biochemical responses of the earthworm Eisenia fetisa (Savigny).This is to elucidate the mechanism of action of heavy metals and explore the potential for using these responses as biomarkers for monitoring contaminated soils. The elemental composition of the soil and fertilizer samples was analyzed using atomic absorption spectrophotometer. Molybdemum (Mo) levels (mg/kg) rated highest in the soil (1580.00± 1.20), inorganic fertilizer (2570.00±1.73) and animal manure (1080.00± 2.00) samples while vanadium (V), arsenic (As), mercury ( Hg), silver (Ag) and chromium (Cr; only in soil), were not detected by our analytical method. The pollution assessment of the metals followed the sequence: Cd (33.34) > Cr (9.84) > Mg (9.50) > Pb (4.31) > etc and Cr (28.88) >Mg (14.46)> Cd (9.74) > Cu (4.43) > etc in the inorganic and animal treated soils respectively. The biochemical responses of the earthworms (Eisenia fetida) exposed to 0.1 and 0.5 g/kg soil application rate for 28days indicated significant (P<0.05) level (IU/I) in all the parameters measured except albumin (ALB) which showed no significant reduction (p>0.05). AST, ALP and GST showed more sensibility with significant difference (p<0.05) in concentration than ALT, ACP and CAT respectively. These biochemical responses measured in E. fetida (Savigy) could be used for chemical testing in the laboratory or for soil contamination surveys.
Keywords:
Earthworms, Eisenia Fetida,Biomarkers, Heavy Metals, Inorganic and Organic Fertilizers
Cite this paper: Ukpabi C. F, Akubugwo E. I, Ibiam U. A, Agbafor K. N, Ezeigbo R. O, Wogu C, Fertilizer Application Effect on Heavy Metal Composition and Biochemical Responses of Earthworm Eisenia Fetida (Savigny) in a Sandy Farm Soil, American Journal of Biochemistry, Vol. 3 No. 3, 2013, pp. 74-79. doi: 10.5923/j.ajb.20130303.02.
1. Introduction
A major agricultural research priority is to sustain soil productivity and to develop better methods to monitor changes in soil physico-chemical properties as affected by management. Evaluation of the effects of contaminants in soils has become a priority, following the abuse, use and reuse of soil for food production. Molecular markers could be used as diagnostic and prognostic early-warning tests to detect and assess the effects of pollution, particularly low concentrations of complex mixture of contaminants[1].Soil assessment methods which only determine total metal concentrations are often not accurate in predicting soil organism toxicity[2]. The toxicity test available for soil fauna have been restricted, until now to growth and reproduction. Thus sublethel invertebrate tests are needed for soil pollution testing in the laboratoryBurrowing animals, soil bacteria, fungi and the roots of plants are the part of the biological processes of soil formation[3]. Earthworms are common in a wide range of soils and may represent 60-80% of the total soil biomass[1]. This makes them one of the most suitable bioindicator of soil organisms for testing pollutants in the soil. Soil pollutants both in acute and chronic exposure intereact with many different cellular components, thereby interfering with the normal metabolic functions causing cellular injuries and on extreme cases, death of soil organisms[4]. Toxicology and biochemical investigation have led to the identification of large number of potential real target components whose normal activities are inhibited, enhance or modified by heavy metal pollutants[5]. The poisoning of heavy metals may be summarized as the effects on enzymes and on cellular macromolecules via free radical formation.The term heavy metal has been called a “Misinterpretation” in an IUPAC technical report due to the contradictory definitions and its lack of coherent scientific basis (Wikipedia, 2013). Heavy metals apply to the group of metals which are toxic or poisonous even at low concentrations and have high atomic number, number of atomic weight and a specific gravity greater than 5.0[21.The possible effect of this definition may arrange toxic metals (Cd and Pb), trace elements (Fe and Zn) and/or exclude light metals (Mg and Bo) as classes of heavy metals.In Nigeria, mineral NPK fertilizers and animal manures are the main fertilizers used by farmers. For many years fertilizers have been regulated to ensure that the product label provides accurate information on essential plant nutrient content. In recent years, concerns about quality of some fertilizer products have extended beyond nutrient contents to the potential presence of non-nutrient toxic substances such as heavy metals. The current fertilizer application recommends 3-6 bags of NPK fertilizer application of nutrients (kg/ha), follows by 1-2 bags of urea[6]. The maximum application rate of this management system is about 0.225g/kg soil/yr for low fertility soil (where, 1 hectare = 2 x 106 kg/soil).Taking the maximum application rate as 50 kg/ha/yr with multiplication factor of 1.0 million. Informal survey indicates that fertilizers product is not tested for heavy metals thus resulting in heavy metal build up in soil and subsequent soil organism poisoning. Thus the aim of this work was to investigate the heavy metals incidence in the application or inorganic and organic fertilizers to farm soil and the biochemical responses of earthworm Eisenia Fetida (Savigny) exposed to the treated fertilizer soils
2. Materials and Methods
2.1. Materials
The inorganic fertilizer (N15P15K15 products of Federal and Chemical Company Limited Kaduna) was purchased in full bag from a market in Aba metropolis while the organic manure (composted pig manure) was collected from pig farm located in Aba North Local Government Area of Abia State, Nigeria. N15P15K15, and pig manure were denoted as CF and PM respectively. All chemicals and reagents used were from reliable sources and of high analytical grade. Heavy metal analyses were carried out using atomic absorption spectrophotometer (UNICAM 969, Japan) whiles the biochemical parameters were determined using spectrophotometer (model 390, Turner®, USA).
