Joseph C. Akan1, Musa M. Mahmud2, Maimuna Waziri3, Zakari Mohammed4
1Department of Chemistry, University of Maiduguri, Maiduguri, Nigeria
2School of General Studies, Mai IdrisAlooma Polytechnic, Geidam, Nigeria
3Department of Chemistry, Federal University Gashua, Nigeria
4Department of Chemistry, Federal University Dutse, Dutse, Nigeria
Correspondence to: Joseph C. Akan, Department of Chemistry, University of Maiduguri, Maiduguri, Nigeria.
Email: |  |
Copyright © 2015 Scientific & Academic Publishing. All Rights Reserved.
Abstract
Watermelon (Citrulus lanatus) samples were freshly harvested from the Mashangwari, Katakamand Krigasawaagricultural locations in Gashua, Bade Local Government Area, Yobe State Nigeria. The watermelon samples were divided into peel, pulp, seed, leaf, root and stem for the determination of some organochlorine pesticide residues (o, p-DDE, p,p’-DDD, o,p’-DDD, p,p’-DDT, dieldrin and aldrin). Soil samples were also collected at different depths for the determination of the above pesticides. Sample collection and preparation was conducted using standard procedures. The concentrations of all the pesticides in the soil and watermelon samples were determined using GC/MS SHIMADZU (GC-17A) equipped with electron capture detector (ECD). The determined pesticides were detected in all the watermelon and soil samples from the three agricultural locations. For DDT and its metabolites, p,p’-DDD showed the highest concentration with a value of 0.08±0.03 µg/g in the peel from the Mashangwari agricultural location, while the lowest concentration of 0.01±0.01 µg/g of p,p’-DDT was observed in the root. For the Katakam and Krigasawaagricultural locations, o,p’-DDE showed the highest concentrations of 0.09±0.04 and 0.09±0.03 µg/g. The concentrations of dieldrin and aldrin from the three agricultural locations were observed in the range of 0.01±0.01 and 0.28±0.06 µg/g. The highest concentration of 0.28±0.06 µg/g dieldrin was observed in the peel, while the root showed the lowest value. The total o,p:-DDE, p,p’-DDD, o,p’-DDD, p,p’-DDT in the peel, pulp than leaf, seed, roots and stem of the watermelon samples from the three agricultural locations were lower than the EU set maximum residue limit (MRL) for total DDT, DDD and DDE in watermelon which is 0.05µg/g, while the concentrations of aldrin and dieldrin found in all the watermelon samples from the three agricultural locations were much higher than the EU set maximum residue limits (MRLs) 0.01 µg/kg. These high concentrations of aldrin and dieldrin could probably explain their persistence in the environment or continued use in the study area. Despite bans and restrictions on the use of some of these pesticides in Nigeria, the observed concentrations of the studied pesticides from the agricultural locations could explain either their persistence in the environment or continued use in the study area. Hence, routine monitoring of pesticide residues in these study locations is necessary for the prevention, control and reduction of environmental pollution, so as to minimize health risks.
Keywords:
Organochlorine, Watermelon, Soil, Pesticide, MRL
Cite this paper: Joseph C. Akan, Musa M. Mahmud, Maimuna Waziri, Zakari Mohammed, Residues of Organochlorine Pesticides in Watermelon (Citrulus lanatus) and Soil Samples from Gashua, Bade Local Government Area Yobe State, Nigeria, Advances in Analytical Chemistry, Vol. 5 No. 3, 2015, pp. 61-68. doi: 10.5923/j.aac.20150503.03.
