Mersiha Suljkanović1, Edita Bjelić2, Jasmin Suljagić2, Azra Kovačević2
1Faculty of Natural Sciences and Mathematics, University of Tuzla, Tuzla, Bosnia and Herzegovina
2Faculty of Technology, University of Tuzla, Tuzla, Bosnia and Herzegovina
Correspondence to: Mersiha Suljkanović, Faculty of Natural Sciences and Mathematics, University of Tuzla, Tuzla, Bosnia and Herzegovina.
Email: |  |
Copyright © 2020 The Author(s). Published by Scientific & Academic Publishing.
This work is licensed under the Creative Commons Attribution International License (CC BY).
http://creativecommons.org/licenses/by/4.0/

Abstract
Among numerous techniques for successful separation and removal of metal cations, transport through bulk liquid membranes attracts special attention of researchers. In this paper, different ligands were applied as possible carriers for Cd(II) ions. Influence of substituents in macrocyclic ligands derived from 18-crown-6 reflected in decreased amount of transported Cd(II) ions, compared to 18-crown-6 (61,26%), in all used solvents, following the order: DCH18C6<DB18C6<B18C6<18C6. Addition of nonionic surfactants as possible carriers for Cd(II) ions within the liquid membranes also resulted with decreased transport rates, due to additional interactions of Cd(II) ions and carriers within the membrane.
Keywords:
Liquid membrane, Cd(II) ions, Surfactants, Macrocyclic ligands
Cite this paper: Mersiha Suljkanović, Edita Bjelić, Jasmin Suljagić, Azra Kovačević, Application of Different Ligands to Optimize Cd(II) Removal Through Liquid Membranes, Advances in Analytical Chemistry, Vol. 10 No. 1, 2020, pp. 7-10. doi: 10.5923/j.aac.20201001.02.
1. Introduction
Since the interactions between metal cations and ligands ("host-guest" interactions) depend on many factors, resulting stability of the formed complex can be increased by varying experimental conditions during transport experiments, e.g. during complex formation in an organic solvent as a membrane. One of the most important factors influencing complex formation is the compatibility between "the host" molecule (ligand) and "the guest" (metal ion). Polyether ligands are among the most suitable host molecules for many metal ions, due to the presence of oxygen atoms as electron donors in their structure, which enables formation of a coordinate covalent bond. Crown ethers have the specific macrocyclic structure of their molecules, consists of the polyether chain-forming "the crown" with hydrophilic cavity and hydrophobic surface.Macrocyclic structure of these ligands provides high stability of formed complexes with metal ions due to the "macrocyclic effect" [1].The affinity of the ligand to the metal ion depends on the structural characteristics of the ligand, and primarily on: the number and type of donor atoms, polarity, the hydrophilic-lipophilic balance of the ligand, chirality and other stereochemical indicators. The cations that best fit the cavity are located in the center and optimize interactions with heteroatoms (oxygen atoms). 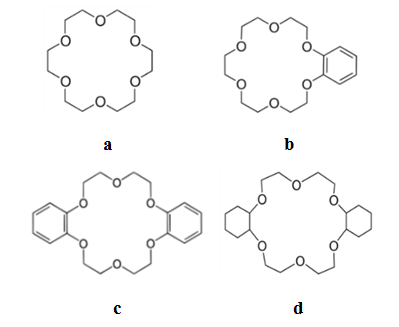 | Figure 1. Structures of polyether ligands: macrocyclic "crown ethers" a) 18-crown-6; b) benzo-18-crown-6; c) dibenzo-18-crown-6; d) dicyclohexano-18-crown-6 |
Specifically, the highest selectivities are expected in cases where the ratios of cation radius and cavity radius of the ligand are closest to 1. Table 1. gives the ionic radius of alkali, alkaline earth and some of the heavy metal cations, as well as the radius of the cavities of the crown ethers determined by CPK (Corey-Pauling-Koltun) molecular models [2].Table 1. Overview of ion radius of alkali, alkaline earth, and heavy metals cations as well as some crown ethers [2] 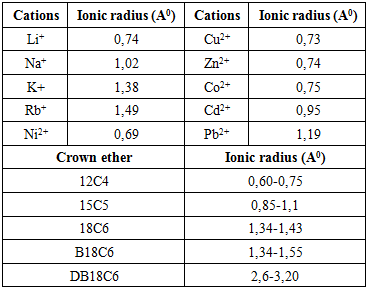 |
| |
|
Application of these systems in transport experiments used for selective removal of toxic metal ions from natural resources is very interesting in recent years [3]. One of the most efficient application of these systems is based on the implementation of the “bulk liquid membrane” (BLM) system, which includes a combination of three processes: extraction, diffusion, and re-extraction of an analyte [4]. In this paper, different ligands were applied for Cd(II) ion complexation. Obtained results are presented as metal ion transport efficiency, expressed through the metal ion concentration in aqueous phases of system, measured with flame atomic absorption spectrometric (FAAS) technique.