2.2. Soil Sampling
Soil samples were collected from the different locations of the Abia State Polytechnic botanical garden Aba Abia State, Nigeria lying between latitude 4 .451 and longitude 30 and 80 91 East. The soil samples were collected by sterile method using plastic auger from a depth of 0-15cm[7]. The auger was washed with water and 70% ethanol before use.
2.3. Experimental Design
The experiment included three treatments with three replication arrangements. The treatments were (a) no application of fertilizer (NF); (b) mineral NPK fertilizer application (CF); and (c) Pig manure application (PM).Each treatment had 0.0, 0.10 and 0.5g/kg soil fertilizer application rates. Fertilizers were fully mixed with soil on a plastic sheet and loaded into various sets of the experimental pots and were allowed for a latent period of 7 days. The moisture content of the soil was adjusted daily with 5-10ml distilled water to compensate for the lost water. The experimental pots were perforated with numerous pin-size holes at the bottom. The adoption of this mild leaching method was done to reflect the true environmental solution. The sets of earthworms were exposed to the various fertilizer application rates and allowed for a period of 28 days in the laboratory.
2.4. Soil and Fertilizer Analyses
The soil and fertilizer samples were anlaysed using the method of the American Standard Testing Material[8] as described in[6]. The samples (2.0g) were weighted into a beaker and digested with 20ml of conc. HNO3 and 10ml of conc. HClO4 on a hot plate with gentle boiling. The digested samples were evaporated to dryness and the residue mixed with 10ml of 2.0 M HCl, filtered into a 100ml standard flask using whatman No1 filter paper. Several digested samples were analyzed for the heavy metal of interest – Mn, B, Cu, Fe, Mo, Ni, Zn, Mg, V, As, Cd, Hg, Pb, Co, Cr and Ag using atomic absorption spectrophotometer (Unicam 969,Japan). The samples were analyzed in triplicates. The effectiveness of the method used was tested by using spiked samples that were later used as reference samples. Soil particle fractionation and moisture content were carried out according to the methods of[8]. The pH was determined using glass electrode pH meter at the ratio of 1:2 sample / water suspensions[9].