1. Introduction
Watermelon (Citrulus lanatus), a member of the Cucurbitaceae family, is related to the cantaloupe, squash, pumpkin and other plants that also grow on vines on the ground. In Nigeria, watermelon is mostly cultivated in the Northern part because it is a warm-loving plant; this makes its production seasonal in the Southern part of the country. The crop is affected by excess water, especially in its least developmental stage (Feher, 1993; Athens, 1998). The sugar content of the fruit is diluted and further internal pressure, due to excess absorption of water could lead to cracking and spoilage of the fruit thus; edibility and market yield significantly (Boyhan and Masiunas, 1999; Lacier and Plummer, 2003). The high demand of this vegetable fruit is not met in the Southern part of the Nigeria because this part has a high rainfall covering many months of the year. The production of watermelon all year round in all parts of Nigeria is expected to improve nutrition, contribute to food security as well as increase revenue of the producers/farmers and create employment opportunities thereby improving the efficiency of utilization of labour (Musmade and Desai, 2001). Watermelon diseases caused by fungi, bacteria, nematodes and viruses are one of the main limiting factors in obtaining higher yields. One of the solutions is to apply pesticides at many steps of the cultivation process. Even when applied in accordance with Good Agricultural Practices (GPA), they can leave residues, which can be detrimental to watermelon safety. The presence of pesticide residues in fruits in general and in watermelon in particular, is one important concern for consumers due to their possible long adverse health effects; especially for children, as they consume a higher proportion of fruits in relation to their body weight and are more susceptible to chemicals since they are in early development stages (Zawiyah et al., 2007).Organochlorines (OCPs) are chlorinated organic compounds used predominantly as insecticides. They are mainly classified into three categories; namely diphenylaliphatics such as DDT and its metabolite, cyclodienes which include aldrin, dieldrin, endrin, heptachlore, endosulfan, and endosulfan sulfate (Nur-Banu and Semra, 2004). These pesticides are typically very persistent in the environment, and are known to accumulate in sediments, plants and animals. Most of them break down slowly and can remain in the environment long after application and in organisms long after exposure (Nur-Banu and Semra, 2004). Organochlorine pesticides are broad spectrum insecticides, active against a great variety of pests. They vary in their chemical structures. The OCPs and their metabolites are mainly classified into three categories; namely diphenylaliphatics, cyclodienes and hexachlorocyclohexanes (Nur-Banu and Semra, 2004).Gashua is located in Yobe State, intense watermelon agricultural activities take place in the area, and is 10 km along the Lake Chad Basin area. The people in the areas are 98% predominantly farmers out of this, merely 85% are crop cultivating farmers. The watermelon production farmers in the study area use pesticides to control pests, diseases, weeds and other plant pathogens in an effort to reduce or eliminate yield losses and preserve high production quality. Lack of knowledge of the uses and effect of these pesticides among small and large scale farmers has resulted in contamination of the crops and soil with pesticide. Such contamination might be absorbed by the plant or vegetable while others leached to the underground water. Such accumulation by plants may affect humans and other species that depend on such watermelon and plants as food. Gashua in Bade Local Government Area (L.G.A), is primarily an agricultural area with intense pesticide usage for cultivating watermelon. Watermelon constitutes an important source of vitamin and mineral for the inhabitants in the area and around the country (Parsons, 2002). Agricultural activities have impacted negatively on the soil. Bioaccumulation and bio-concentration of pesticides in the watermelon is capable of reaching toxic levels in the watermelon even when exposure is low. The study of the levels of pesticide residue in watermelon is very limited in the country. Therefore, the contamination status of watermelon by pesticide residues is unknown. This calls for an extensive study of the pesticide residues status of watermelon in Gashua, Bade Local Government Area.
2. Material and Methods
2.1. Sample Collection
Fresh mature watermelon samples of uniform colour and size were collected from three different agricultural locations namely Mashangwari, Katakam and Krigasawa in Gashua, Bade Local Government Area Yobe State, Nigeria. The leaf, stem and root of watermelon samples were also collected and transported to the laboratory, stored at 25°C for a period of six month. Soil samples were collected from ten plots. In each plot, soil was collected at three depths (0-10 cm, 10-20 cm and 20-30 cm), by using a spiral auger of 2.5 cm diameter. Soil was randomly sampled and bulked together to form a composite sample, collected in clean plastic bags and transported to the laboratory.
2.2. Sample Preparation
The watermelon was thoroughly washed with distilled water to remove dirt, dust, pesticide residues and then washed with pure ethanol to remove micro flora on the surface of the fruit prior to extraction. All glassware and knives were autoclaved at 121°C for 45 min and all other equipments were sanitized with hypochlorite prior to usage. Each unit sample of watermelon was cut into 6 vertical pieces and then divided into peel, pulp and seed portions. Each prepared sample was maintained frozen at −20°C until when needed for analysis and was individually homogenized using a blender (BLIXER-5Plus) immediately before analysis.
2.3. Extraction of Watermelon Samples
Each homogenized (20 g of pulp and 10 g each of peel, seed, leaf, stem and root watermelon respectively) was weighed into an Erlenmeyer flask and extracted using 100 ml of acetonitrile by shaking for 30 min using a reciprocal shaker. The mixture was then filtered by vacuum suction and the residual cake was washed using 50 ml acetonitrile. The filtrates were combined and made up to 200 ml using acetonitrile.
2.4. Clean-up of Sample Extracts
A 2 ml aliquot of the acetonitrile extract was cleaned using solid-phase extraction with a styrene-divinylbenzene cartridge. Eight-ml of water was added to the acetonitrile extract and the mixture was loaded onto the cartridge. The cartridge was washed using 10 ml acetonitrile: water (1: 4, V/v) and 15 ml tetrahydrofuran was passed through the cartridge; the eluate was evaporated to dryness using a rotary evaporator and nitrogen blow down apparatus. The residue was dissolved and diluted using 10 ml of acetonitrile: toluene (3: 1, V/v) and loaded onto a graphite carbon black cartridge (500 mg/6 ml, (Supelclean ENVI-Carb; Sigma- Aldrich, MO, USA); conditioned with the acetonitrile: toluene mixture). An additional 20 ml of the acetonitrile: toluene mixture was passed twice through the cartridge. All of the eluate was collected in a round-bottom flask and evaporated to dryness using a rotary evaporator and nitrogen blow down apparatus, after which the residue was dissolved and diluted using a suitable volume of acetonitrile: water (4 : 1, V/v) mixture.