2. Material and Methods
MaterialFor every transport experiment, two aqueous solutions and one non-aqueous organic solution (membrane), were prepared, as follows. Source Phase solutions were prepared using the AAS standard metal ion solutions (Cd) from Merck, and picric acid (99%, Kemika) and adjusted to pH = 5, using the acetic buffer solution. Membrane Phase solutions were prepared by dissolving different macrocyclic ligands: 18-crown-6, 18C6; benzo-18-crown-6, B18C6; dibenzo-18-crown-6, DB18C6; dicyclohexano-18-crown-6, DCH18C6 (99%, ACROS ORGANICS) and non-ionic surfactants: Triton X-100, Brij 35, Brij 58, Brij 78 (p.a. Sigma-Aldrich) in different organic solvents (p.a. Kemika): dichloromethane (DCM), chloroform (CH), 1,2-dichloroethane (12-DCE). Receiving Phase solutions were prepared also in acetic buffer medium (pH = 5), by dissolving sodium thiosulphate (p.a. Sigma-Aldrich) in it.InstrumentspH measurements of aqueous solutions were performed using the pH meter (GLP31 Crison Instruments).Quantification of metal ions removed during the transport experiments were obtained by Flame Atomic Absorption Spectrometry technique, using the instrument Perkin Elmer AAnalyst 200. Transport ProcedureTransport experiments involved the application of a cylindrical glass vessel, "transport cell" (Figure 2.), with an inner diameter of 5 cm, containing a glass tube (2 cm in diameter) in a central position. The central tube represents a physical barrier between the two aqueous phases. The source phase (SP) contained 10 mL of a mixture of tested metal ion (1∙10-3mol/L) and the counter ion, picrate (1∙10-3 mol/L). The receiving phase (RP), which is outside the central tube, contained a stripping agent (thiosulphate). The membrane phase (MP) contained 50 mL of a suitable ligand (1∙10-3mol/L) dissolved in an organic solvent; the membrane layer lies beneath the aqueous phases and connects them. The membrane phase is mixed with a magnetic stirrer so that under these conditions the contact surfaces between the aqueous phases are straight and precisely defined [5]. Both aqueous phases were analyzed by atomic absorption spectrometry after 3hours, and the concentration of metal ions transported through the membrane was measured.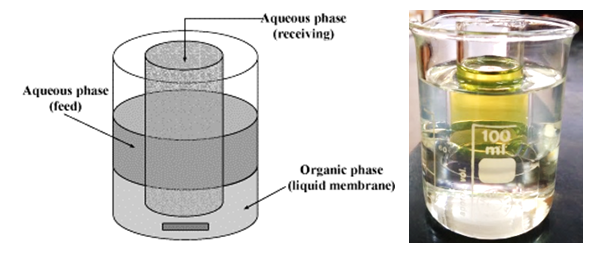 | Figure 2. "Transport cell" [6] used for the experiment: SP-source phase; RP-receiving phase; MP-membrane phase; A-magnetic stirrer |
3. Results and Discussion
In earlier investigations, authors [7], [8] assumed that the most influencing process during transport experiments was metal ions release from complex in membrane phase to receiving aqueous phase through contact surface between two phases. Based on this assumption, the mechanism for metal ion transport was proposed [7].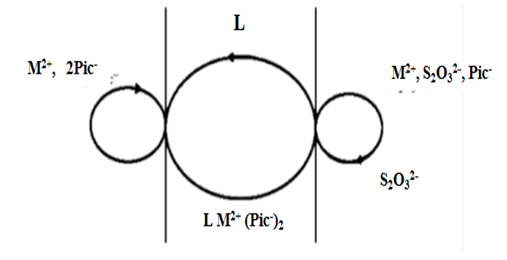 | Figure 3. Proposed mechanism of metal ion transport through an organic liquid membrane containing dissolved ligand (L), from SP (contains: M2+ cations and counterions picrate Pic-) to RP (contains thiosulphate ions) |