2.5. Biochemical Assay
The biochemical parameters were done on individual earthworm at a dilution ratio of 1:2w/v using Tris buffer. Each earthworm was rinsed with distilled and deionized water (< 20 seconds) and was cut and crushed using a laboratory mortar. The resulting crushed tissues were centrifuged at 7500r/min for 15min and the supernatant was removed and stored in a refrigerator at 4oC and biochemical assays, done within 7 days[9].Coelomic fluids were tested for aspartateaminotransferase (AST, EC.2.6.1.1), and alanine aminotransferase (ALT, EC.2.6.1.2), according to[10]. This method is based on the action of the aminotransferases on their substrates to liberate their corresponding amino acids, which react with 2.4 dinitrophenylhydrazine (DNPH) to form coloured complex. Consequently, enzymes activities are measured in relation to the concentration of the colour solutions. Each clarified sample (0.1 ml) was added into a clean test-tube containing 1ml of the corresponding substrate. The mixture was incubated at 37℃ in a waterbath for 15mins and then 0.5ml of DNPH and 0.5ml 0.4N sodium hydroxide (NaOH) were added. The absorbance of the samples and the standard (sodium pyruvate) were read in the spectrophotometer against the reagent blank. The alkaline phosphatase (ALP, EC.3.1.3.1) and acid phosphatase (ACP, EC.3.1.3.2) were estimated according to[11]. This is based on the action of the phosphatases on p-nitrophenylphosphate to liberate p-nitrophenol and organic phosphate, which at acid/alkaline pH values changes colour that can be spectrophotometrically determined. Each clarified sample (0.1ml) was added a clean test tube containing 1ml of corresponding substrate. The mixture was incubated at 37℃ in a water bath for 15mins and then 0.8ml of 0.5N NaOH, 1.2ml of 0.5N sodiumhydrogentrioxocarbonate(IV) (NaHCO3), 1ml of 4-amino-1 phenyl-2,3 methyl-5-pirazolon, and 1ml of potassium ferricyanide were added. The absorbance of the yellow coloured solution produced and the standard (n-nitrophenyl solution) were read in the spectrophotometer against the reagent blank. Catalase (CAT, EC.1.11.1.6) was measured using the Northwest life science specialties kit USA (NWLSSTM). Catalase reduces the hydrogen peroxide which can induce damage in the membrane by initiating lipid peroxidation[1]. A portion of the supernatant (0.1ml) was added to cuvette containing 1.0ml of assay buffer, 1.0ml of sample dilution buffer and 1.0ml of H2O2 regent. The absorbance of the solution was read immediately in a spectrophotometer. The rate of disappearance of H2O2 was followed by observing the rate of decrease in the absorbance and latalase activity calculated.Glutathione-S-transferase (GST, EC.2.5.1.18) were measured by the method of[1]. A portion of the supernatant (0.1M) was added to curvette containing 2.75ml of sodium phosphate buffer (0.1m pH 7.0), 0.10ml reduced glutathione (1.0mM) and 0.05nm 1-chloro-2,4-dinitrobenzene making the total volume 3.0ml. The mixture was quickly mixed and the absorbance read at 340nm. The blank underwent the same treatment as the sample except that distilled water was used in place of sample. The values obtained were subtracted from the blank to give the catalytic activity of GST.Coelomic fluid albumin concentration was determined using bromocresol green method as described by[12]. The samples and indicator (bromocresol green) were pipette into labeled test tubes as indicated in the procedure adapted from[12]. When the indicator binds to albumin, the colour changes and the absorbance of the colour produced was measured using spectrophotometer.
3. Environmental Impact Assessment
The pollution index per metal with a significant quantity considered from pi> 1 was used as assess the effect of the control soil as described by[13]. Pi is a measure of a metal concentration in fertilizer treated soil samples and its concentration in a background sample. Statistical analysis is based on the following mathematical relations.Pi = Cm/CbWhere Cm is the concentration of the metal obtained in the fertilizer – treated soils and Cb is the relative background of concentration of the metal. Pollution range was classified into intervals that define a slight (1.1-2.0), moderate (2.1-4.0), several (4.1-8.0) very severe (8.1-16.0) and excessive pollution (>16.0) respectively.