2.5. Extraction of Soil Sample
Soil samples were extracted using a soil-packed bulb column. Each sample (25 g) was weighed into a glass jar, the soil got fortified at this step, before adding pre-cleaned sand (25 g) and granular sodium sulphate (50 g). The sample mixture was manually shaken for 30 sec, placed on a roller for 30s and then allowed to stand for 20 min to provide time for the sodium sulphate to adsorb any residual moisture from the soil. The sample mixture was transferred to a 250 ml bulb column and the sample jar was triple rinsed with small amounts of 5 ml hexane and transferred to the bulb column. The soil contents were extracted with acetone: hexane (1:1 V/v, 250 ml mixture) and the eluate collected and concentrated to 100 ml using a rotary evaporator. The soil extract was then subjected to additional clean-up.
2.6. Clean-up of Soil Extracts
The concentrated soil eluate was washed by liquid-liquid partitioning with saturated sodium sulphate (25 ml) and distilled water (300 ml) in a separatory funnel (500 ml). After shaking, the aqueous layer was drained into a beaker and the hexane was transferred to a separatory funnel (250 ml). The aqueous layer was returned to the 500 ml separatory funnel and re-extracted with 15% dichloromethane in hexane (40 ml). The organic layers were combined in the 250 ml separatory funnel and gently washed with distilled water (100 ml) for about 30 sec. After discarding the aqueous layer, the organic layer was filtered through sodium sulphate, evaporated to near dryness on a rotary evaporator; the sides of the flask were rinsed down with hexane (20 ml), and evaporated to about 1 ml. The sample extract was quantitatively transferred to a centrifuge tube, concentrated on a nitrogen evaporator to 0.5 ml, and diluted to 2.0 ml final volume in hexane prior to Gas-Chromatographic (GC) analysis.
2.7. Determination of Pesticide Residues
The SHIMADZU GC/MS (GC – 17A), equipped with electron capture detector was used for the chromatographic separation and was achieved using a 35% diphenyl/65% dimethyl polysiloxane column. The oven was programmed as follows: initial temperature 40°C, 1.5 mins; to 150°C, 0.0 min. 5°C /min. to 200°C, 7.5 mins; 25°C /mins; to 290°C with a final hold time of 12 mins. and a constant column flow rate of 1mL/min. The detection of pesticides were performed using the GC-ion trap MS with optional MSn mode. The scanning mode offered enhances selectivity over either full scaned or selected ion monitoring (SIM). In SIM, at the elution time of each pesticide, the ratio of the intensity of matrix ions increase exponentially versus that of the pesticide ions as the concentration of the pesticide approach the detection limit, decreasing the accuracy at lower levels. The GC-ion trap MS was operated in MSn mode and performed tandem MS function by injecting ions into the ion trap and destabilizing matrix ions, isolating only the pesticide ions. The retention time, peak area and peak height of the samples were compared with those of the standards for quantization.