3.1. Macrocyclic Carrier Study
BLM systems with membrane phase containing different macrocyclic ligands, (18C6; DB18C6; B18C6 and DCH18C6) was examined using different liquid membranes: CH, DCM and 1,2-DCE. CH membrane with 18C6 as a carrier showed better transport efficiency, for all ligand systems investigated (Table 2). Table 2. Comparation of Cd(II) transport efficiency through CH, DCM and DCE membranes for different ligands: 1 – 18C6; 2 – DB18C6; 3 – B18C6 4 – DCH18C6 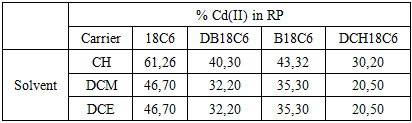 |
| |
|
Although the ring size and cavity diameter remain the same as for 18C6 (1,34 A0), the presence of benzo- and cyclohexyl- groups in the macrocyclic structure probably reduce interactions between these substituted macrocycles (B18C6, DB18C6, DCH18C6) and cations, which lead to a lower transport rate. Also, slightly higher transport rate for B18C6 compared to DB18C6 is probably related to higher rigidity of double substituted DB18C6 ring. Actually, DB18C6 has the most rigid structure among the crown ethers studied [9], [10].Also, the addition of two benzo groups (as in DB18C6), caused the value of complex stability constant to decrease due to the loss of the flexibility of the ring, resulting with electron withdrawal from oxygen atoms, which consequently reduces the interactions between cations and ligands, lowering the transport efficiency. On the other hand, for DC18C6, the presence of two cyclohexyl groups causes an increase in the basicity of the oxygen atoms in comparison with DB18C6, which leads to the ability of the DC18C6 complex to adapt to different conformations as required for greater stability of the complex [11].
3.2. Study of Surfactants as Additional Carriers
The possibility of using nonionic surfactant TX-100 as carrier for Cd(II) ions in liquid membrane systems has been investigated (Figure 4.) TX-100 addition resulted with the content of Cd (II) ions in the RP of 52%, which is lower compared to the system with only 18C6 (>60%). Comparation between systems using other macrocyclic ligands with addition of TX-100, showed also the reduction of transport in the RP. Stronger interactions within the membrane (cation-18C6-surfactant) may result in difficult cation release at the MP / RP boundary, either due to the formation of micellar structures in the membrane (incorporating the formed complexes), or due to the additional complexation of the cation by the surfactant as a ligand. 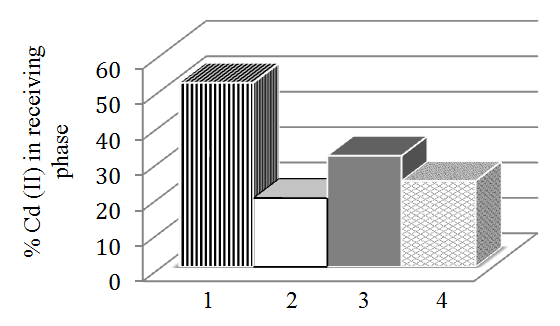 | Figure 4. Comparation of Cd(II) transport efficiency through CH membranes for different ligands: 1 –18C6+TX100; 2- DB18C6+TX100; 3–B18C6+TX-100; 4-DCH18C6+TX100 |