4. Results and Discussion
Textural analysis showed a preponderance of sand fraction (76.00%) followed by clay (16.00%), then silt (8.00%) thus classifying the soil as sandy clay as presented in Table 1. Although sandy soils are known to have a poor retention capacity for water and metals, the relatively moderate proportion of clay suggests that the soil will not drain easily with implications for potential deleterious impact of retained pollutants on environmental receptors. The acidic pH 5.90±0.05 recorded for the soil is within the range for agriculture soil[14]. Soil pH plays a major function in the sorption of heavy metals as it controls the solubility and hydrolysis of metal hydroxides. The soil also showed moderate organic matter content of 1.75± 0.02%.Table 1. Physico-Chemical Characteristics of the Farm 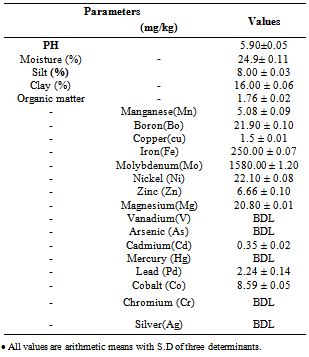 |
| |
|
Elemental composition of the farm soil used for the study is shown in table 1. The result revealed Mo (1580.00±1.20mg/kg) as the highest content while V, As, Hg, Cr, and Ag were not detected by our analytical method. The farm soils showed heavy metal contents below the ranges of threshold values reported in literature for agricultural soils[15] establishing the suitability of the soils for planting. The elemental composition of the fertilizers is show in Table 2. Mo levels were the highest whereas V, As, Hg and Ag were not detected by our analytical method. Heavy metals detected were magnesium (Mg), Iron (Fe), nickel (Ni), zinc (Zn). Cadmium (Cd), manganese (Mn), copper (Cu), cobalt (Co). lead (Pb). Chromium (Cr), and boron (B). Pb (7.43±0.80mg/kg) and Cd (11.32±1.12mg/kg) were detected in CF while only Cd (3.06 ±0.90mg/kg) was detected in PM. The concentrations of these heavy metals in the CF sample were higher than those in the PM sample except Fe and Mn.The amount of heavy metals added to soil was estimated by calculating the pollution index per metal of the various fertilizers. This parameter is a good tool for accessing the heavy metal loading of the soil. The summary of the pollution index assessment of the metal content revealed that metal levels were generally higher in the CF treated soil than in the PM treated soil. Cd, Pb and Cu pollution indexes were significant higher in CF treated soil than the PM treated soil. The pollution assessment of the metals followed the sequence: Cd (33.34) > Cr (9.84) > Mg (9.50) > Pb (4.31) > etc and Cr (28.88) >Mg (14.46)> Cd (9.74) > Cu (4.43) > etc in the CF and PM treated soils respectively.Table 2. Heavy metal contents and pH values of the Inorganic and Organic Fertilizers used in the Study 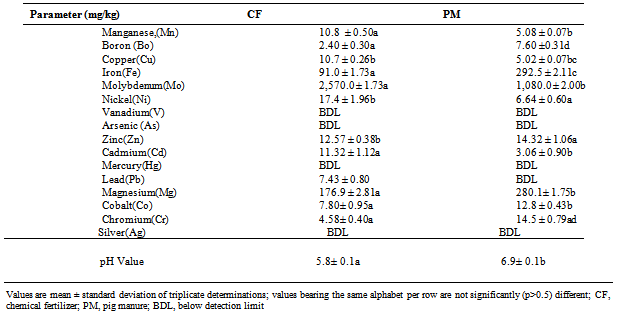 |
| |
|
Table 3. Biochemical Responses of Earthworm Exposed to the Various Fertilizer Treated Soils 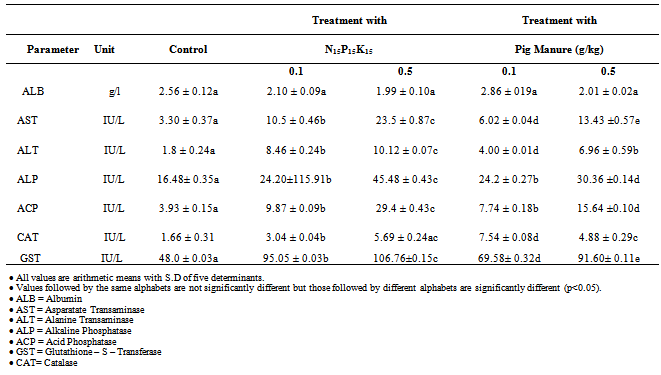 |
| |
|
Table 4. Summary of Pollution Indexes Assessment of the two Fertilizers 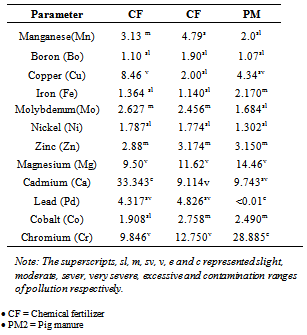 |
| |
|
Biochemical and toxicological responses of earthworm exposured to the two application rates (0.1 and 0.5 g/kg soil) of the chemical (CF) and animal (PM) fertilizer treated soils, indicated significant increase (p<0.05) in concentrations (IU/L) of aspartate transaminase (AST), alanine transaminase (ALT), alkaline phosphatase (ALP), acid phosphatase (ACP), catalase (CAT) and glutathione -s- transferase (GST). Albumim (ALB) showed no significant reduction (p>0.05) across the fertilizers at the two application rates. The result also indicated approximately 20% and 10% death ratios among the earthworms exposed to the chemical and animal fertilizer treated soils respectively. AST showed sensitivity with a significant difference (p<0.05) in concentration of 7.20 IU/L between the 0.1 g/kg soil application rate of the CF treated soil (10.5±0.46 IU/L) and the control (3.30±0.37 IU/L) than ALT which recorded a difference in concentration of 1.66 IU/L at the same application rate. Similar trends were also observed in coelomic AST and ALT