3. Results
The concentrations of some organochlorine pesticide residues in the peel, pulp, seed, leaf, root and stem of watermelon samples from Mashangwari agricultural location are as presented in Table 1. The concentrations of o,p’-DDE ranged from 0.01±0.01 to 0.06±0.04 µg/g; 0.01±0.01 to 0.08±0.03 µg/g p,p’-DDD; 0.01±0.01 to 0.052±0.02 µg/g o,p-DDT; 0.01±0.01 to 0.02±0.01 µg/g p,p-DDT. For aldrin, the concentrations ranged from 0.01±0.01 to 0.12±0.01 µg/g while the concentrations of dieldrin ranged from 0.02±0.03 to 0.22±0.06 µg/g. The highest concentration of o,p’-DDE in the watermelon sample was observed in the peel with a value of 0.06±0.04 µg/g, while the lowest concentration of 0.01±0.01 µg/g was observed in the root. For p,p’-DDD, the highest concentration of 0.08±0.03 µg/g was also observed in the peel, while the lowest concentration was also in the root. Similarly, for o,p-DDD, p,p-DDT, dieldrin and aldrin the highest concentratrations were observed in the peel with values ranging from 0.22±0.06 µg/g to 0.15±0.03 µg/g, while the lowest concentrations were observed in the root with values ranging from 0.02±0.01 to 0.01±0.01 µg/g. For Katakam Agricultural location, the concentrations of organochlorine pesticide residues in the peel, pulp, seed, leaf, root and stem of watermelon samples are as presented in Table 2. The concentrations of o,p’-DDE ranged from 0.01±0.01 to 0.09±0.04 µg/g; 0.01±0.01 to 0.07±0.04 µg/g p,p’-DDD; 0.01±0.01 to 0.07±0.03 µg/g o,p’-DDT and 0.01±0.01 to 0.04±0.01 µg/g p,p’-DDT. For dieldrin, the concentration ranged from 0.04±0.02 to 0.028±0.06 µg/g while the concentration of aldrin ranged from 0.02 ± 0.01 to 0.20±0.04 µg/g. Similarly, the concentration of some organochlorine pesticide residues in peel, pulp, seed, leaf, root and stem of watermelon samples from Krigasawa location are as presented in Table 3. The concentrations of o,p-DDE ranged from 0.02±0.01 to 0.09±0.03µg/g; 0.02±0.01 to 0.07±0.01 µg/g p,p’-DDD; 0.01± 0.01 to 0.03± 0.02 µg/g o,p’-DDD and 0.01±0.01 to 0.02±0.01 µg/g p,p’-DDT. For dieldrin the concentrations ranged from 0.02±0.01 to 0.15±0.04 µg/g, while the concentration of aldrin ranged from 0.01±0.01 to 0.09±0.05 µg/g. The highest concentration of o,p-DDE in the watermelon sample from the Katakam agricultural location was observed in the peel with a value of 0.09±0.04 µg/g, while the lowest concentration of 0.01±0.01 µg/g was observed in the root. For p,p-DDD, the highest concentration of 0.07±0.04 µg/g was observed in the peel, while the lowest concentration of 0.01±0.01 µg/g was also in the root. Similarly, the highest concentrations of o,p-DDD, p,p-DDT, aldrin and dieldrin were found in the peel with values ranging from 0.04±0.01 µg/g to 0.28±0.06 µg/g, while the lowest concentrations were observed in the root with values ranging from 0.01±0.01 to 0.02±0.01 µg/g respectively. A similar trend was also observed in the Krigasawa agricultural location.Table 1. Mean Concentrations of Some Organochlorine Pesticide Residues in Different Parts of Watermelon Samples from Mashangwari Agricultural Location, Gashua, Yobe State 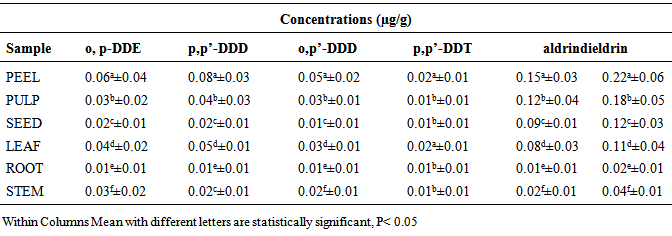 |
| |
|
Table 2. Mean Concentrations of Some Organochlorine Pesticide Residues in Different Parts of Watermelon Samples from Katakam Agricultural Location, Gashua, Yobe State 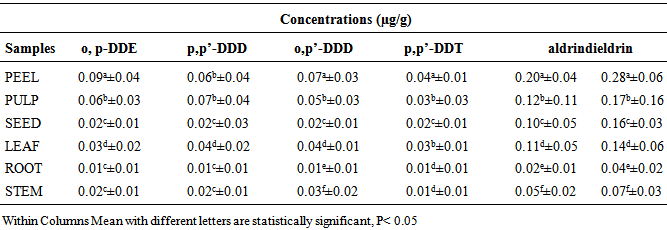 |
| |
|