Previous conclusion about influence of nonionic surfactant can be obtained also from the study of other nonionic surfactants: (Brij 35, Brij 58 and Brij 78) used in BLM systems with macrocyclic ligands 18C6 and B18C6, within chloroform as a liquid membrane (Figure 5.). 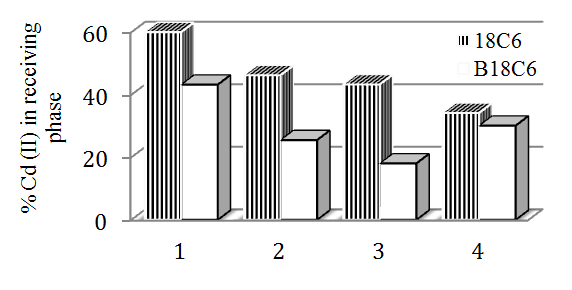 | Figure 5. Comparation of Cd(II) transport efficiency through CH membranes for different ligands: 1 –18C6; B18C6 2 –18C6+Brij78; B18C6+Brij78; 3- 18C6+Brij 58; B18C6+Brij58 4-18C6+Brij35; B18C6+Brij35 |
4. Conclusions
18-crown-6 ether is the most suitable for complexation of Cd(II) ions compared to dibenzo-18-crown-6, benzo-18-crown-6 and dicyclohexano-18-crown-6 due to its greater flexibility. Presence of nonionic surfactants TX-100, Brij 35, Brij 78 and Brij 58 with crown ether in membrane decreases transport efficiency due to incorporation of formed complexes into the micellar structures (reverse micelles) which stabilize them, preventing decomplexation and metal ions releasing from membrane to receiving phase.
References
[1] | Geary, C.D., Zudans, I., Goponenko, A.V., Asher, S.A., Weber, S.G. (2005) Electrochemical investigation of Pb2+ binding and transport through a polymerized crystalline colloidal array hydrogel containing benzo-18-crown-6., Anal. Chem. 77, 185. |
[2] | Vögtle, F. (1995) Supramolecular Chemistry. Concepts and Perspectives, VCH, Weinheim. |
[3] | Kellner, R., Mermet, M., Otto, H. Widmar (1998) Analytical chemistry, Wiley, Weinheim, 293. |
[4] | ShokrollahiI A, Ghaedi M, Shamsipur M (2009) Highly selective transport of mercury (II) ion through a bulk liquid membrane. Quim Nova 32, 153–157. |
[5] | Nipamanjari, Deb, Sanjib Bagchi, Asok, K., Mukherjee (2010) Charge transfer complex formation between TX-100/CCl4. Molecular Physics 108 (11), 1505–1511. |
[6] | Fahmideh-Rad E., Rounaghi GH., Arbab Zavar MH., Chamsaz M. (2010) Spectrometric determination of Pb2+ cation after selective bulk liquid membrane transport using benzo-18-crown-6 as carrier. Der Pharma Chemica 2(6),8-18. |
[7] | Malihe, S. Kazemi, Golamhossein Rounaghi (2010) Highly selective transport of lead cation through bulk liquid membrane by macrocyclic ligand of decyl-18-crown-6 as carrier, Russian Journal of Inorganic Chemistry 55 (12), 1987–1991. |
[8] | Reusch, C.F, Cussler E.L (1973) Selective membrane transport, AlChE J. 19(4) 736-741. |
[9] | Kashanian, S., Shamsipur, M., (1989) Complex formation of alkaline earth cations with benzo-15-crown-5 and some 18-crowns in methanol, dimethylformamide and dimethyl sulfoxide solutions, Inorg Chim. Acta 155, 203-206. |
[10] | Amini, M.K., Shamsipur, M, (1992) Complex formation of hydronium ion with several crown ethers in 1,2-dichloroethane, acetonitrile, and nitrobenzene solutions, J Solution Chem 21, 275-288. |
[11] | Salman, S.R., Derwish, G.A.W., Al-Marsoumi S.M.H. (1996) Molecular complexes of crown ethers, part 4 Complexes of crown ethers with picric acid. Journal of Inclusion Phenomena and Molecular Recognition in Chemistry 25, 295-302. |