levels of earthworms exposed to 0.5 g/ kg soil application rate that showed more significantly different (p<0.05) in concentration of 23.5±0.87 IU/L and 10.12±0.07 IU/L from the control that showed 3.30±0.37 IU/L and 1.8±0.24 IU/L respectively, than AST and ALT in earthworms exposed to PM treated soil.ALP also showed more sensitivity with a significant difference (p<0.05) in concentration of 7.72 IU/L between the 0.1 g/kg soil application rate of the CF treated soil (24.20± 5.91 IU/l) and the control (16.48±0.38IU/L) than ACP which recorded a difference of 5.94 IU/L at the same application rate. This observation was more pronounced in 0.5g/kg soil application rate of the CF treated soil. Though there were an increases in coelomic ALP and ACP levels of the earthworms exposed to PM treated soil at 0.1 and 0.5g/kg soil application rates, they were not significant (p>0.05) when compared with earthworm exposed to the CF treated soil.Significant increase in transaminases (AST and ALT) and phosphatases (ALP and ACP) activity in earthworms exposed to these fertilizers could be due to the possible leakage of enzymes across damaged membranes. Although their precise biochemical mechanisms in the earthworms are not fully understood, increased fertilizer application increased coelomic AST, ALT, ALP and ACP activities of earthworms reflecting a situation of tissue damage.Cells and tissues protect themselves from reactive oxygen species damage by means of enzymes such as catalase and glutathione- s- transferase. Catalase reduces the hygrogen - peroxide. Glutathione –S- transferase plays a key role in the defense of cells against reactive oxygen species and xenobiotics. GST showed more sensitive with a significant difference (p<0.05) in concentration of 47.05 IU/L between the 0.1 g/kg soil application rate of the CF treated soil (95.05± 0.03 IU/L) and the control (48.00± 0.03 IU/L) than CAT which recorded a concentration difference of 2.26 IU/L. The defense enzymes, GST and CAT recorded lower values in the earthworm sample exposed to PM treated soil when compared to the values obtained in the samples exposed to the CF treated soil.The earlier work of[18] and[16] have intrigued the possible detrimental link between trace elements are analogs of toxic metals (eg Zn which is an analogs of Cd). This relative lack of selectivity in transmembrance ion transport may partially explain why toxic metal can enter cells, even against a concentration gradient. Recent research reveals that organisms which cannot excrete heavy metals efficiently appear to be genetically predisposed to this condition[17][19] Recent studies have promoted that heavy metal toxicity is associated with the formation of reactive oxygen species which may causes severe injury/damage to the biological system[19],[5]. This work agrees well with[5] that cellular damage releases enzymes into the blood stream and the levels of these enzymes have the potential to indicate toxicity. The significant increase (p<0.05) in the activity of these enzymes in the earthworms exposed to contaminated soils indicates putative tissue distress to the earthworms.
5. Conclusions
These results showed that heavy metals could interfere with the normal biochemical metabolic processes by the generation of free radicals. The responses were particularly sensitive as significant changes were detected at the lower concentration of 0.1g/kg application rate. The research indicated that AST, ALT, ALP, ACP, CAT and GST can be used as biomarkers of cellular damage, AST, ALP and GST showed more sensitivity with significant difference (p<0.05) in concentrations than ALT, ACP and CAT respectively. These biochemical responses measured in E. fetida (Savigy) could be used for chemical testing in the laboratory or for soil contamination surveys.
ACKNOWLEDGEMENTS
The authors wish to acknowledge the technical assistance received from the staff of Intentional Energy Services Limited Port-Harcourt, River State. Nigeria.