Table 3. Mean Concentrations of Some Organochlorine Pesticide Residues in Different Parts of Watermelon Samples from Krigasawa Agricultural Location, Gashua, Yobe State 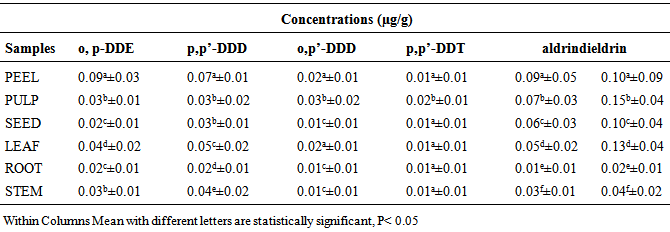 |
| |
|
The concentrations of organochlorine pesticide residues in different depth (0-10, 11-20 and 21-30 cm) of soil samples at the Mashangwari, Katakam and Krigasawa agricultural location is as presented in Figure 1. The concentration of o,p-DDE ranged from 0.54 to 0.82 µg/g; 0.54 to 0.77 µg/g p,p-DDD; 0.43 to 0.64 µg/g o,p-DDD; 0.27 to 0.43 µg/g p,p-DDT. For dieldrin, the concentration ranged from 1.23 to 1.56 µg/g, while the concentration of aldrin ranges from 1.33 to 162 µg/g. The highest concentration of o,p’-DDE in the soil sample was observed at a depth of 21-30cm with a value of 0.82 µg/g, while the lowest concentration of 0.54 µg/g was observed at a depth of 0-10 cm. For p, p’-DDD, the highest concentration of 0.77 µg/g was also observed 21-30 cm while the lowest concentration of 0.54 µg/g was also observed 0-10 cm. Similarly, the highest concentrations of o,p’-DDD, p,p’-DDT, dieldrin and aldrin were observed at a depth of 21-30 cm with values ranging from 0.43 to 1.62 µg/g, while the lowest concentrations were also observed at a depth of 0-10 cm with values ranging from 0.27 to 1.33 µg/g respectively.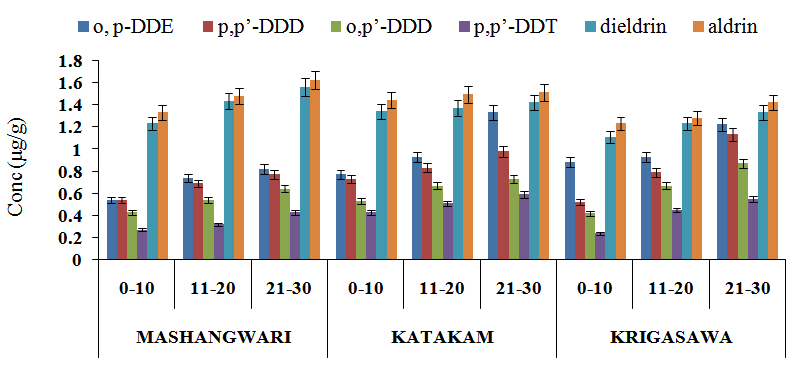 | Figure 1. Mean Concentrations of Some Organochlorine Pesticide Residues in Different Depth of Soil Samples from Mashangwari, Katakam and Krigasawa Agricultural Location, Bade Local Government Area, Yobe State |
For Katakam agricultural location, the concentration of ofo,p-DDE in different depth (0-10, 11-20 and 21-30 cm) ranged from 0.77 to 1.33 µg/g; 0.73 to 0.98 µg/g p,p’-DDD; 0.53 to 0.73 µg/g o,p’-DDD and 0.43 to 0.59 µg/g p,p’-DDT, that of dieldrin ranges from 1.34 to 1.42 µg/g. The concentration of aldrin ranges from 1.44 to 1.51 µg/g. The highest concentration of o,p-DDE in the soil sample was observed at 21-30 cm depth with a value of 1.33 µg/g, while the lowest concentration of 0.77 µg/g was observed at a depth of 0-10cm. For p,p-DDD, the highest concentration of 0.98 µg/g was also observed 21-30 cm, while the lowest concentration of 0.73 µg/g was also observed 0-10 cm. Similarly, the highest concentrations of o,p-DDD, p,p-DDT, dieldrin and aldrin were observed at a 21-30 cm depth with values ranging from 0.59 to 1.51µg/g, while the lowest concentrations were also observed at a depth of 0-10 cm with values ranging from 0.43 to 1.44 µg/g respectively.At the Krigasawa agricultural location, the concentrations of o,p-DDE ranged from 0.88 to 1.22 µg/g; 0.52 to 1.13 µg/g p,p-DDD; 0.42 to 0.87 µg/g o,p’-DDD; 0.24 to 0.55 µg/gp,p’-DDT. For dieldrin, the concentration ranges from 1.11 to 1.33 µg/g, while the concentration of aldrin ranges from 1.23 to 1.42 µg/g. The highest concentration of o,p-DDE was observed at a depth of 21-30 cm with a value of 1.22 µg/g, while the lowest concentration of 0.88 µg/g was observed at a depth of 0-10 cm. For p,p-DDD, the highest concentration of 1.13 µg/g was also observed at 21-30 cm depth, while the lowest concentration of 0.52 µg/g was also observed at a depth of 0-10 cm. The highest concentrations of o,p-DDD, p,p-DDT, dieldrin and aldrin were observed at 21-30 cm depth with values ranging from 0.55 to 142 µg/g, while the lowest concentrations were also observed at a depth of 0-10 cm with the values ranging from 0.24 to 1.23 µg/g respectively.
4. Discussion
The concentration of o,p:-DDE, p,p’-DDD , o,p’-DDD, p,p’-DDT were detected in all the watermelon samples from the three agricultural locations. The highest concentration of o,p-DDE in the watermelon samples was significantly higher in the peels with a value of 0.06±0.04 µg/g, while the lowest concentration of 0.01±0.0102 µg/g was significantly observed in the root (p<0.05). For p,p-DDD, the highest concentration of 0.08±0.03 µg/g was observed in the peels, while the lowest concentration was also observed in the root. Similarly, the highest concentrations of o,p-DDD and p,p-DDT were statistically detected in the peels with values of 0.05±0.02 µg/g and 0.02±0.0102 µg/g, while the lowest concentration was observed in the root with a value of 0.01±0.01 µg/g Table 1. The highest concentration of o,p-DDE in the watermelon sample from the Katakam agricultural location was observed in the peels with a value of 0.09±0.04 µg/g, while the lowest concentration of 0.01±0.01 µg/g was observed in the root. For p,p-DDD, the highest concentration of 0.07±0.04 µg/g was statistically observed in the peel, while the lowest concentration of 0.01±0.01 µg/g was statistically observed in the root. Similarly, the highest concentrations of o,p-DDD and p,p-DDT were statistically observed in the peels with values of 0.07 ± 0.03 µg/g and 0.04±0.01 µg/g while the lowest concentrations were observed in the root with values ranging from 0.01±0.01 µg/g to 0.02±0.01 µg/g Table 2. Similar trend were also observed from the Krigasawa agricultural location Table 3.The total concentrations of o,p:-DDE, p,p’-DDD and o,p’-DDD in all the watermelon samples from the three agricultural locations were significantly higher than that of p,p’-DDT (p<0.05). The pesticide DDT gradually degraded to DDE and DDD through biological and photochemical transformations under both aerobic and anaerobic conditions (Thomas et al., 2008). This supports the high concentrations of o,p:-DDE, p,p’-DDD and o,p’-DDD when compared to p,p’-DDT. The DDT/ DDE and DDD ratio is usually used to know whether DDT input has recently occurred (Doonget al. 2002). DDT is generally used against a wide variety of agricultural and forest pests and against insect pests including vectors such as mosquito and tse-tse flies. The concentrations of o,p:-DDE, p,p’-DDD, o,p’-DDD and p,p’-DDT were significantly higher in the fruit (peel and pulp) than in the leaf, seed, root and finally the stem. The higher concentration of DDT and its metabolites in the peel and pulp might be due to photosynthesis within the plant leaf to produce the fruit. A similar study was carried out by (Santamaria et al., 1999) which showed that the pollutants content of various parts of plant differs. They reported that in vegetable organs the concentrations of pollutants are in the order of fruit> leaf> stem> root> tuber> bulb>seed. The total o,p:-DDE, p,p’-DDD , o,p’-DDD, p,p’-DDT in the peel, pulp than leaf, seed, roots and stem of the watermelon samples from the three agricultural locations were lower than the EU set maximum residue limit (MRL) which is 0.05µg/g. The low level of DDT instead of DDE as seen on Table 1, 2 and 3 suggests that DDT had been metabolized to DDE over time, and is currently in use for control of pests in the three agricultural areas. There were no statistical differences (p>0.05) in the levels of o,p:-DDE, p,p’-DDD , o,p’-DDD, p,p’-DDT from the three agricultural locations, which probably indicates that there are similarities in the levels of applications of these pesticides by farmers. The difference in pesticide concentration in the parts of these vegetables i.e root, stem and the leaf is as a result of uptake of nutrients from the root to the leaf for photosynthesis. The detection of this pesticide residues in watermelon samples collected from the three agricultural locations, indicates that, like other developing countries, such pesticides are still in use here. Although the use of DDT and its metabolites has been banned in Nigeria since 2008, it is still being used. DDT and its metabolites persist in the environment and is known to bioaccumulate (Ware, 1978). DDT and its metabolites have been classified by NAFDAC as probable human carcinogens. Despite bans and restrictions on the usage of these pesticides in Nigeria, the observed concentrations of the studied organochlorine pesticides in the watermelon samples from the three agricultural locations could explain either their persistence in the environment or continued use in the study area.The highest concentration of o,p’-DDE in the soil sample from the Mashangwari, Katakam and Krigasawa Agricultural locations were observed at a depth of 21-30 cm with a value of 0.82 µg/g, 1.33 µg/g and 1.22 µg/g respectively, while the lowest concentration of 0.54 µg/g, 0.77 and 0.88 µg/g were observed at a depth of 0-10 cm. For p, p’-DDD, the highest concentration of 0.77 µg/g, 0.98 µg/g and 1.13 µg/g were also observed at a depth of 21-30 cm, while the lowest concentration of 0.54 µg/g, 0.73 µg/g and 0.52 µg/g were also detected at a depth of 0-10 cm. Similarly, the highest concentrations of o,p’-DDD, p,p’-DDT, dieldrin and aldrin were observed at a depth of 21-30 cm with values ranging from 0.55 to 1.62 µg/g, while the lowest concentrations were also observed at a depth of 0-10 cm with values ranging from 0.27 to 1.33 µg/g in Figure 1. All the pesticides used are mostly synthetic organic compounds. The soil may act as an important sink for persistent