References
[1] | Saint-Denis M, Labrot F. Narbonne J. F, and Ribera D. (1998). Glutathione, Glutathione –Related Enzymes and Catalane Activities in the Earthworm Eisenia Fetida. Archives of Environmental contamination and Toxicity. 35(4) 602-614. |
[2] | Martinez, C.E. and Tabatabai M. A.,(1997).Decomposition of biotechnology by-products in Soils. Journal ofEnvironmental Quality,25:625-632. |
[3] | Dick, W. A., Cheng, I. and Wang, P. (2000). Soil acid and alkaline phosphatase activity is pH adjustment indicators. Soil Biology and Biochemistry, 32 1915-1919. |
[4] | Pramanik, P., Ghosh, G.K., Ghosal, P.K. and Banik, P.,(2007). Changes in organic –C,N,P and K and enzyme activities in vermicompost of biodegradable organic wastes under liming and microbial inoculants. Bioresource Technology. 98(13): 2485-2494. |
[5] | Wang, Z.Z., Zhang, Y. M., Gugo, Y. C., Xia, W. S., Li, Z. W., and Deng, J. F., (1998). Monitoring of Soil heavy metal pollution by earthworm. Environmental Science 4: (10) 1-6. |
[6] | FMA & NR (1989). Fixed Potassium Density Distribution (FPDD) of Federal Ministry of Agriculture and Natural Resources, Adopted for fertilizer used and management practices for crops in Nigeria. Series no. 2. (Revised 1995). |
[7] | Ebong, G.A., Akpan M.M and Mkpenie V.N. (2008). Heavy metal contents of municipal and rural dumposite soils and rate of accumulation by Carica papaya and Talinum triangulare in Uyo, Nigeria. E- Journal of Chemistry 5(2), 281-290. |
[8] | ASTM (1999). American Society for Testing and Materials. Official document on methods of testing all types of materials. Washington D. C. 29. |
[9] | Nwinuka, N.M., Essien, E.B. and Osuji, L.C.,(2003).Soil Analysis. In. Onyeike, E. N. and Osuji J. O.(ed).Research Techniques in biological and Chemical Sciences. Springfield Publishers, Limited. Owerri, Nigeria. 369-401. |
[10] | Reitman, S., and Frankel, S., (1975). A colourimetric method for the determination of serum oxaloacetatic and glutamic pyruvic transaminases. American Journal Clinical Pathology 28: 56-63 |
[11] | King E.J. and Armstrong A.R., (1995). Effect of wilting and processing on the nitrate and nitrite content of some Nigerian vegetable. Nutritional Health 10(3); 269-275 |
[12] | Cheesbrough, M. (2000). Medical Laboratory Manual for Tropical Countries. Microbiology, Linacre House, Jordan Hill Oxford, 260-287. |
[13] | Nwachukwu, M. A., Feng, H. and Alinnor, J.(2010). Assessment of heavy metals pollution in soil and their implications within and around mechanic villages. International Journal of Environmental Science and Technology, 7(2). 347-358. |
[14] | Atafar Z, Messdaghinia A.R, Nouri J. Homaee M, Yunesian M., Ahmadimoghaddam and Mahvi A.H. (2010). Effect of fertilizer application on soil heavy metal concentration. Environmental Monitory and Assessment., 160(1-4), 83-89. |
[15] | Ebong, G. A., Etuk, M.S. and Essien, J.P (2007). Impact of Mechanized Farming on the Heavy Metals Load of an Ultisol Located in the Niger Delta Region of Nigeria. Journal of Applied Sciences, ISSN 1812 – 5654. |
[16] | Alloways , B. J and Ayres, D. C. (1999). Chemical Principles of Environmental Pollution. Blackie Academic and Professional. An imprint of Chapman and Hall, Oxford, UK, 291. |
[17] | Godfrey M., Wojuk D.P., Krone C.A (2003). Apoliporotein E. Genotyping as a potential biomarker for mercury neurotoxicity. J. Alzheimer Dis 5(3): 189-195. |
[18] | Wikipedia,(2003).www.IUPAC.org/heavymetals.html |
[19] | Vutukuru S.S., Prabhath N.A., Raghavender M., and Yerramilli A (2007). Effect of Arsenic and Chromium on the serum Amino-Transferases Activity in Indian Major Carp, Labeo rohita. International Journal of Environmental Research and Public Health. 4(3), 224-227 |
[20] | Buchannan, B.B., Gruissem, W. and Jones, R.C. (2000). Biochemistry and Molecular Biology of plants. 1st Edition, I.K International Pvt Limited Indian 1158-1230. |