organic pollutants including many pesticides used presently or in the past. They are relatively insoluble in water and are retained strongly by the soil. The soil acts as a filter and buffer and degradation of pollutants with respect to storage of pollutants with the help of soil organic carbon. The soil acts also as a pathway for pesticide transport to contaminate ground/ surface water, plants, food and affects human via runoff, leaching, transfer of mineral nutrients and pesticides from soil into the plants and animals that constitute the human food chain. Persistent pesticides slowly break down into the soil and lead to contamination which is closely correlated to human activities, agricultural applications and deforestation which leads to soil erosion (Bhattacharya et al., 2003). This explains the high concentration of the pesticide at a depth of 21-30cm.Several studies (Singh et al., 2005; Gaoet al., 2005) have reported the detection of pesticides in soil and the most frequent pesticides detected were the organochlorines (OC) which is more persistent and stays in the soil, decomposes very slowly and may persist for several years as they are insoluble in water and are retained by the soils. Studies of pesticide residues have been reported by several researchers (Baig, 1999, Jabbaret al., 1993; FAO, 2002; Sanperaet al. 2002). (Jabbaret al., 1993) studied the hydrophobicity and persistence of pesticides that controlled the accumulation in different soil series of Pakistan. It was observed that less water soluble (<1 mg L-1) pesticides have the potential to accumulate in sediment and aquatic biota, which is in full agreement with the present study that most pesticides used in the three agricultural locations were insoluble in water that is why they persist in the soil without any influence of temperature, humidity and microbial activity affecting them. The highest concentrations of dieldrin and aldrin were significantly observed in the peels with values of 0.15±0.03 and 0.22±0.06 µg/g, while the lowest concentrations were observed in the root with a value of 0.01±0.01 and 0.02±0.01 µg/g. From the Katakam and Krigasawa agricultural locations, the highest concentrations of dieldrin and aldrin were detected in the peels with values of 0.20±0.04 and 0.28±0.06 µg/g, while the lowest concentrations were observed in the root with values ranging from 0.02±0.01 to 0.04±0.02 µg/g. Aldrin is an alicyclic chlorinated hydrocarbon and is rapidly converted to the epoxide dieldrin (GESAMP, 1993). The presence of higher significant concentrations of dieldrin recorded in the analyzed samples from the three locations as against aldrin (Table 4.1, 4.2 and 4.3) imply that, there may be conversion of aldrin to dieldrin by an epoxidation in biological systems (Rumsey and Bond, 1974) and, therefore dieldrin is expected to be found in relatively higher levels than aldrin. The low level of aldrin than dieldrin was as a result of its susceptibility to volatilization, photodegradation, and heat to form metabolites of dieldrin (Fan and Alexeeff, 1999). Moreover, it could also result from biodegradation of aldrin aided by fungi and bacteria and the major transformation product ketoendrin indicated elsewhere (IPCS, 1992). The results obtained from the present study are in agreement with those of the United States Department of Health and Human services (USDHHS, 1993), which showed that aldrin is rapidly converted into dieldrin in plant and animal tissues. This is so because dieldrin is extremely non-polar and therefore has a strong tendency to adsorb tightly to plant waxes. This observation is in agreement with the findings of the research carried out on soil samples from Awash Basin in Ethiopian state farm soil, which indicated the presence of aldrin in soil (Westbom 2008) and its illegal availability and use. The concentrations of aldrin and dieldrin found in all the watermelon samples from the three agricultural locations were much higher than the EU set maximum residue limits (MRLs) 0.01 µg/kg. These high concentrations of aldrin and dieldrin could probably explain their persistence in the environment or continued use in the study area.
5. Conclusions
The concentrations of the studied organochlorine pesticides were observed to be higher in the peel of the watermelon samples, while the root had the lowest concentrations from the three agricultural locations. The concentrations of all the pesticides in the soil samples were observed to be higher at the depth of 21-30 cm, while the lowest concentrations were detected at the depth of 0-10 cm. The total o,p:-DDE, p,p’-DDD , o,p’-DDD, p,p’-DDT in the peel, pulp than leaf, seed, roots and stem of the watermelon samples from the three agricultural locations were lower than the EU set maximum residue limit (MRL) for total DDT, DDD and DDE in watermelon which is 0.05µg/g, while the concentrations of aldrin and dieldrin found in all the watermelon samples from the three agricultural locations were much higher than the EU set maximum residue limits (MRLs) 0.01 µg/kg. Despite bans and restrictions on the use of some of these pesticides in Nigeria, the observed concentrations of the studied pesticides from the agricultural locations could explain either their persistence in the environment or continued use in the study area. Hence, routine monitoring of pesticide residues in these study locations is necessary for the prevention, control and reduction of environmental pollution, so as to minimize health risks.
References
[1] | Athens, F. (1998) Commercial Watermelon Production. Extension Service, University of Georgia, College of Agriculture. |
[2] | Baig, M. N., Darwent, A. L., Harker, K. N. and O’Donnovan, J.T. (1999). Preharvest Applications of Glyphosate for Yellow Toadflax (Linaria vulgaris) Control. Weed Technology, 13: 777-782. |
[3] | Bhattacharya, A. K, Mandel, S. N and Das S.K. (2006). Biaccumulation of Chromium and Cadmium in Commercial Edible Fishes of Gangetic West Bengel Trends in applied Science Research 1: 511-517. |
[4] | Boyhan, M and Masiunas, J. (1999). Identifying and Managing Cucurbit Pests. University of Illinois at urban Champaign. |
[5] | Doong, R.A., Peng, C.K., Sun., Y.C and Liao, P.L. (2002). Composition and Distribution of Organochlorine Pesticide Residues in Surface Sediments from the Wu-Shi River estuary, Taiwan. Mar Pollut Bull 42:246–253. |
[6] | FAO: Food and Agricultural Organisation (2002). World Agriculture: Towards 2015/2030. |
[7] | Feher, T. (1993). Watermelon: Citrulluslanatus (Thumb) Matsun and Nakai B. O. Pergamum Press, Oxford, UK. |
[8] | Gao, J., Liu, L., Liu, X., Lu, J., Zhou, H., Huang, S., Wang, Z and Spear, P.A. (2005). Occurrence and Distribution of Organochlorine Pesticides – Lindane, p,p_-DDT and Heptachlor Epoxide – in Surface Water of China. Environ Intern 34: 1097–1103. |
[9] | Jabbar, A., Zafar, S. M., Parveen, Z and Mubarik, A. (1993). Pesticide residues in cropland soils and Shallow Groundwater in Punjab Pakistan. Bull. Enviro. Contam. Toxicol, 51(2): 268–273. |
[10] | Lacier, G and Plummer, R. (2003). Vegetables and Melons Outlook Report from the Economic Research Services USDA, VGS-296, April 13. |
[11] | Musmade, A.M and Desai, U.T. (2001). Cucumber and Melon In Handbook of Vegetables’ Science and Technology: Production, Composition, Storage and Processing (ed. D.K salunkhe, S.S Kadam), Marcel Dekker, Inc, pp.245-247. |
[12] | Nur-Banu, O.E and Semra, G.T. (2004). Determination of Organochlorine Pesticides in Groundwater of Mediterranean Region of Turkey. Adnan Menderes University, Proceedings Book 249 Turky. pp.53-59. |
[13] | Parsons, J. (2002). Gardening column: Watermelons. Texas Cooperative Extension of the Texas A & M University System. |
[14] | Sanpera, C., Ruiz, X., Llorente, G.A., Jover, L and Jabeen, R. (2002). Persistent OrganochlorineComponds in Sediment and Biota from the Haleiji Lake: A wildlife Sanctuary in South Pakistan, Bull. Environ. Contam. Toxicol. 68: 237-244. |
[15] | Santamaria, I., Velasco, G., Pendas, A. M., Paz, A and Lopez-Otin, C. (1999). Molecular Cloning and Structural and Functional Characterization of Human Cathepsin F, A New Cysteine Proteinase of the Papain Family with a Long Propeptide Domain. J. Biol. Chem.274: 13800-13809. |
[16] | Singh, K.P., Malik, A., Mohan, D and Takroo, R. (2005). Distribution of Persistent Organochlorine Pesticide Residues in Gomti River, India, Bull. Environ. Contam.Toxicol74: 146-154. |
[17] | Thomas, J.E, Ou, L.T and Al-Agely A. (2008). DDE Remediation and Degradation. Rev. Environ Contam. Toxicol194:55–69. |
[18] | Ware, G.W., Cahil, W.P., Estesen, B.T and Buck, N.A. (1978). Accumulation of DDT in Soils.Bull EnvironlContam. Toxicol. 20: 143-144. |
[19] | Zawiyah, S., Cheman, Y.B., Nazimah, S.A.H., Chin, C.K., Tsukamoto, I., Hamanyza A.H and Norhaizan, I. (2007). Determination of Organochlorine and Pyrethroid Pesticides in Fruit and Vegetables Using SAX/PSA Clean-up Column. Food Chem,102: 98–103. |